Dawson Geology Graduate Society Trip 2016: Iceland and Northern Norway
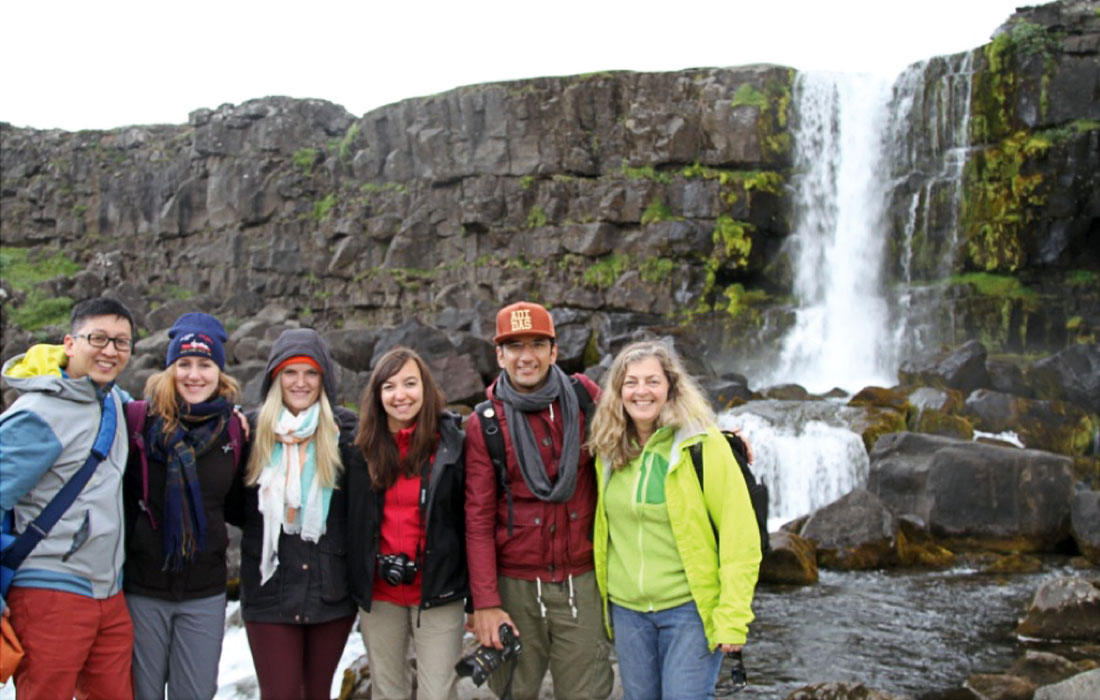
Dawson Geology Graduate Society Trip 2016: Iceland and Northern Norway
An 11 day fieldtrip was undertaken to Iceland and Northern Norway to gain an understanding of past and present geomorphological and tectonic processes that lead to the unique landscape of Northern Norway, the processes that formed the continental and oceanic crust, and the geothermal and volcanic activity of Iceland. Part 1 of this report appeared in the January 2017 RECORDER.
Participants: Taylor Campbell, Anne Hargreaves, Rachel Milligan, Laura Broom, Carlos Wong and Masoud Ali
Relationship between tectonics and landscape
The Mid/North Norwegian passive margin formed through several stages of rifting from the Late Palaeozoic to the Palaeogene. Studies show that the contrasts in landscape type occur across some of the major faults in Lofoten, such as Nappstraumen, Sortlandsundet and Raftsundet. The westernmost islands (Moskenesøy and Flakstadøy), show evidence of having younger uplifts and are characterized by high alpine landscapes. To the east, the landscape is more subdued with gently dipping surfaces, defined by flat-topped summit remnants, cut by valleys. Most of the valley and ridges in Lofoten display planar and steeply dipping slopes resembling a series of domino-style fault blocks bounded by both ESE-WSW and NNW-SSE dipping normal faults. Tilted Mesozoic basin remnants and bounding normal faults are locally preserved inside fjords. These provide markers of pre-depositional basement topography, providing a glimpse of the Mesozoic landscape (Bergh and Corner, 2015). The final stage of landscape evolution was during the Quaternary (last 2.6 Ma). During this period, cirque-glacier erosion took place alternating with valley-glacier and ice-sheet erosion. The glacier erosion that took place was in valley cuts and in zones of bedrock weaknesses, allowing the deeply incised, oriented landscape that is obvious today (Bergh and Corner, 2015) (Figure 10).
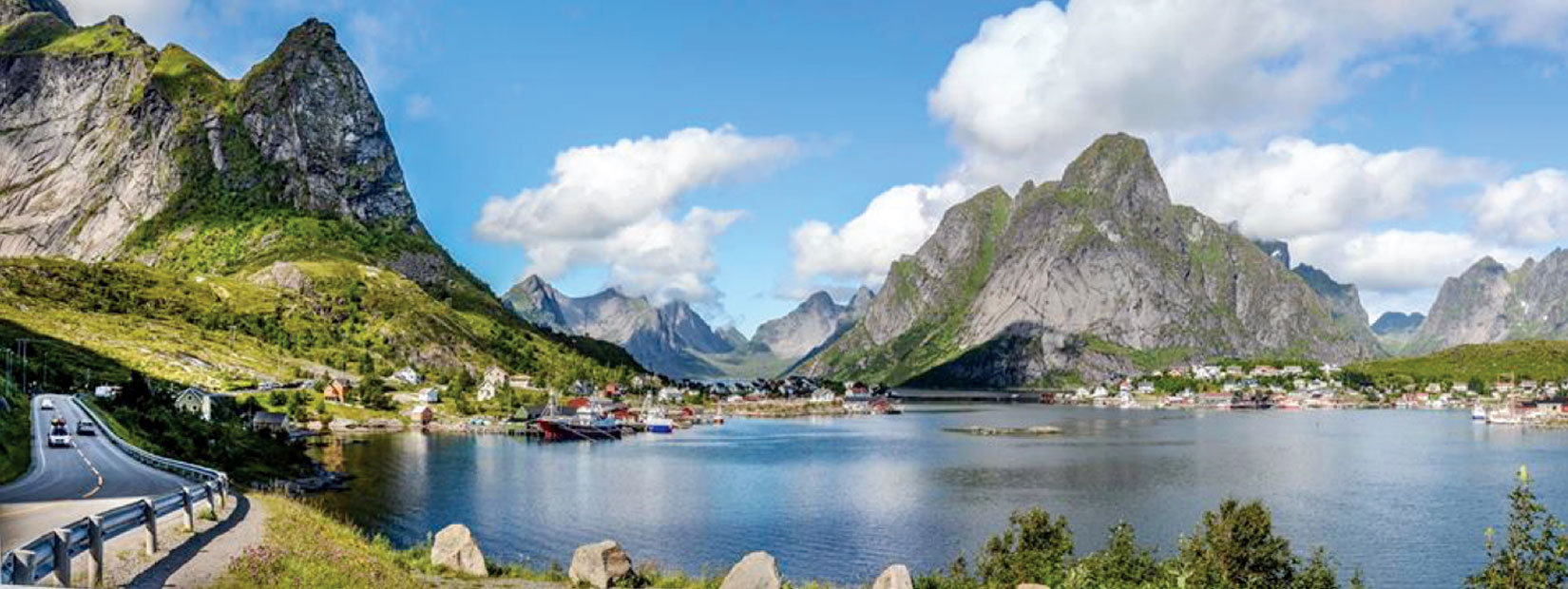
Photo by Masoud Ali, 2016.
Precambrian rocks in Lofoten are very resistant to erosion and, although substantially modified, these chains of mountains lasted during extended periods of severe glacial conditions. Lofoten is a very unique setting as an exposed ridge of lower crustal rocks that formed basement highs, on a very high and extended continental margin. All of which is a result of a combination of tectonic events and glacial erosion and weathering. Lofoten has breathtaking views and provides an exceptional opportunity to study the effects of past and present geological processes (Geological Survey of Norway, 2005).
Petroleum Systems near Lofoten
Iceland was the first stop of our trip, where we had 4 days, from July 13-July 17, 2016 to explore the unique and beautiful country. As geoscientists, experiencing Iceland first-hand is an invaluable experience. It gives a unique opportunity to view and envision what Earth was like early in its formation. It shows us the boundary of the Eurasian and the American tectonic plates with abundant glacial, fluvial and lacustrine sediments. The history of Iceland involves the interaction of both the fire of volcanoes and the ice of glaciers, during the deposition of alternating volcanic and sedimentary successions, which have been deposited subaerially, subglacially and subaqueously (Thordarson and Hoskuldsson, 2013).
Norway has a large amount of petroleum resources offshore. The continental shelf is six times larger than mainland Norway and the country has 570,000 km2 of the shelf open for exploration (NPD, 2016). Exploration is occurring near the Lofoten Islands, both southwest of the region in the Norwegian Sea and northeast of the area in the Barents Sea (Figure 11).
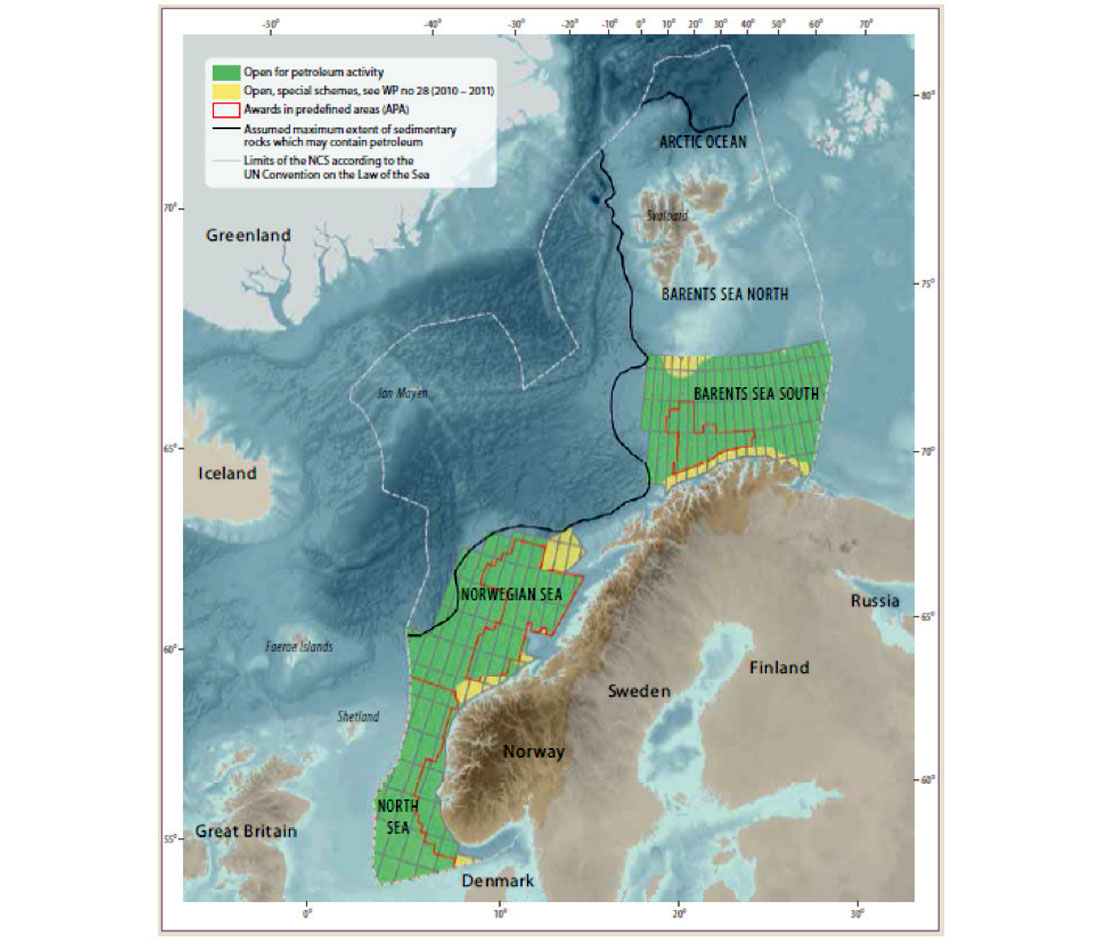
In the Norwegian Barents Sea exploration is open and ongoing. The structure of the region has been largely affected by salt tectonics and uplift. In the southeastern region the best reservoir rocks consist of Middle and Upper Triassic and Jurassic sandstones which have been interpreted as a delta plain environment with channels which flooded over the fluvial plain. Other reservoirs include Jurassic and Lower Carboniferous sandstones and Carboniferous and Permian limestones and reef structures. (NDP, 2013). Lower Triassic and Carboniferous sandstones have reservoir potential where they have not been buried deeply near the coast. Source rocks producing gas include coal horizons in Lower Carboniferous and Triassic rocks as well as organic rich shales and limestones in Carboniferous and Lower Triassic rocks (NDP, 2013). Oil prone source rocks include Upper Jurassic organic rich shales which have the potential to produce oil, but are immature and have not been deeply buried enough and Carboniferous limestones and shales which have reached a temperature high enough to produce oil and gas. Petroleum migration is assumed to be vertical in the area (NDP, 2013).
Petroleum resources occur in the Norwegian Sea offshore of the Lofoten region though the area is not open for exploration. Reservoir rocks include Triassic and Jurassic sandstones (NDP, 2010). Jurassic sedimentary rocks are sedimentary wedges on the flanks of fault blocks and there are possible sandstone reservoirs in the Upper and Lower Cretaceous deposited during uplift and erosion on the adjacent mainland (NDP, 2010). There is also the potential for reservoir rocks within sandstones of a thick Paleocene fan deposit. The source rocks in the region include organic rich shales that were both oil and gas prone and were deposited in the Late Jurassic (NDP, 2010), though in some regions of offshore Lofoten the source rock is buried too deeply to produce oil or even gas at greater depths. Coals of the Triassic and Early and Middle Jurrasic are also potential source rocks. The region has experienced extensive uplift and erosion near the coast during the Late Cretaceous and Paleogene which could have caused source rock to have been uplifted after burial causing hydrocarbon production to slow or stop and be released from the traps.
Northern Norway geology and landscape
The primary reason for the unique form of the coastal Norwegian landscape is uplift which took place for most of the Tertiary (2-66 Ma). The rocks from the Paleozoic extend from the land seawards, far beyond the present day coast line. Overlying Paleozoic rocks of the Caledonian mountain chain, in the coastal areas near the Arctic Circle, are now deeply eroded. Relics can be found in the high peaks along the coast and mountain summits. The characteristic form of the coastal landscape is due to the cold climate with frost-weathering together with erosion from waves, rivers and glaciers. Fjords and strandflats (large, flat-lying areas present along the coast), were formed primarily at the end of the Tertiary and also in the Quaternary, when large ice sheets covered the country (Bargel, 2009).
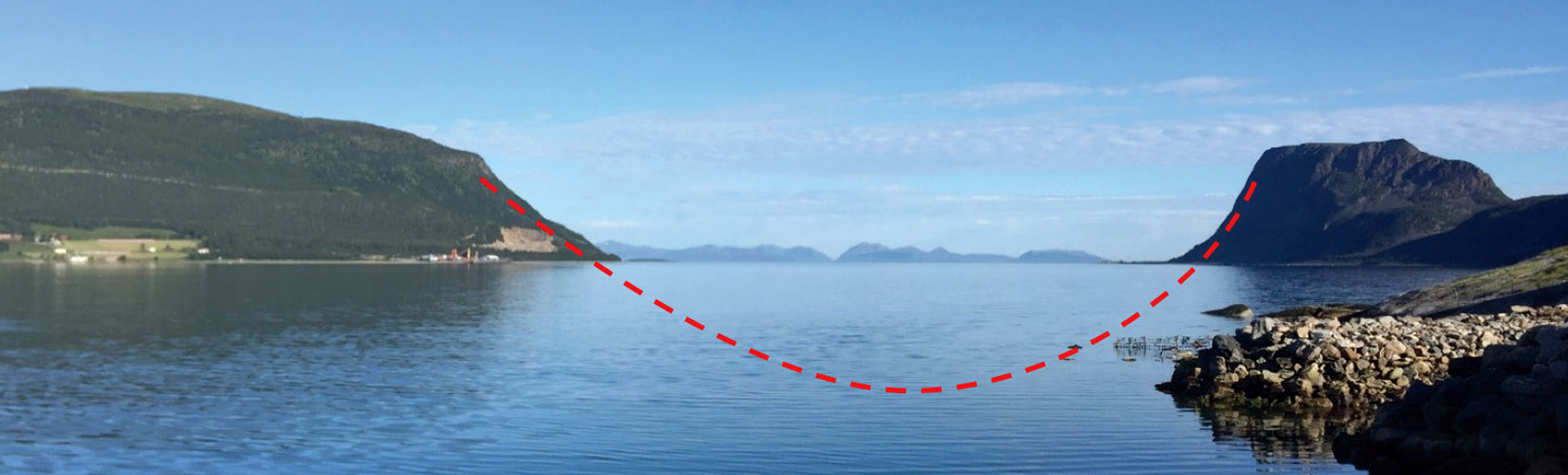
Valleys and fjords
Old river valleys developed in joint zones and along faults, rock boundaries and other weak zones in the bedrock. These are typically V-shaped and were later excavated by glaciers, making them more U-shaped. Fjords are long narrow inlets with steep cliffs, usually carved by glacial erosion. There are many fjords along the Norwegian coast, which we observed on our very winding drive from Leknes, Lofoten to Harstad, where we had to drive around all of the fjords. Glaciers carved river valleys that are deeper than sea level, and connecting them to the Norwegian Sea. We saw these fjords all over the Norwegian coastline. Some of these fjords were as deep as 1.3 km below sea level. During the Mesozoic there was considerable deposition of sediments in which created large reservoirs for oil and gas today. Most of the sediments deposited prior the glaciers were removed as the glaciers carved these river valleys, exposing these U shaped valleys and fjords in the Norwegian coastline (Ostergren and Rice, 2004).
Norway was covered by ice sheets as thick as four kilometers, 2-3 Ma. The effects of the ice sheet weight pushed down the Baltic Shield. As the ice sheets melted, the Baltic Shield continuously rebounded and still rebounds today at a rate of 1 m per century (Ostergren and Rice, 2004) under isostatic rebound.
It was a great opportunity to be able to see up-close how geomorphological features formed by glaciers, such as fjords, can have such a vast effect on the landscape. Overdeepening fjords are great examples of glacial erosion exposing the bedrock and full scale outcrops. These geological features are great for the studies of various fields, such as petroleum geology, hydrology, and engineering. These outcrops are great for gathering information and to develop infrastructures for foundations and tunnel construction, applications for groundwater resources management and to identify locations of potential oil fields. These fjords can also have a great potential for implementing hydroelectric power generation, a form of green electricity that Norway implements and supplies to their country and other counties. In these fjords they have discovered cold water coral reefs from the northern parts of Norway to the southern parts of Norway. This ecosystem is still not well understood, but is very important for the fishing industry in Norway.
Acknowledgments
The Dawson Geology Graduate Society students who had the opportunity to participate in this field trip would like to acknowledge and thank everyone who contributed to the funding of our amazing field trip. We would like to particularly thank the Canadian Society of Exploration Geophysicists Foundation, Shell Canada the Dalhousie Student Union, the Dawson Geology Graduate Society and the Earth Science Department for all of their generous contributions. We would also like to thank Carla Dickson, who helped in applying for funding and in the organization of our trip. Our trip was a once in a lifetime opportunity to travel with great, like-minded friends, all who share a love and interest for geology and geophysics.
References
Bergstrøm, B., Olsen, L., Sveian, H., Gustavson, M and Gjelle, S. 1995. Revised by Bargel, T.H. 2009. Geology and landscape around the Arctic Circle in Norway. Geological Survey of Norway. [online]. Available from https://www.uef.fi/documents/640649/725289/Review_Norway_Part_I.pdf/84f6776b-4f96-4cd9-8d17-922db3538036[accessed August 8, 2016].
Bergh, S.G., and Corner, G. 2015. Proterozoic igenous and metamorphic rocks: a template for Mesozoic-Cenozoic brittle faulting and tectonic inherited landscapes in Lofoten-Vesterålen, North Norway. 33 IGC, The Nordic Countries.
Directorate, Norwegian Petroleum. 2016. Petroleum resources on the Norwegian continental shelf 2016. [online]. Available from www.npd.no [accessed August 15, 2016]
Directorate, Norwegian Petroleum. (2013). Petroleum resources on the Norwegian continental shelf 2013. [online]. Available from www.npd.no [accessed August 15, 2016]
Directorate, Norwegian Petroleum. (2010). Petroleum resources in the sea areas off Lofoten, Vesterålen and Senja. [online]. Available from www.npd.no [accessed August 15, 2016]
Emslie, R.F., and Hunt, P.A. 1990. Ages and petrogenetic significance of igneous mangerite charnockite suites associated with massif anorthosites, Grenville province. The Journal of Geology 98: 213-231
Geological Survey of Norway. 2005. Geological Survey of Norway. [online]. Available from http://www.ngu.no/FileArchive/227/2005_086.pdf [accessed August 8, 2016].
Google Maps. 2016. Leknes, Norway to Harstad, Norway [Street Map]. Available from https://www.google.ca/maps/dir/Leknes,+8370,+Norway/Harstad,+Norway/@68.4622334,12.857739,7z/data=!3m1!4b1!4m13!4m12!1m5!1m1!1s0x45de12acaf43485f:0x5c5fb3e75f9ff61d!2m2!1d13.6096372!2d68.1465068!1m5!1m1!1s0x45dc7c3d37b4255b:0x6e89b5c524c6757e!2m2!1d16.5414503!2d68.7986342 [accessed August 10, 2016].
Google Maps. 2016. Harstad, Norway to Bodø Airport. [Street Map]. Available from https://www.google.ca/maps/dir/Harstad,+Norway/Bod%C3%B8+Airport,+Olav+V+gate,+%20od%C3%B8,+Norway/@68.0237767,14.3549811,8z/data=!3m1!4b1!4m13!4m12!1m5!1m1!%20s0x45dc7c3d37b4255b:0x6e89b5c524c6757e!2m2!1d16.5414503!2d68.7986342!1m5!1m1!%20s0x45df10516a4a91b5:0x63df55226f896eea!2m2!1d14.3622351!2d67.2683109 [accessed August 10, 2016].
Jousset, P., Haberlandb, C., Bauer, K., and Arnason, K. 2011. Hengill geothermal volcanic complex (Iceland) characterized by integrated geophysical observations. Geothermics, 40: 1–24.
Lynne, B.Y., 2013. Siliceous sinter: and early exploration tool and direct link to a geothermal reservoir. Proceedings of the 38th workshop on geothermal reservoir engineering, Stanford University, SGP-TR-198
Markl, G. 2001. REE constraints on fractionation processes of massive-type anorthosites on the Lofoten Islands, Norway. Mineralogy and Petrology 72: 325-351.
Martin, R.F. 2012. The petrogenesis of anorogenic felsic magmas and AMCG suites: Insights on element mobility and mutual cryptic contamination from polythermal experiments. Lithos 151: 35-45.
McLelland, J.M., Selleck, B.W., Hamilton, M.A., and Bickford, M.E. 2010. Late- to post tectonic setting of some major proterooic anorthosite-mangerite-charnockite-granite (AMCG) suites. The Canadian Mineralogist 48: 1025-1046.
National Energy Authority of Iceland. 2009. Geothermal development and research in Iceland. Orkustofnun.
Norðdahl, H., Ingolfsson, O, and Ivarsson, G., 2008. Geology of Iceland, 33 IGC Excursion 5 Fieldtrip guidebook, July and August 2008.
Ostergren, R.C., and Rice, J.G. 2004. The Europeans: A Geography of People, Culture, and Environment
Saemundsson, K. 2008. Geology and gradient wells. Presented at Short Course III on Exploration for Geothermal Resources. Organized by UNU-GTP.
Summitpost.org. 2015. Summitpost.org. [online]. Available from http://www.summitpost.org/presten/718136 [accessed August 8, 2016].
Thordarson, T. and Hoskuldsson, A., 2013. Classic Geology in Europe 3, Iceland, Second Edition. Dunedin Press.
Share This CSEG Report