Curtin University is centrally located in Perth at the heart of Australia’s premier energy and mining state of Western Australia. The Department of Exploration Geophysics, part of the Curtin WA School of Mines (WASM) at Curtin University, provides a comprehensive range of internationally recognised specialist geophysics degree level courses ranging from a BSc and MSc to MPhil and PhD. The department offers training in the latest techniques in exploration geophysics. The academic staff and postgraduate students are continuously involved in frontier scientific research in this field. In addition to Australian students, the postgraduate geophysics research degrees attract highly capable students from a multitude of cultural backgrounds from around the world.
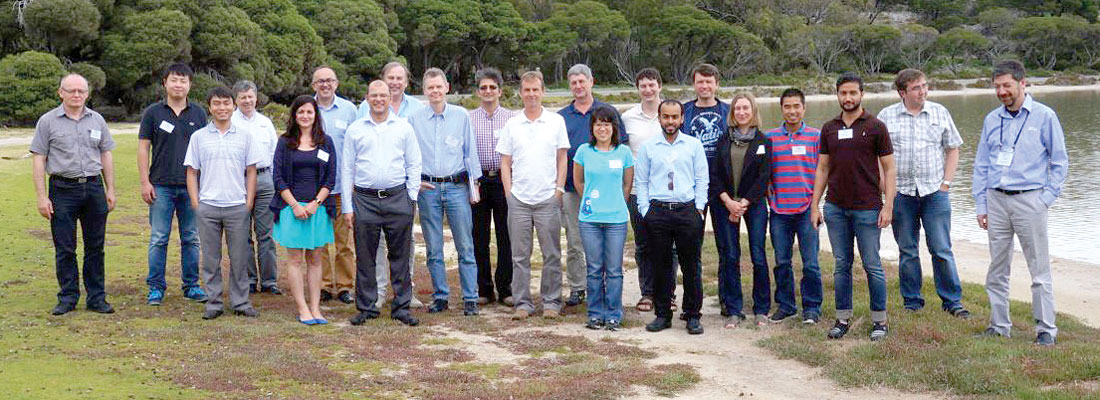
The department places a strong focus on associations with energy industry organisations and plays a vibrant role as the key academic partner within a research consortium of Australian and global oil industry majors and leading geophysical contractors. An annual conference of CRGC (Curtin Reservoir Geophysics Consortium) is held on Rottnest Island for members to review advances made in geophysics research by the group. The broad aims of the consortium are: to conduct geophysical research and develop new technologies for the benefit of the petroleum industry; and, to train students in geophysical methods and research relevant to the needs of members. Current CRGC research covers four major project areas:
- Theoretical and experimental rock physics;
- Seismic processing and imaging;
- Reservoir characterisation and monitoring;
- Sea-bed electromagnetics.
For the last ten years, the department has been a participant in the Cooperative Research Centre for Greenhouse Gas Technologies (CO2CRC). As part of this participation, the department was heavily involved in the Otway Basin CO2 Geosequestration Project. This project started out as the first land time-lapse seismic survey carried out in Australia and continues to push scientific boundaries with the recently deployed buried permanent monitoring array using both traditional as well as distributed acoustic sensors. Since 2010, the department has also been involved in the Deep Exploration Technologies Cooperative Research Centre (DETCRC). Although focused on deep mining exploration, the geophysical technologies being developed also address some petroleum problems, such as land seismic acquisition, seismic while drilling, and data processing for environments with complex near-surface conditions.
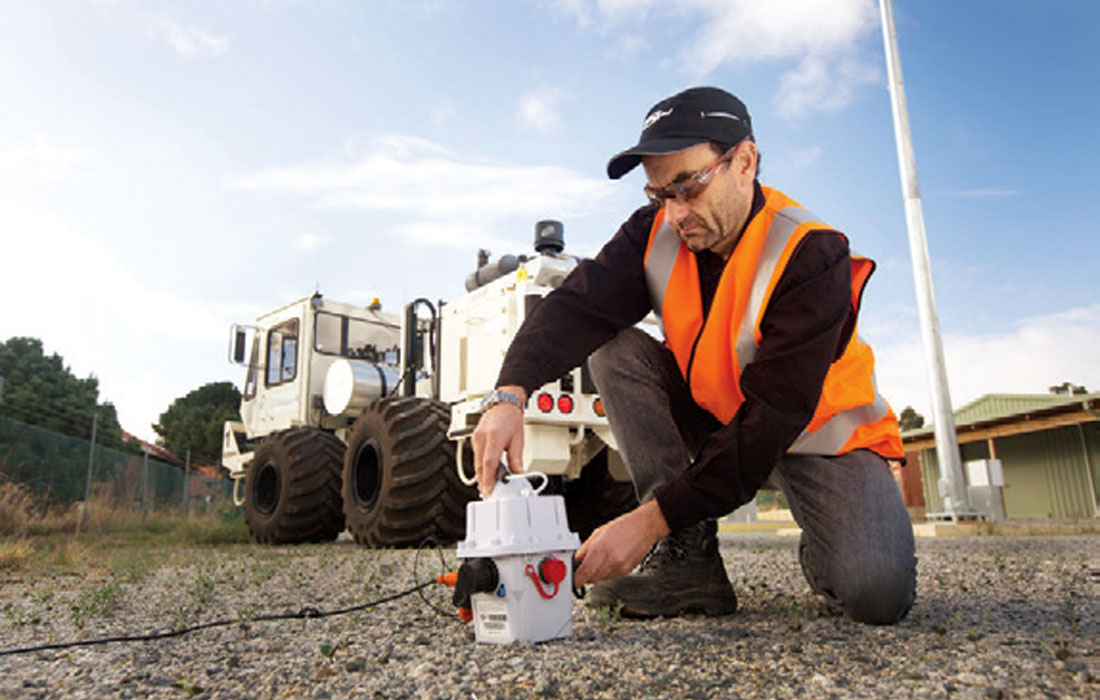
Professor Boris Gurevich is Head and Lead Researcher of the Department of Exploration Geophysics and is the Director of the Curtin Reservoir Geophysics Consortium (CRGC). Other senior academics within the research groups are Milovan Urosevic, Anton Kepic, Brett Harris, Maxim Lebedev, Andrej Bona and Roman Pevzner. The research team includes a dozen junior academics and four technical support staff. Geophysicist Rob Ross provides expertise as CRGC looks to expand its involvement with partners.
Theoretical and Experimental Rock Physics
Seismic signatures of patchy saturation
A major effort of the department is focused on the study of the effects of patchy saturation on seismic signatures. The main objective is to quantify the effect of random spatial distribution of fluid patches. The approach is based on the general theory of heterogeneous poro-elasticity developed by CRGC over the last decade. The aim of the current effort is to build a general model for elastic properties of partially saturated rock with a given statistical distribution of fractures and with arbitrary contrast between the properties of the two fluids (e.g., gas and liquid). The department is also performing a series of fluid injection experiments with X-ray Computer Tomography and ultrasonic control to validate the theoretical findings. The effect of capillary forces on the elastic properties of partially saturated rocks is also being explored. On field scale, the results of theoretical research have been applied to time-lapse logs acquired at the Nagaoka CO2 storage site.
Modelling of stress dependent attenuation and dispersion due to squirt flow in clastic and carbonate rocks
The department has developed a new squirt flow model in which all parameters can be independently measured or estimated from measurements. The pore space of the rock is assumed to consist of stiff porosity and compliant (or soft) pores present at grain contacts. The effect of isotropically distributed soft pores is modelled by considering pressure relaxation in a disk-shaped gap between adjacent grains. This derivation gives the complex and frequency-dependent effective bulk and shear moduli of a rock, in which the soft pores are liquid-saturated and stiff pores are dry. The resulting squirt model is consistent with Gassmann’s and Mavko-Jizba equations at low and high frequencies, respectively. As expected, the dispersion and attenuation are the strongest at low effective stress and much smaller at higher effective stress. The department will be testing and refining this model using ultrasonic and low-frequency measurements on clastic and carbonate rocks saturated with different fluids. The model is also being extended to partial saturation, anisotropy and viscoelastic fluids.
Modelling of properties of rocks saturated with heavy oil
Rock physics for heavy oil is different from rock physics for conventional fluids because its viscoelastic rheology makes Gassmann theory and all its extensions inapplicable in principle. The department has developed an approximate methodology for fluid substitution in heavy-oil reservoirs. The methodology is based on one particular equivalent-medium approach known as coherent potential approximation (CPA). The methodology has been successfully tested on low frequency laboratory measurements. Currently, the department is developing an alternative model for heavy oil rocks based on the concept of quasi-static squirt flow.
Low frequency acoustic measurements
Theoretical rock physics models need to be tested and calibrated using laboratory measurements. To this end, the department is performing comprehensive experimental testing of these theories using broadband measurements of dynamic elastic properties of rock samples. Experiments are conducted using a combination of forced-oscillation ultra-sensitive strain gauge measurements (10 Hz – 2KHz), laser interferometry (100 Hz – 200 kHz) and ultrasonic testing (100-1000 kHz). The results are being compared with theoretical predictions computed using numerical simulations.
Modelling elastic properties of fractured reservoirs
A major effort of the department’s rock physics group is directed towards modelling attenuation, dispersion and frequency dependent anisotropy of porous reservoirs permeated by aligned fractures. Over the last decade, this group has developed a methodology of fluid substitution in fractured reservoirs, which is based on the combination of anisotropic Gassmann equations and Schoenberg’s linear slip parameterisation of the effect of fractures on rock properties. Between 2003 and 2006, the group developed a model for attenuation and dispersion of P-waves propagating perpendicular to a periodic system of parallel planar fractures and validated this model with numerical simulations using a poroelastic extension of reflectivity method. These simulations helped to extend the attenuation/dispersion model to randomly spaced fractures and to oblique incidence.
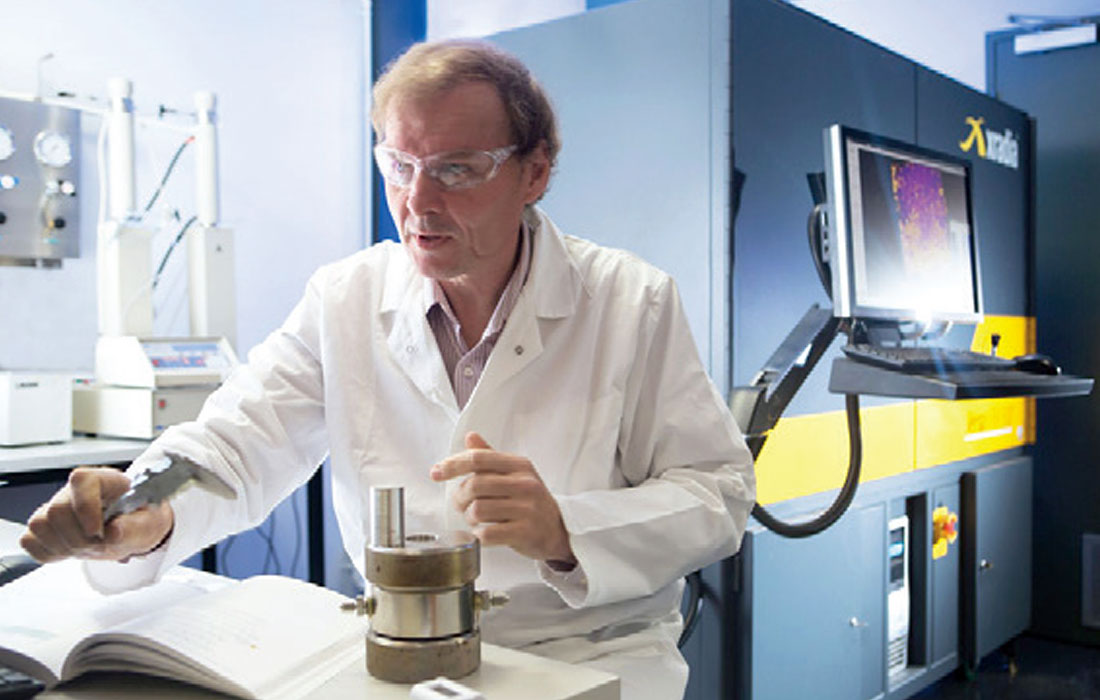
More recently, the group developed a model for seismic attenuation and dispersion caused by the presence of sparsely distributed finite fractures in the porous reservoirs. The model is based on the combination of Biot’s theory of poroelasticity with the ideas of a multiple scattering theory. The current effort in this area is focused on the deeper understanding of the implications of this theory and its extensions to:
- Oblique incidence;
- Shear waves;
- Higher fracture densities;
- Arbitrary aspect ratios.
While all of the above models are designed for a single set of aligned fractures, real reservoirs often contain multiple fracture sets. Moreover, similar phenomena (fluid flow between pores and fractures) lead to frequency dependent attenuation and dispersion in isotropic rocks with microcracks, compliant grain contacts, etc. These effects are being studied by extending the aligned fracture models to arbitrary angular distributions of fractures.
Modelling stress-induced anisotropy
Stress is one of the major causes of anisotropy in the earth. Understanding it is important for imaging, reservoir characterisation and monitoring. There is a need to be able to distinguish stress-induced from fracture-induced anisotropy. In recent years, the department has developed theoretical models of stress-induced anisotropy of rocks. The department first considered an isotropic linearly elastic medium (porous or nonporous) permeated by a distribution of discontinuities with random (isotropic) orientation (such as randomly oriented compliant grain contacts or cracks). When this isotropic rock is subjected to a small compressive stress (isotropic or anisotropic), the number of cracks along a particular plane is reduced in proportion to the normal stress traction acting on that plane. This effect is modelled using the Sayers-Kachanov noninteractive approximation. The model predicts that such an anisotropic crack closure yields elliptical anisotropy, regardless of the value of the ratio of the normal to shear compliance. It also predicts the ratio of Thomsen’s anisotropy parameters as a function of the compliance ratio and Poisson’s ratio of the unstressed rock. The model is tested using laboratory data. In addition, it has been extended to large stresses, and also to a general triaxial stress state (leading to orthotropic symmetry). The results can potentially be used for differentiating between stress and fracture induced anisotropy, and also for predicting P-wave anisotropy from S-wave anisotropy; the latter may be estimated from shear-wave splitting as measured by modern sonic logs or VSP.
Seismic Processing and Imaging
Passive seismic and Seismic Interferometry
Seismic imaging and subsurface characterisation without the use of active sources has gained importance due to the need for both monitoring microseismic events and simplified acquisition of data. The department’s focus in the area of passive seismic and interferometry includes: using drilling activities as a seismic source; passive imaging of diffractors and their characterisation and separation from microseismic events; and, generating shear-wave sources at the locations of 3C geophones in VSP type experiments for imaging and anisotropy estimation.
3D / 3C VSP
Vertical seismic profiling enables analyses and understanding of the geological origin of the wave field generated. The biggest advantage over surface seismic methods is that both transmitted and reflected fields are available for an analysis; in situ rock-sample measurements are conducted by recording VSP data. VSP data is used for most of surface seismic related investigations to utilise the benefits of having full wave field available for the analysis. Frequently, significantly higher resolution VSP data are encountered in comparison to surface seismic data. Hence, 2D and 3D images obtained with VSP data can be important for reservoir development, particularly for deep hydrocarbon accumulations. The main areas of the department’s research interest are: 2D/3D imaging using monotypic and converted waves; estimation of anisotropy and attenuation from VSP data; and, developing alternative approaches to compensation for attenuation.
Imaging methods that do not require separate velocity analysis
Seismic migration methods rely on a background velocity model of the subsurface. Methods that can provide velocity models automatically along with the migrated seismic data can form an important part of the imaging and characterisation workflows. The department is developing various pre-stack imaging methods that result in subsurface velocity models. Currently, the focus is on time migration algorithms. The methods generally rely on estimation of extra attributes, such as local slopes or curvatures, or use of stationary phase in a summation over the velocities.
Using diffractions for imaging and migration steering
Seismic diffractors correspond to many important subsurface features that are usually not well imaged using methods developed for reflection seismology. Specialised methods that focus on imaging of diffractors thus enhance the resolution of the subsurface images. One of the research topics of the department is imaging of the diffractors along with their seismic characterisation using their amplitude response. This approach is closely linked to improving reflection imaging in low signal to noise environments since only small parts of the diffraction hyperbolae used for summation in standard migration algorithms contain the energy from the reflection. Therefore, the inclusion of all the traces from such diffraction hyperbola may not contribute constructively to the final image. One way of identifying the traces that do not contribute constructively to the final image is by using the amplitude/energy distribution information used in the above-mentioned diffraction imaging/characterisation. The department uses a modified 3D Kirchhoff post-stack migration algorithm that utilises coherency attributes obtained by diffraction imaging algorithm in 3D to weight or steer the main Kirchhoff summation.
Reservoir Characterisation and Monitoring
Land and downhole time-lapse seismic monitoring
In the framework of CRC for greenhouse gas technologies, CRGC researchers are involved in the first integrated land time-lapse seismic project in Australia. The project involves monitoring of CO2 sequestration using 4D seismic data and time-lapse 3D VSP over Naylor field, Otway Basin, Victoria. The work involves research in the following topics:
- Analysis of land 2D and 3D seismic repeatability with field tests using surface and buried geophones;
- Developing time-lapse processing flows and specialised algorithms for consistent processing of legacy land data shot with different sources and in different weather conditions;
- Analysis and modelling of time-lapse noise;
- Rock physics modelling of changes of elastic properties of reservoir rocks from flow simulations;
- Seismic forward modelling of time-lapse seismic signal from flow simulations;
- Assessment of feasibility of time-lapse monitoring using integration of geological modelling, flow simulations, rock physics, seismic forward modelling, and seismic noise estimation;
- Data acquisition using novel technologies, including buried geophones, permanent sources, and distributed acoustic sensors.
Sea-bed electromagnetics modelling, 3D visualisation and survey design
Sea Bed Electromagnetic methods are a relatively new and valuable technology for hydrocarbon exploration in deep water settings. Modern surveying equipment provides the possibility of multi-offset, multi-frequency and more recently multi-azimuth data sets. The arrangement and orientation of EM sources and receivers relative to the geoelectrical nature of the target and host is a key part in determining the success of a Sea Bed Electromagnetic survey. Further analysis of complex Sea Bed EM data sets, which typically include MT data, requires the assistance of inversion. The CRGC research in Sea Bed EM is focused on: (a) integrated visualisation and survey planning; (b) interactive 2D/3D inversion of Sea Bed Electromagnetic data; (c) design of novel instrumentation; and, (d) understanding the impacts of electrical anisotropy on the Sea Bed EM response.
Laboratory and Field Equipment
To support application of geophysical research to real world problems, the department operates state of the art laboratory and field data acquisition equipment.
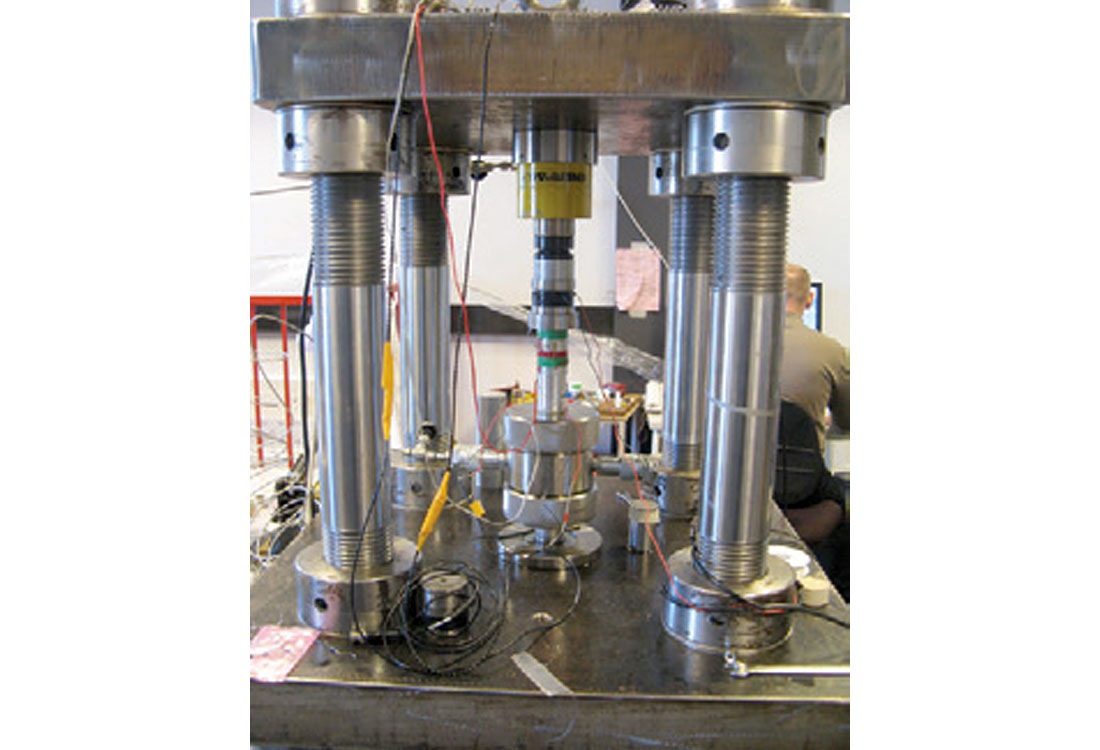
Laboratory equipment includes:
- Triaxial Cells for core sample ultrasonic measurement at confining and axial pressures up to 70 MPa, pore pressure of fluids up to 60 MPa, temperature up to 80°C;
- Unique Triaxial Cell for core sample measurements at seismic frequencies applying dynamic stress level less than 10-7 – confining and axial pressures up to 70 MPa, pore pressure of fluids up to 60 MPa, temperature up to 80°C;
- True-Triaxial Cell for sample measurements using Laser Doppler interferometer, stress level up to 100 MPa (with pore pressure control);
- Plastic core flooding cells transparent for X-ray, for simultaneous measurement of ultrasonic wave velocities and distribution of fluids – pressures up to 50 MPa, pore pressure up to 40 MPa;
- Pressure cell for measurement of fluid bulk modulus – pressure up to 40 MPa, temperature up to 80°C;
- Pressure cells for powered compression and ultrasonic measurements;
- Various acquisition systems for signal registration;
- Access to medical CT;
- Micro-CT XRMVersa500 – Xradia;
- Resistivity measurements systems. Field equipment includes:
- 5000 channel Sercel + 1200 channel Seistronix Seismic Systems;
- Inova Univibes and weight drop sources;
- 10 level 3C VSP tool;
- AGI Supersting and Syscal Pro 72 Resistivity sets;
- SMARTem and GDP 32 electro-magnetic receivers;
- In-house EM transmitters and RVR receivers;
- Mala GPR system;
- Phoenix MT, EM and IP system;
- GEM GSM19 magnetometers.
To contact the department, send an email to info@geophy.curtin.edu.au
Join the Conversation
Interested in starting, or contributing to a conversation about an article or issue of the RECORDER? Join our CSEG LinkedIn Group.
Share This Article