The School of Earth Sciences is situated at the historic heart of the University of Bristol campus, in the neo-Gothic Wills Memorial Building. The School is one of the top 3 Earth Science research organisations in the UK (e.g., REF2014) and is currently ranked 15th in the world (QS World University Rankings – 2014/15). The School is organised functionally into six research groups (Geophysics, Petrology, Palaeobiology, Volcanology, Geochemistry, Environment), each with a distinct, international brand. The groups make up a vibrant research community that works collaboratively on six Research Themes: (1) Earth Surface Processes; (2) Dynamics and Architecture of the Solid Earth; (3) Evolution of Biodiversity and Morphology; (4) Formation, Structure and Dynamics of Planets; (5) Crustal Magmatism, Volcanism and Geological Risk; and (6) Resources and Reservoirs. The breadth of these Themes emphasizes an explicit recognition that cutting-edge research defies traditional boundaries, and often requires an interdisciplinary approach. The School produces over 120 ISI cited publications per year, including, on average, a publication in Science or Nature every month.
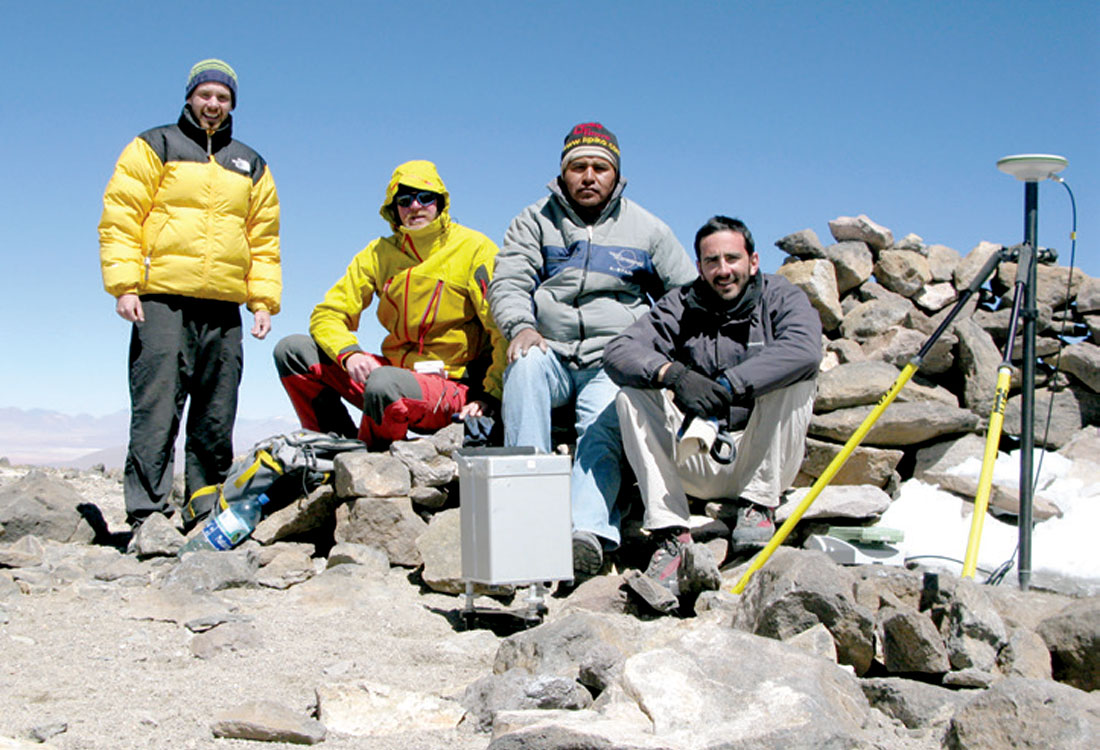
The School currently offers ten undergraduate degrees, which cover three disciplines, Geophysics, Geology, and Environmental Geosciences, including year-abroad study options in the third year. The school offers joint programs with the School of Biological Sciences.
One year Masters (MSc) degrees are offered in Palaeobiology and in Volcanology, as is a one year Masters by Research (MRes) with any research group.
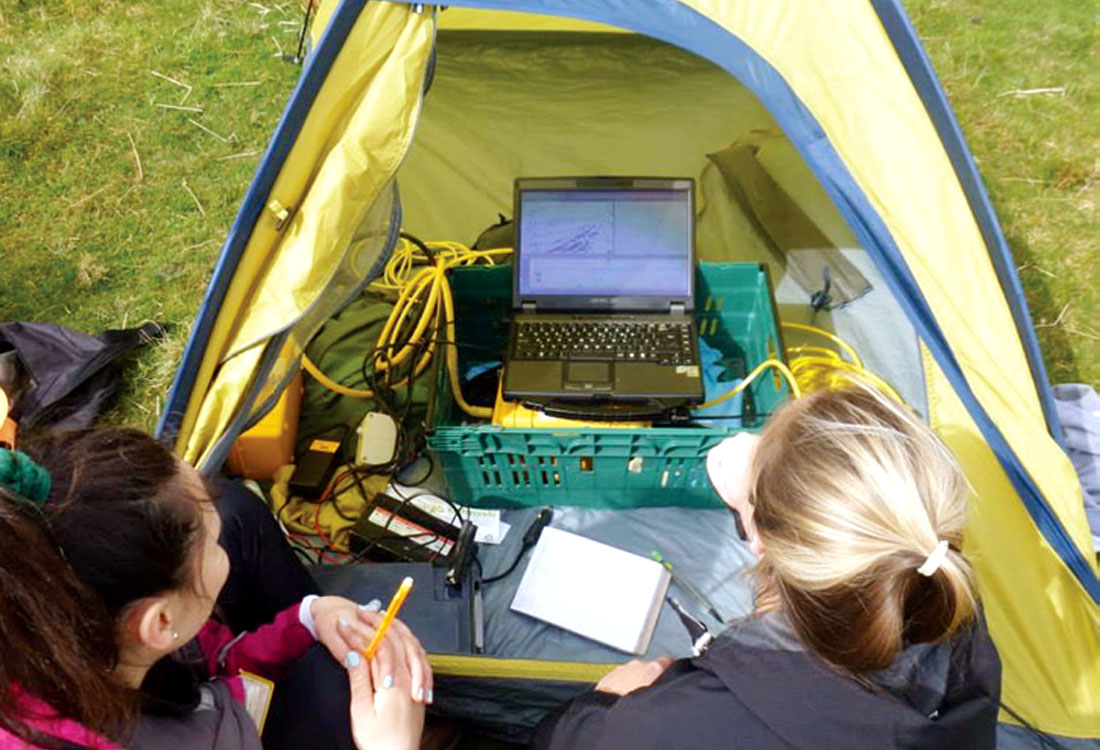
Geophysics at Bristol
The Geophysics Group at Bristol is currently comprised of 12 permanent Faculty, 11 postdoctoral researchers and 12 PhD students, making it one of the largest geophysics groups in the UK. It owns and operates a wide breadth of geophysics equipment, which enhances research and teaching. Researchers in this group work on all but one of the School’s Research Themes, and benefit from a diverse background of funding from industry, government funding bodies and the EU. Members of the group are involved in planetary geophysics, earthquake studies, seismic imaging, volcanology, multi-phase flow and fluid mechanics, and geodesy and tectonics. The School has recently entered into a strategic partnership in Geophysics with the British Geological Survey and they collaborate on a number of joint projects including shale gas stimulation monitoring, geothermal energy and volcanic hazards.
The group is involved in a number of applied research projects as part of the Resources and Reservoirs research theme. A good example of the multi-disciplinary approach to applied research at Bristol is the Bristol Porphyry Deposits project (http://bristolpcd.org), a nearly £4million collaborative project between BHP-Billiton and Bristol. The project involves researchers from 4 of the School’s 6 research groups and aims to modify existing porphyry copper deposit (PCD) models in the light of recent advances in geological research.
For the remainder of this article we summarize three joint industry projects that focus on the application of cutting edge technologies to better understand petroleum reservoirs, both conventional and unconventional.
Bristol University Microseismicity ProjectS (BUMPS)
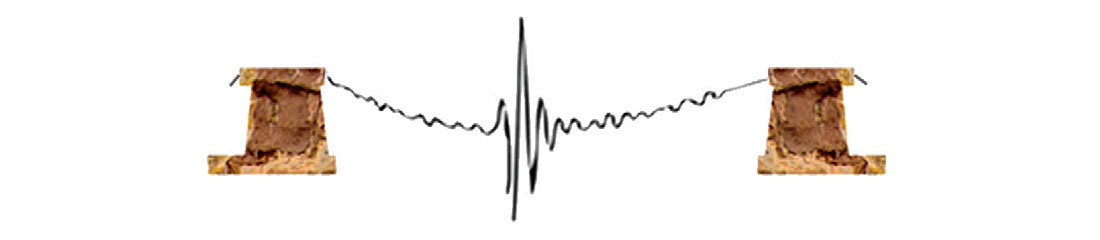
The focus of the BUMPS project is the passive monitoring of microseismic events and their use in characterising reservoirs in a number of geologic settings. These microearthquakes will occur naturally due to regional tectonic stresses, but can be also induced through activities such as hydraulic stimulation, enhanced petroleum recovery, waste injection, fluid extraction, and underground mining. Such monitoring offers insights into the dynamic state of stress – invaluable information for developing effective strategies for drilling, injection, fracturing and production programs. BUMPS is currently in its third phase, sponsored by 10 companies, with significant funding from the Natural Environment Research Council UK (NERC) and the British Geological Survey (BGS).
The focus of the first phase of the project was on borehole monitoring, where array-data were used to refine event locations, identify multiplets, determine focal mechanisms and estimate seismic anisotropy. Technique development continued in the second phase, including the use of surface arrays, and the emphasis shifted to the monitoring of hydraulic fracture stimulation and the storage of CO2. This also led to the formation of the Bristol CO2 Group (BCOG), which has now been involved in 3 of the world’s largest CCS projects (see Verdon et al., PNAS, 2013), and is a research provider for the Aquistore project near Estevan Saskatchewan, the world’s first commercial CCS project. Furthermore, the research developed in BUMPS is now being applied to geothermal energy and volcano monitoring.
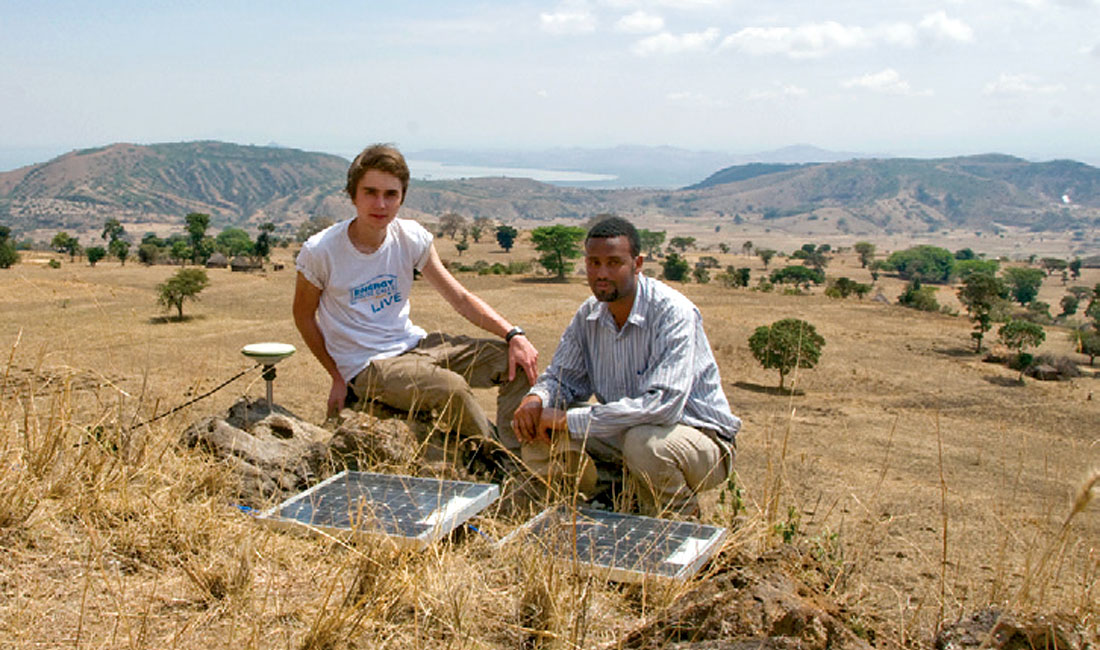
The third and on-going phase is concentrating on key questions and issues facing industry and regulators. These include: When is overburden seismicity a problem? Can we track where fluids and proppant go? Within the context of ‘traffic light’ monitoring schemes, what are the errors in estimates of earthquake magnitudes and focal mechanisms? What role do pre-existing fracture networks play in stimulations? Bristol has now monitored a number of sites for regulatory purposes and is now combining the use of InSAR and seismicity to better understand induced seismicity.
A novel area of research in the BUMPS project has been the development of tools to estimate seismic and attenuation anisotropy using frequency dependent observations of shear-wave splitting (see Kendall et al. (CSEG RECORDER, 2014) and references therein). A key development has been a methodology to invert shear wave splitting measurements for fracture properties including size, density, orientation and compliance. Observations of temporal variations in shear wave splitting have been used to infer fracture network development and the associated increases in fracture compliance due to enhanced permeability.
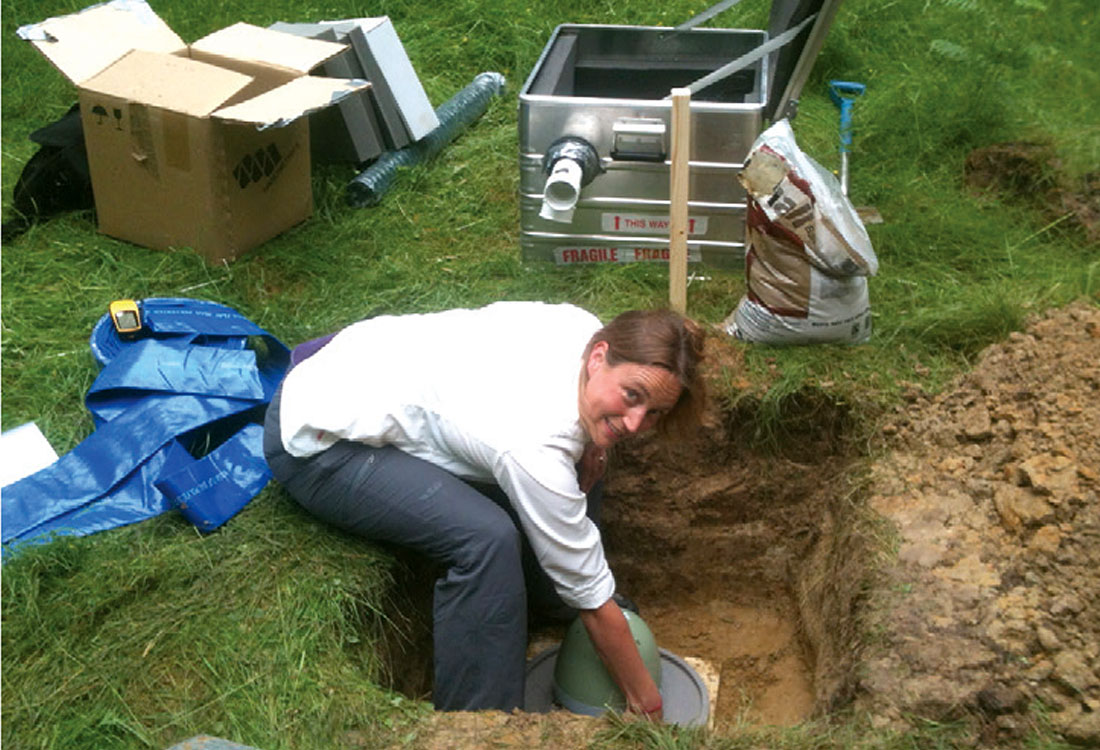
BUMPS is led by Professor Michael Kendall. Other Bristol staff involved include Drs. James Verdon, James Wookey, Max Werner, Anna Stork, Alan Baird and Anna Horleston. For more information see www1.gly.bris.ac.uk/BUMPS and www1.gly.bris.ac.uk/BCOG.
Integrating Petroleum Engineering, Geomechanics and Geophysics (IPEGG)
Geomechanics has, in recent years, emerged as an important area for the oil and gas industry because of the need to extract petroleum from challenging environments such as deep water, high temperature-high pressure, or structurally complex fields. Many reservoirs experience significant compaction during petroleum extraction, which can lead to problems such as sea floor subsidence and seismicity, which can dramatically alter reservoir permeability and redistribute reservoir stresses. These geomechanical-related problems are not easily addressed by conventional software tools used by petroleum engineers. The IPEGG initiative has brought together expertise in reservoir deformation and fluid flow at the University of Leeds, the University of Bristol, and Rockfield Software Ltd – a Swansea-based company that specialises in advanced numerical analysis for geomechanical applications.
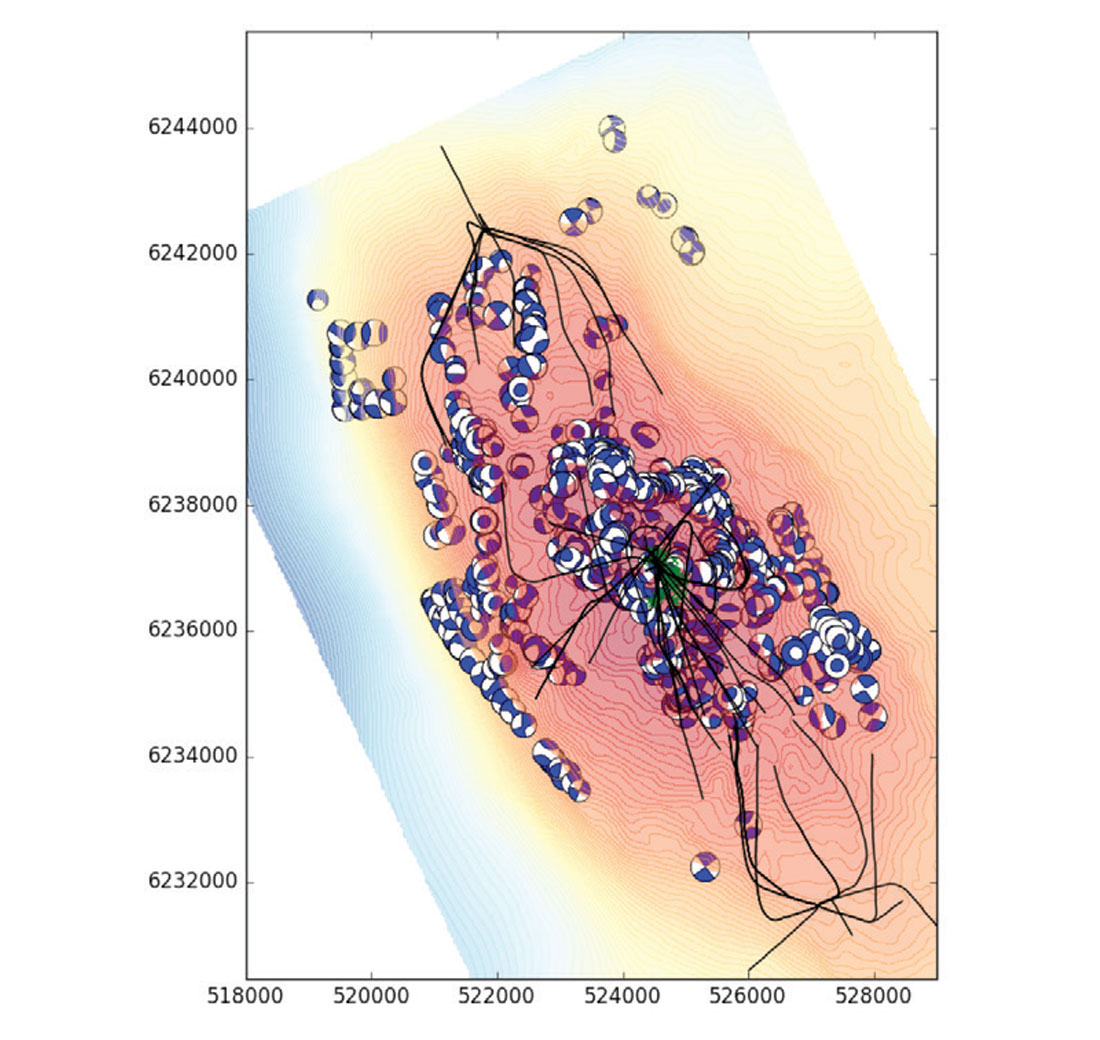
The aim of this theme is to develop and apply coupled fluid-flow and geomechanical simulations to be then integrated with seismic modelling to help predict reservoir behaviour. The work has been facilitated through three JIPs, each coordinated through the Industry Technology Facilitator (ITF) in the UK and sponsored by 15 companies and the Department of Energy and Climate Change (DECC) in the UK. The original project, IPEGG, coupled Rockfield’s finite element (FE) geomechanics software (ELFEN) with a number of reservoir simulators. The output was used in turn to predict deformation and changes in seismic attributes such as velocities, amplitude variations with offset and azimuth (AVOA), and microseismicity. The resulting software was ultimately applied to a full-field simulation of the Valhall field, a large deforming chalk reservoir in the Norwegian sector of the North Sea. The model successfully predicted the observed sea-bottom subsidence, microseismicity and patterns of stress induced AVOA.
Following on from this the GESER project developed components of an integrated geomechanical-seismic toolbox for guiding production in tight gas sand reservoirs. The toolbox contained modules that can: (i) help locate and characterise sweet spots in in tight gas formations via natural and induced fractures; (ii) predict reservoir stress distributions before and during production; and (iii) provide a better estimate of stress conditions from borehole image logs. Through this project, observations of temporal variations in fracture-induced anisotropy in a tight-gas sandstone were interpreted in terms of an interrelation between pre-existing fractures and the development of new fractures during hydraulic stimulation.
The main goal of the current JIP, FracGas, is to develop a FE-based modelling technology and microseismic methodology that will improve industry understanding of hydraulic fracturing processes. Improved planning of hydraulic fracture stimulation could significantly increase the profitability and ultimate recovery of gas from tight sandstone and shale formations.
This initiative was originally led by Professor Quentin Fisher at the University of Leeds and involves Dr. Doug Angus (Leeds), Dr. Martin Dutko (Rockfield) and Professor Michael Kendall, Dr. James Verdon and Dr. Alan Baird at Bristol. For more information see: homepages.see.leeds.ac.uk/~eardang/Industry_funded_JIPs/JIPs.html
Carbonate Diagenesis: Process, Product and Prediction
Work in this area focuses on fundamental controls on flow and reactions in carbonates, linking processes and products. The distribution of reservoir quality and potential fluid flow behaviors within carbonates lithologies are often complex and scale-dependent, arising from the interplay between depositional facies and multiple stages of diagenesis that modify the reservoir from birth to final burial. Deconvolving these multiple stages is challenging. While outcrop and petrographic studies may reveal the diagenetic processes that affect a reservoir, there is rarely sufficient data to provide clear guidance on the 3D distribution of their products. This is addressed using process-based numerical models to integrate knowledge of geophysics, hydrology, geochemistry, structural and sedimentary geology and petrophysics.
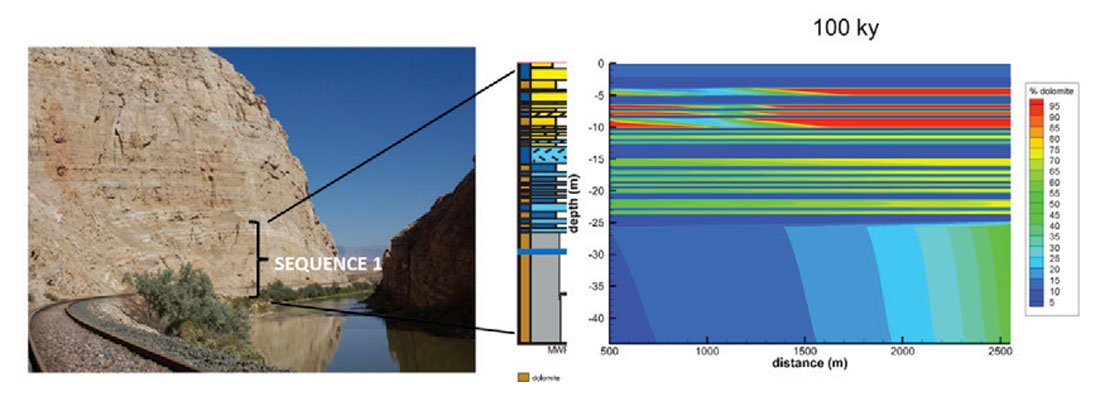
Over the past 15 years Bristol has developed CARB3D+, an innovative forward modelling package for the prediction of sedimentary architecture and diagenesis of carbonate platforms. This work has been funded by the Leverhulme Trust and 8 companies. CARB3D+ allows interactions between depositional facies distributions and syndepositional dissolution and cementation to be explored within a strict sequence stratigraphic framework and provides understanding of the complex distributions of reservoir quality that commonly result. Applications range from exploring fundamental controls on reservoir quality in generic platforms, to simulation of outcrop analogues (such as the Triassic Latemar and Pegherolo Massifs) and specific reservoirs (for example to predict multiple overprints by meteoric diagenesis at the Tengiz Field in Kazakhstan).
More recently the group has developed world-leading expertise in reactive transport modelling of carbonate diagenesis. Bristol is now in the second phase of IRT-MODE, funded by 5 companies via the Industry Technology Facilitator (ITF). This project integrates models of reactive fluid flow with existing observational data to generate a new workflow, applied to predict the temporal and spatial distribution of reflux dolomitisation. Pore-scale textural influences on reactive surface area and permeability are integrated into inter-well to regional- scale models of reactive flow. In collaboration with Manchester, Bergen and Royal Holloway Universities this has recently been extended via the PD3 Project to evaluate the impact of later overprinting by hydrothermal diagenesis, with specific application to hydrothermal dolomites in rifted Tertiary carbonates of the Red Sea. Modelling is already suggesting novel solutions to Mg2+ mass balance requirements, both in terms of plumbing and fluid chemistry.
Research in carbonate processes at Bristol is led by Dr. Fiona Whitaker, but also involves Professor Maurice Tucker, and Drs. Tom Scott, Tatyana Gabellone, and Brioch Hemmings. For more information see:
http://www.bridge.bris.ac.uk/projects/carb3dplus and https://sites.google.com/site/bristolitfproject/home
Join the Conversation
Interested in starting, or contributing to a conversation about an article or issue of the RECORDER? Join our CSEG LinkedIn Group.
Share This Article