Summary
Information about chemical changes that occur in rocks during burial—diagenesis—can help improve geologic fracture characterization and prediction by accounting for rock mechanical property changes through time and by providing evidence for how fractures grow and become sealed. Diagenesis information can also be a key ingredient in better geophysical interpretation of seismic data. Work on fracture diagenesis suggests that even in relatively simple regional fracture systems where even the largest fractures are likely to be difficult to detect with seismic, heterogeneous cement precipitation can combine with fracture arrays to produce domains of differing fracture porosity preservation. The size of these domains in many cases is probably sufficiently large, as much as tens to hundreds of meters in height and width, that they are plausible candidates for creating a meaningful seismic response. Recent work on fractures that takes diagenesis into account shows that some common assumptions that geophysicists make about fractures, such as the alignment of open fractures parallel to current maximum horizontal stress directions, cannot be sustained and should be replaced with inferences based on site-specific subsurface data on fracture attributes from observed relations between fractures and diagenesis.
Fractures in a Chemically Reactive Environment
Post depositional sediment alteration or diagenesis includes cement precipitation and rock and cement dissolution. Diagenesis is pervasive in the chemically reactive environment of the subsurface, where hot water is ubiquitous. Diagenesis can progressively alter rock properties and pore pressure and therefore affect when and how fractures grow (Laubach et al., 2009). Precipitation and dissolution in fractures can stiffen, widen, or seal fractures. Research on the interaction of deformation processes and chemical alteration (or structural diagenesis) is providing insights into fracture and fault attributes, improving characterization, and enhancing pre-drill predictions. It is beyond the scope of this note to review all of these developments; some have been discussed recently in a theme issue of the Journal of Structural Geology (Laubach et al., in press) and in AAPG Bulletin (Olson et al., 2009). Here we briefly discuss some developments in fracture diagenesis that are germane to seismic interpretation and development of seismic methods.
Fractures in subsurface rocks remain challenging to predict or characterize owing to the unavoidable difficulty in obtaining meaningful subsurface fracture samples: the dimensions and configurations of most wellbores and the arrangement of many fractures assures that few or no fractures may be sampled in potential zones of interest. Although many hydrocarbon reservoirs having low porosity likely are productive because natural fractures enhance hydrocarbon delivery to wellbores, exploration and development decisions must often be made in the face of uncertainty about how fractures contribute to production and how these contributions (or lack of contribution) vary on bed-by-bed, field and play scale. Understanding of the effects of natural fractures on producibility remains incomplete.
Geologic investigation relies on sampling natural fractures using cores (Nelson, 1985; Hooker et al., 2009) or logs (Barton and Moos, 2009). Such studies are commonly also guided by analysis of outcrop analogs (e.g., Hennings et al., 2000; Laubach and Ward, 2006) (Figure 1). The conceptual underpinning of these studies is predominantly mechanics and the focus of many such studies is on open natural fractures, since these may locally enhance permeability or even augment storage capacity. Yet core studies reveal both open and sealed fractures in fractured shale, sandstone, and carbonate rocks (Figure 2). The causes and consequences of cement deposits that seal or partly seal fractures has received less attention despite evidence that the presence of cemented or open fractures can mean the difference between zones that do not contribute to production and those that do (Laubach, 2003).
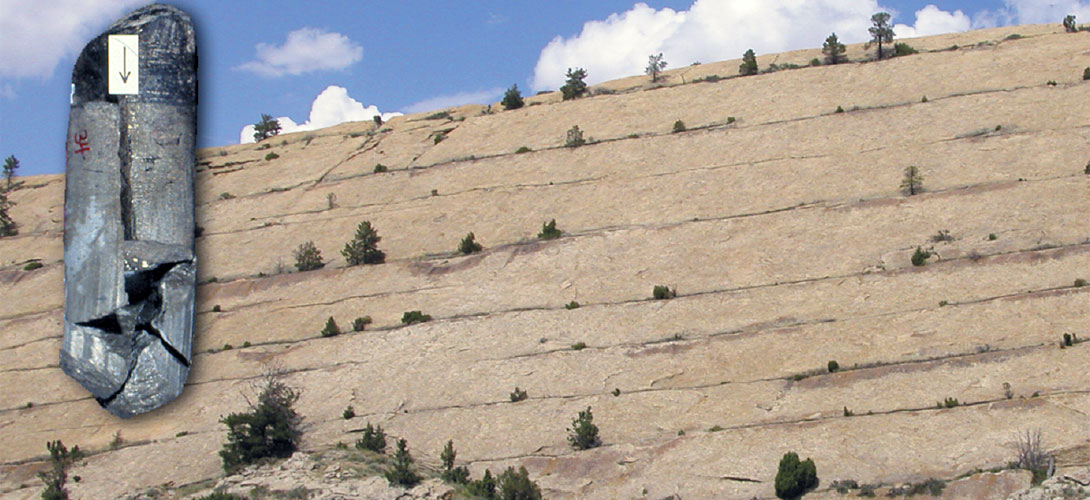
Understanding cement precipitation (diagenesis) and how it interacts with fracture mechanics provides a more complete conceptual model for understanding and extrapolating the limited data provided by core and logs and provides a sounder basis for understanding data obtained from outcrop analogs (which can be misleading). With insights from diagenesis and fracture mechanics, more effective site-specific geomechanical and statistical models can be obtained (Olson et al., 2009). These insights can be brought to bear on interpreting seismic response (Marrett et al., 2007).
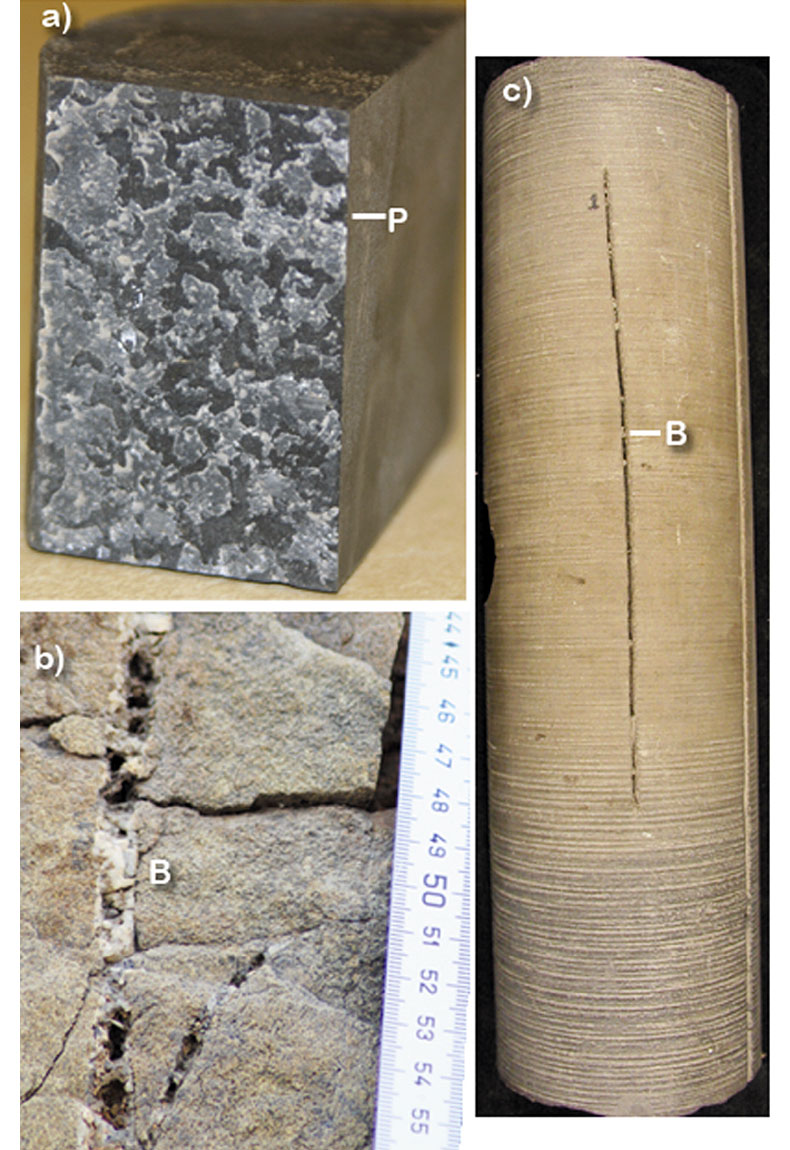
Systematic Patterns of Cement Deposits in Fractures
Although to some extent patterns of open and sealed fractures make intuitive sense—smaller and older fractures tend to be sealed—other patterns are not intuitive. For example, large fractures of the same set may be open in one bed but sealed in the next (Laubach and Ward, 2006) or open in one location but not the next (Laubach, 2003). Such patterns have obvious implications for targeting or avoiding zones for completion or for development planning, if they can be accurately diagnosed.
Cement precipitation and fracture can interact in several ways. For example, cement can influence sandstone mechanical properties, helping to localize fractures in certain depth intervals or rock types (Laubach et al., 2009). Cement in fractures can line or locally bridge between the walls of fractures. If present in large enough volumes cements can block fracture pore space and impede flow in fractures.
Considering the timing of fracture growth relative to diagenetic reactions is useful for understanding how cement fills fractures. In some cases, cement can be shown to be contemporaneous with fracture opening (synkinematic cement). The evidence for this is in local and usually isolated cement deposits that contain textural evidence of repeated fracture and cement deposition (crack-seal textures) (Laubach et al., 2004a, b). In sedimentary rocks such deposits may locally bridge the fracture, while porosity is preserved elsewhere. Fluid inclusions trapped within isolated cement deposits in otherwise open fractures record the timing and rate at which fractures open (Becker et al., 2010). Textural cross-cutting relations allow inference of the sequence of cement growth. Together with fluid inclusion information this allows reconstruction of fluid temperature and pore fluid pressure evolution during fracture opening and assessment of fracture age. This is essential information for selecting the proper geologic model for predicting the distribution of fractures away from points of observation. For example, evidence that fractures formed during folding is useful for deciding if a curvature attribute is appropriate for predicting the occurrence of fractures in the interwellbore region. Studies of these types of cement deposits show that they rarely seal large fractures.
Fracture size plays an important role in how fractures seal. The negligible volume of smaller fractures compared to their surface area means that such fractures readily fill. The fill rate depends primarily on geochemical environment, rock type, and temperature, thus degree of fill (or size of fracture filled) depends on fracture age (thermal exposure). Together, these factors result in systematic patterns of size dependent fracture fill. For Laramide fractures in Cretaceous tight gas sandstones in the Rockies, for example, fractures having apertures of 0.1 mm are commonly sealed with synkineamtic quartz, but quartz does not fill larger fractures (although the large fractures may be sealed with other mineral phases). In contrast for fractures in tight gas sandstone outcrop analogs that have experienced a much longer and hotter burial history, much wider fractures are sealed (Laubach and Diaz-Tushman, 2009).
Other processes also have a role. Simulations of quartz cementation within sandstone fractures by Lander et al. (2004) and dolomite precipitation in dolostones (Gale et al., 2010) show that porosity is a function of fracture size and the ratio of rate of net fracture opening to the rate of quartz precipitation. These models are corroborated by reconstructions of actual fracture opening histories and fill sequences (Becker et al., 2010).
Information on systematic sealing patterns can be used to modify mechanical and statistical models of fracture patterns to aid permeability estimation, simulation, and other practical assessments of fracture contributions. For fracture patterns generated by geomechanical models where aperture, length and fracture network geometry reflect subcritical crack growth, realistic fracture geometries can be generated in response to small strain loading (Olson et al., 2009). Physical connectivity in the fracture networks depends on strain anisotropy but is typically high based on trace pattern geometry. When the effects of diagenesis are added, however, where smaller aperture fracture segments are preferentially filled, connectivity and effective fracture length is significantly reduced. The effects on expected fracture contributions to producibility can be dramatic (Philip et al., 2005). Philip et al. (2005) and Olson et al. (2009) estimated equivalent permeabilities for a variety of fracture patterns generated by a geomechanical model for various values of strain anisotropy, subcritical index, and layer thickness in an attempt to discern attributes that controlled flow in networks. Under higher strain anisotropy, single set networks were generated consisting of nonpercolating, parallel fractures. These results showed that permeability was dependent on fracture cumulative length, but it was also influenced by average segment length. Patterns of the same cumulative trace length but larger average segment length had higher equivalent permeability than those with smaller average segment lengths. Imposing the effects of synkinematic cement on the fracture pattern preferentially occluded small aperture segments of the fractures, reducing average fracture length and diminishing effective permeability.
These history dependent changes in fracture abundance and length might have a perceptible impact on the seismic response of fractures: fracture sizes and abundance can control the magnitude of seismic signature and open fracture length may be related to the magnitude of velocity anisotropy, and could also affect diffraction patterns. Moreover synkinematic cement can stiffen fracture systems and prevent them from closing even where they are not oriented parallel to the current maximum horizontal stress; such fractures do not necessarily close when the fluid pressure within them is reduced (Laubach et al., 2004a). In many tight gas and shale gas fracture systems fractures may be much less prone to closure when the fluid pressure within them is reduced than is typically assumed. Multiple sets of open fractures can coexist (Laubach and Ward, 2006; Rajabi et al., in press).
Heterogeneous Patterns of Sealed and Open Fractures
Empirical evidence shows that heterogeneous patterns of infilling of large fractures by cements is a common occurrence (Laubach, 2003). Core demonstrates that sealed and open fractures having identical strike can be interspersed over vertical distances that range from a few meters or less to decimeters and over lateral distances of meters to kilometers. The mineral phases responsible for sealing large fractures are commonly carbonate minerals. The distribution of these damaging late cements may be highly heterogeneous within a field or even individual sandstone. Rock composition, stratigraphic and structural position, and basin plumbing can all affect the distribution of late cements and the pattern of sealed fractures. Understanding these controls is an important goal of ongoing research.
Production and core data demonstrate that in some instances it is the degree of cement fill in fractures rather than fracture orientation that limits fluid flow. Flow occurs only where fractures are not sealed with cement. In the absence of reliable measurements of both open fracture strike and SHmax, these features should not be presumed to be parallel (Laubach et al., 2004a). Even in the most mechanically favorable environment, precipitated cements can seal any orientation fracture (Laubach, 2003). Recognition of, and distinction among, uncemented fractures, partially cemented fractures containing cement bridges, and completely cemented fractures is essential in effective reservoir characterization and is a challenge for seismic methods. This might be feasible if mineral fill within a fracture (e.g., mineral bridges) affects shear compliance across the fracture differently than normal compliance (Sayers et al., 2009).
Patterns and scale of heterogeneity of sealed and open fractures is illustrated in Figure 3. The parameter shown is a core-based predictor (degradation index) of the location of large open and fractures; low values (<50%) predict open fractures; high values predict nearby fractures are sealed. Where large fractures are present in core in this area, the predictor has been shown to accurately identify depths having either open or sealed fractures (Laubach, 2003). Where fractures are sealed they may produce smaller effects on seismically measurable attributes such as anisotropy. The data suggest that the size of the domains having open or sealed fractures ranges in height from a few to tens of meters. Well spacing here and in most core datasets is too wide to say much about lateral dimensions except that within given sandstones shifts from open or sealed fractures may exist from one location the next. But studies of this phenomenon in outcrop analogs suggest lateral dimensions of domains having one attribute or the other that may be in the range of tens to hundreds of meters in some instances (Laubach and Ward, 2006). The dimensions of these domains of open or sealed fractures are probably sufficiently large that they are plausible candidates for creating a meaningful seismic response. Areas of sealed fractures, for example, might produce greatly reduced anisotropy.
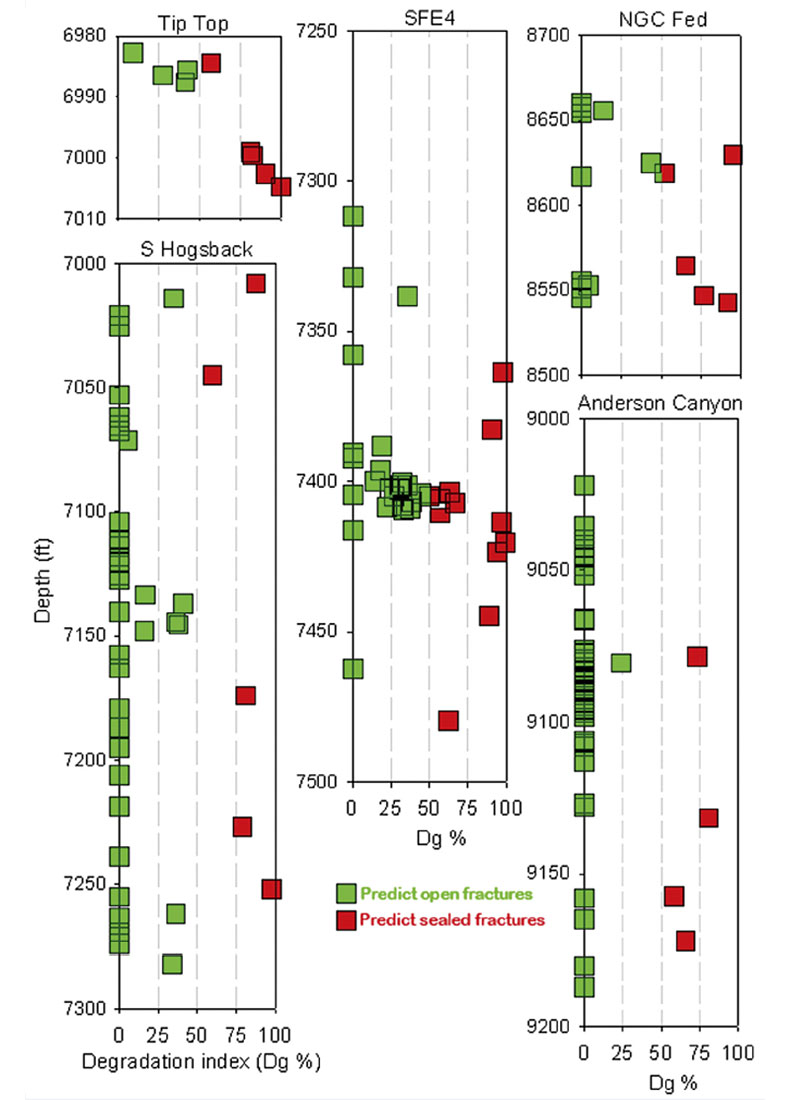
Discussion and Conclusion
Diagenesis has many potential effects on how and when fractures open and close. One key way that diagenesis modifies fracture attributes is by sealing some fractures but propping others open. Thus open fractures are not necessarily oriented parallel to the current maximum horizontal stress (Laubach et al., 2004a; Rajabi et al., in press). In part this is because even in the most mechanically favorable environment for closure, precipitated cements can prop open in any orientation without sealing or blocking fluid flow in the fractures. In many tight gas and shale gas fracture systems fractures may be much less prone to closure when the fluid pressure within them is reduced than is typically assumed. Differing amounts of isolated cement deposits in otherwise open fractures can influence seismic response, for example by modifying the ratio of normal to tangential compliance of fractures (Sayers et al., 2009).
Geological research on natural fractures challenges assumptions frequently made by geophysicists. Instead of isolated, regularly spaced, large, equally compliant fractures, the Earth presents complex clustering of fractures having a wide range of sizes and variable compliance dictated by natural cements in the fractures and the rock mass. Documenting these fracture attributes in the interwell space is a key challenge for geophysicists.
Acknowledgements
Supported by Chemical Sciences, Geosciences and Biosciences Division, Office of Basic Energy Sciences, Office of Science, Grant No. DE-FG02-03ER15430, U.S. Department of Energy and industrial associates of the Fracture Research & Application Consortium.
Join the Conversation
Interested in starting, or contributing to a conversation about an article or issue of the RECORDER? Join our CSEG LinkedIn Group.
Share This Article