The Edinburgh Anisotropy Project is a long running industry-academic research consortium working on all aspects of seismic anisotropy, with a particular emphasis on anisotropic rock physics, fracture detection and converted-wave seismic methods. In recent years EAP has increasingly addressed problems arising in seismic shale characterization. The ultimate goal of this work is to develop anisotropy based methods into a robust and efficient tool to determine the physical properties of shale from seismic data. We believe that multicomponent seismic has an important role to play in shale characterization, and our approach is based on the careful development and calibration of suitable anisotropic rock physics models.
Shales exhibit very strong intrinsic anisotropy, much of which is attributable to alignments in the clay and organic phases. Since the pioneering work of Hornby and co-workers in the 1990’s, efforts have been made to capture this effect through application of equivalent medium theory, particularly the self-consistent and differential effective medium approximations. Markedly different approximations are needed depending on the amount of organic matter present.
For kerogen-rich shales, we typically use models which have kerogen as a background material, with other minerals inserted as inclusions. An example of this is shown in Figure 1, where the elastic stiffnesses of the Bazhenov shale are modelled by the anisotropic Differential Effective Medium (DEM) theory. We can see organic shales with thin inclusions exhibit higher anisotropy. With an increase of aspect ratio, C11 and C44 become closer to C33 and C66, indicating that the anisotropy become weaker. Black circles indicate the estimated elastic stiffnesses for the Bazhenov shale sample by Vernik and Liu (1997) when the kerogen volume percentage is 16.8%.
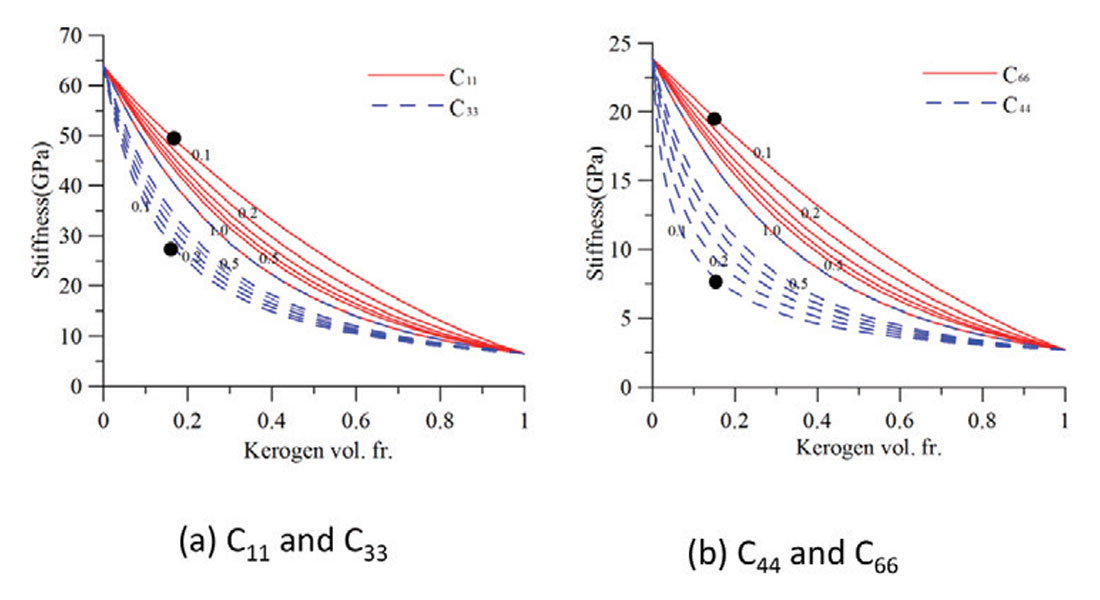
Maturity can also have a strong impact on the seismic properties of shales. As the source rock matures, porosity is generated within the organic matter and this can directly impact the seismic properties. We have applied equivalent medium theory to model this process, as illustrated in Figure 2. Key factors in the model are the kerogen content and the degree of hydrocarbon related porosity.
The final goal of the work is to apply the rock physics to the interpretation of seismic data. Subtleties in the shale properties can have important implications for seismic amplitude interpretation. When vertical fractures are present in the shale, we typically expect orthorhombic symmetry to be present due to the combination of the intrinsic transverse isotropy with a vertical symmetry axis and the horizontal transvers isotropy arising from the fractures. In these orthorhombic scenarios the azimuthal anisotropy contains very important information on fracturing and composition, but we argue that correct interpretation relies on careful modelling of the expected responses.
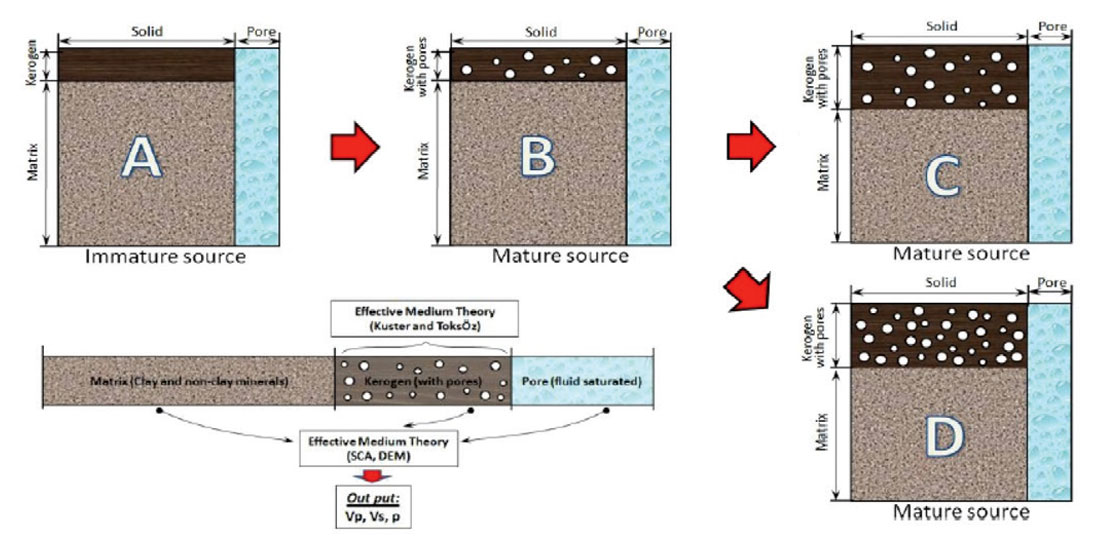
We have illustrated this point through extensive modelling studies. We use the VTI model considered above for the organic shale, and insert a vertical fracture set using Schoenberg’s linear slip theory. This allows us to calculate the reflection coefficients as a function of angle of incidence and azimuth for various values of fracture compliances, but also for varying kerogen content.
Azimuthal variations in PP reflection coefficients are often interpreted in terms of the Ruger equation, which allows an “anisotropic gradient”, denoted Bani , to be calculated. It is commonly assumed that this anisotropic gradient should be an indicator of the fracture properties. In our case we calculated the anisotropic gradient as a function of kerogen content, while keeping the properties of the fracture system constant. As illustrated in Figure 3, the anisotropic gradient can vary quite strongly with kerogen content for constant fracture properties. We believe that this implies that attempts to interpret azimuthal anisotropy without a detailed knowledge of the underlying rock physics relationships can be potentially dangerous. The work also emphasises the potential importance of shear-wave data. If shear-wave splitting is available to give an estimate of the fracture density, then these measurements can in principle be used in conjunction with the P-wave data to resolve both fracturing and composition.
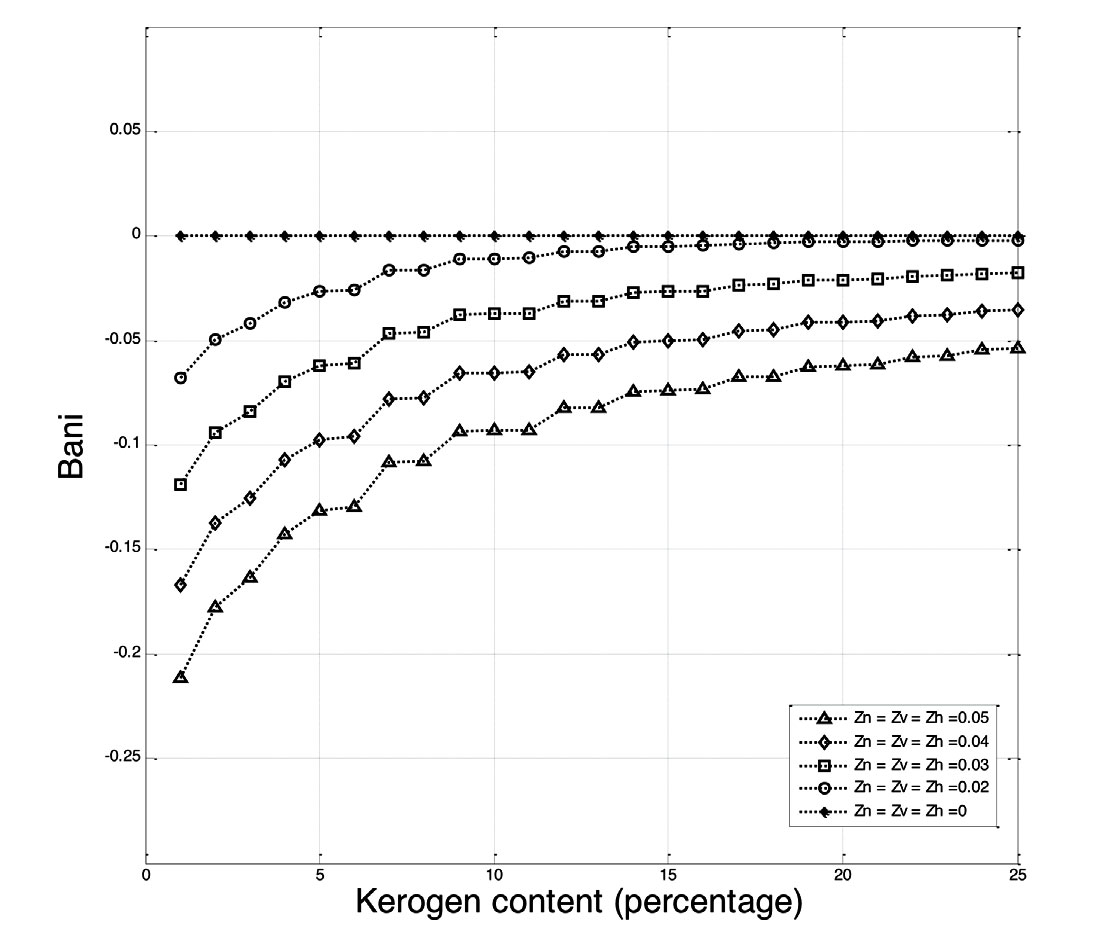
Such complications illustrate the need to develop robust and practical methodologies which can be applied to real data. To create suitable models we typically rely on well-log data. Our approach begins with the construction of models which allow the elastic properties to be predicted from the composition logs. Typically, this involves defining which phases are to be considered as “background” and “inclusion”, and inverting a collection of aspect ratios from the data using combinations of the self-consistent and differential effective medium theories. To maximise the predictive power of the models we are keen to keep the number of parameters to a minimum, and this often translates to assuming that each aspect ratio remains unchanged throughout the modelling exercise. Often this is not possible, as changes in the geology are reflected in microstructural properties, requiring a different model for different intervals. The appropriate background phase can also vary spatially.
This variability in properties is illustrated in Figure 4. Following the probabilistic method of Jiang and Spikes (SEG Annual Meeting, 2012) we have estimated the pore aspect ratio as a function of depth for the Barnett Formation from the sonic and porosity logs. The vertical axis is depth and the horizontal axis is aspect ratio. Markedly different results are apparent in the different formations, and this is consistent with the varying microstructures. In such cases, we need to specify different rock physics models for the various intervals.
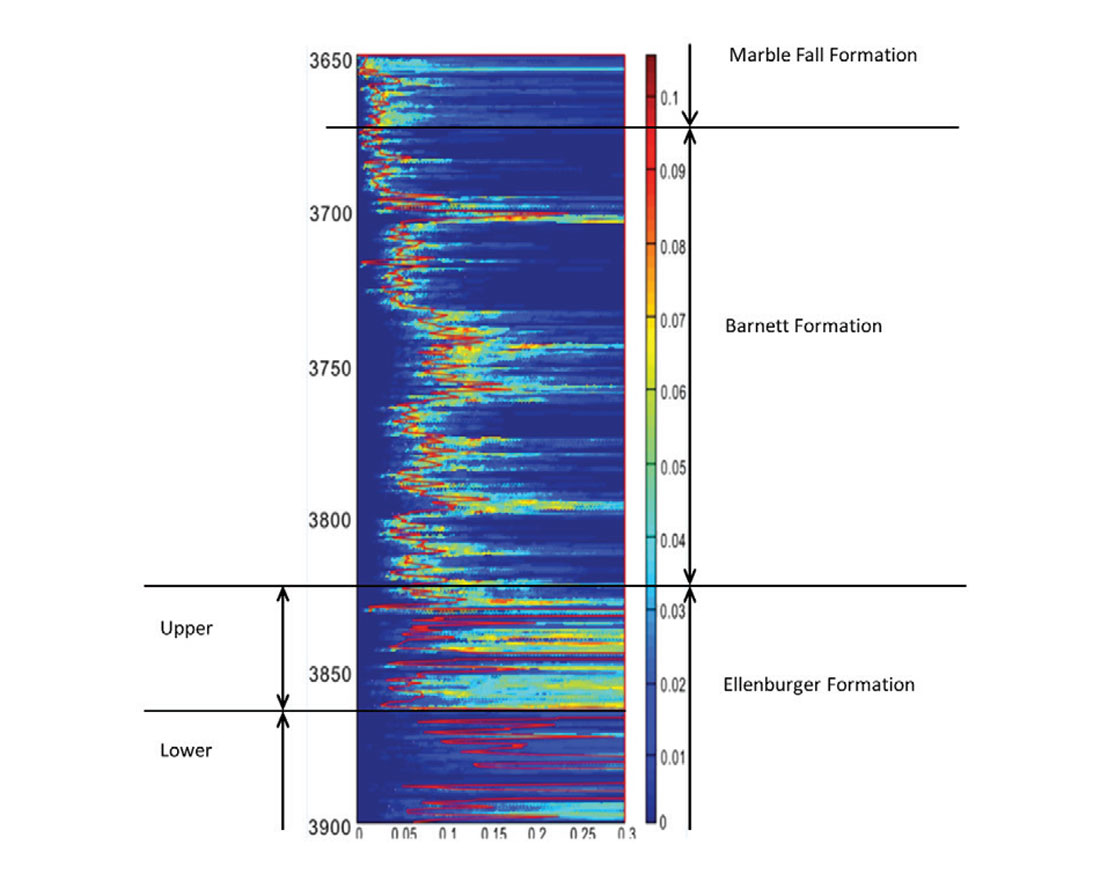
We are currently working on a number of integrated projects, in which we combine well log, VSP and seismic reflection data. One such project involves data from the Marcellus shale in the USA. The scope of the research begins with modelling the well logs, but progresses to consider anisotropy measurements from VSP. This requires us to update our models to account for the observed anisotropy, but will also allow us to calibrate the azimuthal anisotropy to FMI data. We aim to demonstrate the usefulness of these fully calibrated anisotropic models through analysis of the reflection seismic data, including both PP and PS, producing maps of composition and fracture properties.
Another area of interest is a microseismic project in the Barnett Shale. The project is measuring shear-wave splitting from microseismic events during hydraulic fracturing. We hope to observe temporal variations in the anisotropy, which may be related both to the fracturing and to pressure changes. Observation of frequency-dependence of the shear-wave splitting has the potential to estimate scale lengths associated with the fractures, and we are keen to assess the viability of this idea.
We see significant scope for the application of seismic anisotropy to shale problems and hope that our approach, based on rock physics theory but heavily oriented towards practical data analysis, can contribute to the development of this important area of current research.
Acknowledgements
Article contributed by Mark Chapman. Mark is Senior Lecturer in Rock Physics at the University of Edinburgh. He joined the Edinburgh Anisotropy Project in 2000, and since that time has been working on the application of seismic anisotropy to a range of industrial problems. His current work in rock physics ranges from fundamental theoretical development to practical applications, with a significant current emphasis on problems related to shale geophysics.
Join the Conversation
Interested in starting, or contributing to a conversation about an article or issue of the RECORDER? Join our CSEG LinkedIn Group.
Share This Article