Some quick facts regarding Canada’s share of the world’s fresh water, courtesy of (Environment Canada, 2018). Canada has 20% of the world’s fresh water, but only 7% of the world’s renewable fresh water. The difference can be attributed to “fossil” water that is trapped in ice, aquifers, and lakes, and therefore is not considered renewable. Furthermore, 85% of Canada’s renewable water flows north into the arctic and is not reasonably accessible to Canada’s densely concentrated southern strip. But in the big picture we are blessed when it comes to water – consider that Canada’s ~36 m people represent less than half a percent of the world’s 7.8 billion people, so we’re literally swimming in fresh water on a per capita basis. Other parts of the world are short of water, some desperately, and as human populations inexorably rise, desalination of sea water is an increasingly critical field of science and technology. Figure 1 is a global water stress map, showing the ratio of annual water use (upstream consumption) to available renewable water supply.
Currently about 1% of the world’s people rely on desalination (often abbreviated to desal) for their water supply, but the expectation is that this will rise sharply in the coming years. A couple of examples of countries leading the way are Kuwait, which gets 100% of its fresh water via desalination, and Singapore. Singapore has a long and fractious history of getting much of its water supply from neighbouring Malaysia. The threat of political issues causing a disruption in its water supply motivated the small but densely populated island nation to pursue a long-term goal of water self-sufficiency. They have achieved this with a holistic approach that includes innovative water catchment, storage, reclamation/recycling and desalination technologies. Figure 2 shows Singapore’s 3rd desal plant, a reverse osmosis operation delivering 30 million gallons of freshwater per day (they have since built a 4th and number 5 is in the works). I expect all nations with access to sea water that experience freshwater shortages will be investing in desalination infrastructure in the coming decades, following the lead of countries like Singapore and Kuwait.
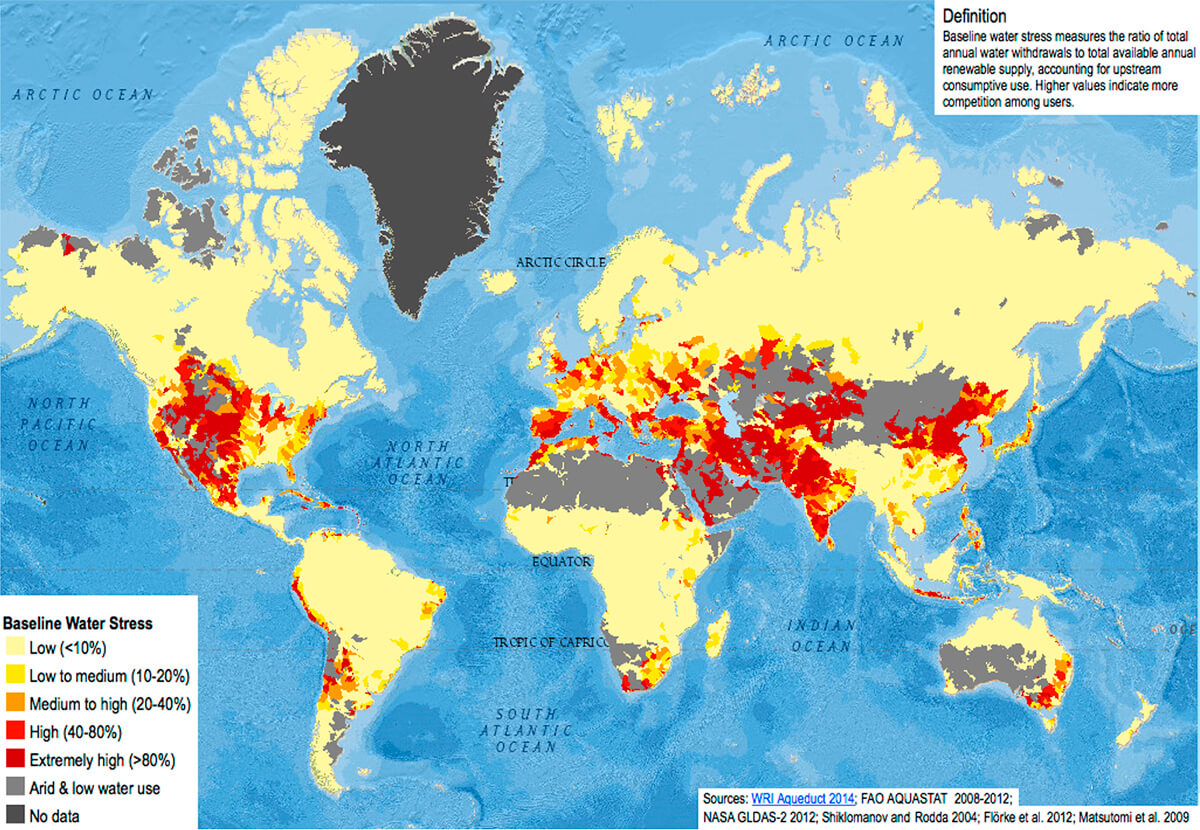
In this article I will specifically look at the ways we remove salt from sea water to produce potable fresh water, not the more general meaning of desalination which can include the removal of salt minerals from soil and other liquids and solids. As I like to do, I will start with a quick look at historical desalination methods, then an overview of the economic factors at play, and end up with a summary of desalination methods.
Desalination in the past
The human body is simply unable to process salt water. The kidneys produce urine that is less salty than sea water, so the body requires fresh water to dilute the sea water in order to process it. In the absence of an external supply of fresh water, the body therefore would run out of internal water in its attempt to deal with the sea water, and become severely dehydrated to the point of toxicity. Surely many times in the distant past ancient humans were frustrated by situations where there was a lack of fresh water and an abundance of salty water. At some point they must have stumbled on primitive methods of distillation. The very oldest surviving texts of various ancient cultures such as Sumerian, Babylonian, and those of China and the Indian subcontinent, whether it be scrolls, tablets or whatever, indicate use of distillation techniques. There is a section in Exodus 15:22–26 that some scholars believe refers to a type of distillation method:
When they came to Marah, they could not drink the water of Marah because it was bitter; therefore it was named Marah. And the people grumbled against Moses, saying, “What shall we drink?” And he cried to the LORD, and the LORD showed him a log, and he threw it into the water, and the water became sweet.
Around 2,400 years ago, in Aristotle’s Meteorologica, he most definitely refers to distillation:
Salt water when it turns into vapour becomes sweet and the vapour does not form salt water again when it condenses.
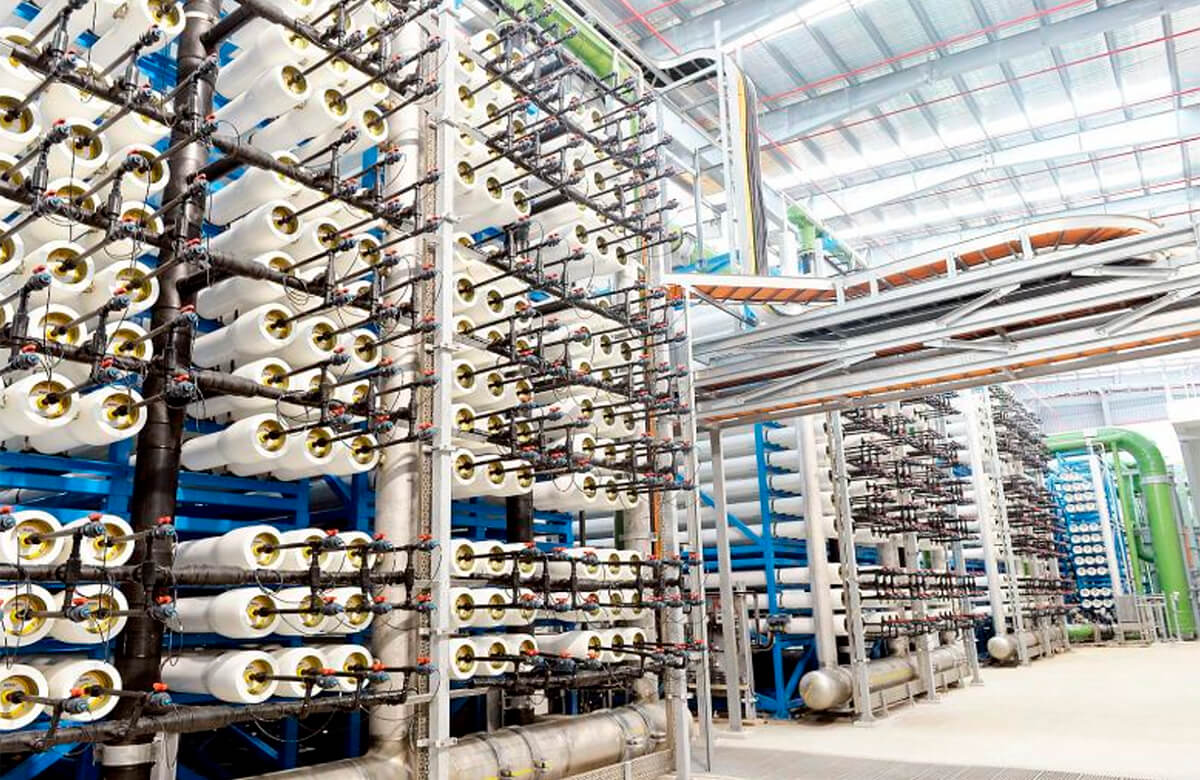
All the ancient sea-faring cultures of the world developed ways to boil sea water on board, and then capture and cool the steam produced. In one of my first articles (Kuhn, Ancient Chinese Drilling, 2004) I describe how between 1,500 and 2,000 years ago there was a salt industry in Szechuan, China that was drilling deep wells to coproduce brine and natural gas, with the latter being used to heat the brine to produce salt. When I wrote that I was focused on the salt extraction aspect, but I suppose it would have been easy for them to also cool and condense the steam to produce water, if it was needed. At any rate, people have been producing freshwater using small scale distillation methods for thousands of years. Industrial scale water distillation operations however are a recent development, really only starting in the 1930’s when the abundant and concentrated energy available from oil began to be used to produce freshwater in the parched Middle East.
Economics of desalination
Separating and removing salt from sea water is an energy intensive process. The energy required to produce potable water from a local freshwater supply such as a lake, river or well is in the vicinity of 0.2 kWh/m3 or lower. By contrast, experts estimate the absolute (i.e. theoretical) minimum energy required to desalinate sea water is 1 kWh/m3, but actual efforts rarely achieve rates below 2 kWh/m3. So the energy cost, and therefore in most cases the monetary cost, to desalinate seawater is at least 10 times greater than that of freshwater production.
On top of that, many areas of human population are inland, at great distances from seawater, and usually at elevations greater than sea level; the cost to transport desalinated water to inland regions adds considerably to the total cost. For some inland cities, the effort to pipe desalinated water in from the coast could more than double the total cost. Lack of water and poverty often go hand in hand, and this means that many of the world’s most water-poor regions cannot afford the capital investment required for desalination infrastructure. The high cost of desalination represents one of the biggest challenges facing its use, and therefore there is considerable effort being put towards newer technologies that can lower these costs. Many of these newer technologies try to make as much use as possible of low-cost natural energy sources such as wind, sun, and waves.
Desalination methods
Desalination methods can be grouped into two broad categories, thermal-based and membrane-based. Pretty well all the methods used currently fall into these two categories, although there are some exceptions. A summary of the more common methods follows.
Solar evaporation
Solar evaporation is of course nature’s way of distilling water. The sun heats salt water in seas and oceans, evaporation occurs, and the water vapour, “sweet” in the words of Aristotle, eventually condenses and falls back down as freshwater rain. Primitive distillation methods exist that add some ways to, first, prevent the water vapour from escaping and then, second, cause it to condense and be collected. This could be as simple as draping a tightly knit sheet of fabric over a ditch of salty water, allowing the sun’s rays to heat it, and then catching the drips of water as they condense on the sagging sheet.
Modern, industrial scale versions of this method, termed solar thermal powered desalination, do exist. Solar humidification is one such modern method. Large solar sills similar to greenhouses are built, where sunlight passes through sloping glass or plastic covers over large troughs of salty water. The water evaporates, condenses on the slightly cooler covers, and then drips down into freshwater collection troughs. This method is not really able to produce the volumes of water that people require for their everyday needs, but it is very suitable for agricultural uses.
Another obvious way that the sun’s power can be harnessed is through the use of solar panels (photovoltaic cells), which produce electricity that can then be used to power, or partially power, several of the other methods described here.
Evaporation
Wikipedia includes evaporation in its list of desalination methods, and uses IBTS Greenhouse as an example, claiming a desalination energy cost of 0.45 kWh/m3. I detect bullshit. Rather than a scientific explanation of what they are doing to achieve such an unbelievable rate of efficiency, instead there is a word salad that includes lots of buzzwords like systems integration, closed cycles, plus flattering comparisons with conventional desalination methods.
I suspect the Wikipedia entry on IBTS Greenhouse is mainly a PR effort. As far as I can tell, these huge agricultural installations (10 km2 is mentioned as a typical size) are suited for desert environments, make use of available wind and solar polar, and integrate many ways to efficiently use and preserve water, including very advanced recycling methods, especially using waste water to irrigate the greenhouses. But as far as the desalination part goes, I believe they employ solar humidification as described above, plus standard wind and solar powered methods.
I do know there are other ways water vapour can form besides evaporation, for example sublimation. Sublimation occurs when ice goes directly from solid to vapour, something that early prairie pioneers used to dry their washing on clotheslines in the winter. I am not aware of any desalination method that exploits sublimation; however the next section covers a method that does involve freezing and ice.
Freeze-thaw
I could not find any industrial freeze thaw desalination operations, but I did find a report (Boysen & Stevens, 2002) on a large-scale test project of this method in Devils Lake, North Dakota. This small city is located near a chain of saline lakes, and they wanted to test the viability of using the freeze thaw method to provide the town with fresh water. The method is simple – the salty water is sprayed onto pads during the winter, and mounds of low salt-free ice build up. In the spring they melt, providing the fresh water.
In this test they produced 3.68 million gallons of water with a salinity of 458 ppm total dissolved solids. For perspective, seawater has a salinity of about 35,000 ppm, and less than 500 ppm is considered pure water. The study concluded that a commercial plant would be able to deliver 93 million gallons of raw water (still requiring normal processing, but actually higher quality than the town’s current well water supply), which represented about ¼ of the town’s annual demand, at a cost of USD 1.30 per m3. By comparison, a state-of-the-art desalination plant in Carlsbad California was delivering freshwater for about USD 1.75 per m3 in 2019 (not adjusted for inflation).
As a footnote, a Devils Lake desalination plant did go into operation in 2010, but it is a reverse osmosis plant partly powered by solar panels. While perhaps cheap, I suspect the seasonal nature of the freeze thaw method makes it a logistically unattractive investment, but it’s interesting, and who knows, maybe someday it’ll come in handy!
Vacuum distillation
I wouldn’t view this as a desalination method in and of itself, rather it is a distillation trick that allows the use of low temperature waste heat from a variety of industrial processes, including power generation. For example, many nuclear plants are located on coastlines, making them ideal candidates for auxiliary desalination distillation operations using this method.
The lower the ambient pressure a liquid is in, the lower the temperature at which it turns into vapour. So, if salt water is placed in a low-pressure vessel, it will boil at a lower temperature. I should note there is a freeze thaw/vacuum distillation hybrid method where water is frozen in a vacuum using an artificial cooling method, but otherwise similar to the technique in the previous section.
Multi-stage flash (MSF) distillation
This method used to be very popular, and somewhere between a quarter to a half of the world’s desal plants use MSF. However, reverse osmosis is more efficient, and no new MSF plants are being built anymore.
The MSF distillation method (Figure 3) is scientifically interesting – a really creative application of Boyle’s Laws. It is made up of a series of chambers, with each chamber maintained at a different pressure, getting successively lower from the start to the finish of the system. The salty water is brought into the system through heat exchange tubes that run through the tops of all the chambers, coming in at the end and going all the way to the beginning where there is a heater. By running backwards through the system, the brine is preheated prior to entering the heating chamber, where its temperature is brought up to just below its boiling point. It then enters the first chamber which is at a slightly lower pressure; a small amount of water flashes into steam and condenses when it comes into contact with the heat exchange tubes, and drips down to be collected. The remaining briny liquid, now at a slightly lower temperature due to the heat lost through condensation, flows into the next chamber; this second chamber is at a lower temperature calibrated to cause another small evaporation, and the process is repeated. This goes on until approximately 85% of the water has evaporated, condensed and been collected. A typical MSF desal plant can contain anywhere between 10 and 30 chambers with a ~2C temperature difference between each of them.
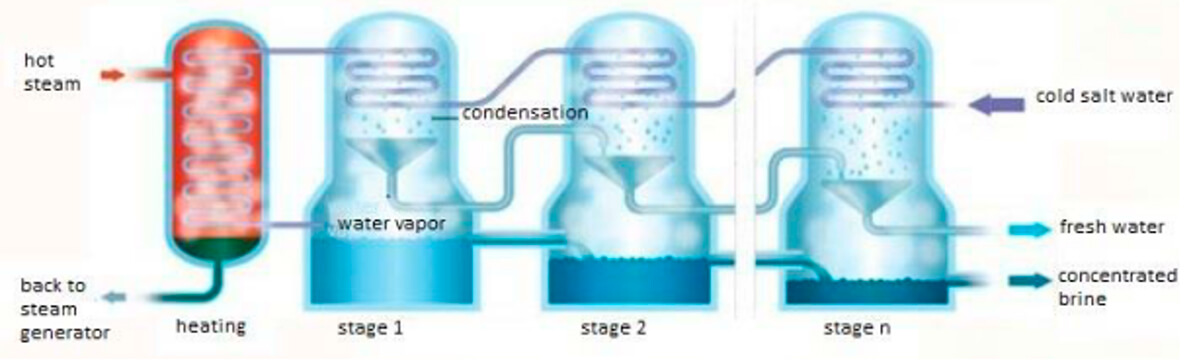
This method is also very suited to operate in concert with industrial operations that generate excess heat. It was very common for MSF desalination plants to be built alongside cogeneration power plants, as this type of power plant produces water that needs to be cooled. If you’re interested in how cogen power plants work, check out my article on them (Kuhn, Cogeneration, 2013); they are somewhat similar to MSF desalination in that they use stages to squeeze the maximum amount of electricity out of their heat source.
Multiple-effect distillation (MED)
This method (Figure 4) is similar to MSF distillation. It also consists of a series of chambers of descending pressures and temperatures, connected by tubes, but in this method the steam is carried inside the tubes, and the brine is sprayed onto the outsides of the tubes. Before the first stage, brine is heated until it turns to steam. This steam is carried inside tubes that run into the first chamber, where briny water is sprayed onto the hot tubes. The steam thus generated by contact with the hot tubes is captured and fed into the same steam pipes which then run into the second chamber, where more briny water is sprayed onto the outside of the tubes and turns into steam. And so on for several stages.
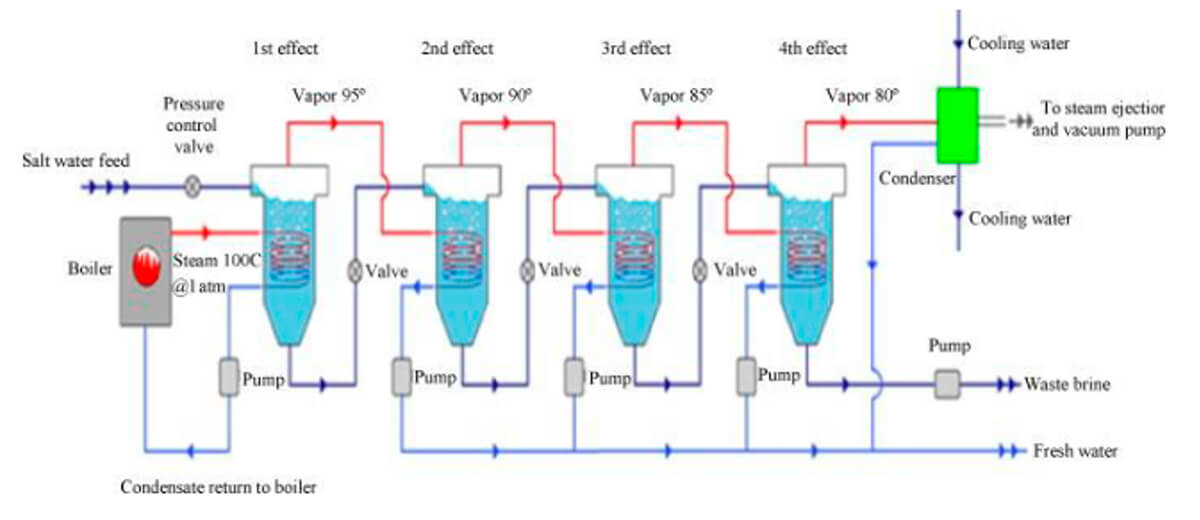
MED is the most thermodynamically efficient thermal desal method, and there are various ways to increase the efficiency, generally at a cost of a more expensive plant and/or more time taken to complete the process. Tubes can be made thinner and longer, more stages can be built into the system, and the briny water left behind in one stage can be used as the input to the next stage, instead of preheated fresh brine.
Vapor-compression distillation
I must admit that I find it hard to understand the steps involved in this method of desalination, so I’ll keep it brief. The key aspect is that instead of directly heating briny water to cause evaporation, vapour is first created, and then is compressed; this step is usually powered by electricity. The hot, compressed steam is then sent through tubes immersed in a low-pressure bath of briny water. When the steam’s heat transfers through the tube walls into the brine, some of the brine evaporates, and distillation occurs. I don’t believe this method is particularly efficient, but it is suitable for small scale applications such as luxury seaside resorts that require their own desal plants. All that is required is a small plant, electricity, and a supply of sea water.
All these thermal methods employ complex, carefully calibrated combinations of temperature and pressure, to most efficiently achieve distillation in very controlled way that minimizes unnecessary heat loss. The subtle advantages of this method over that method are probably only fully understood by scientists and engineers expert in thermodynamics. But at their most basic level, they can be distilled (pun intended) down to three steps – heating, evaporation, and condensation.
Reverse osmosis (RO)
This method uses a membrane to separate the salt from the water it is dissolved in, so it is really a physical filter operating at a microscopic level. A pressure differential causes the smaller water molecules in the brine on the higher-pressure side to pass through the membrane to the lower-pressure side, leaving the larger salt molecules behind.
RO membranes are sophisticated composites typically made up of three layers (Figure 5). At the top (the infeed side) is a thin-film aromatic polyamide which acts as the filter. The defining characteristic of this film is that it is relatively high permeability, but it has really small, consistently sized pores that very effectively bar unwanted particles from flowing through. The other two layers primarily provide structural support.
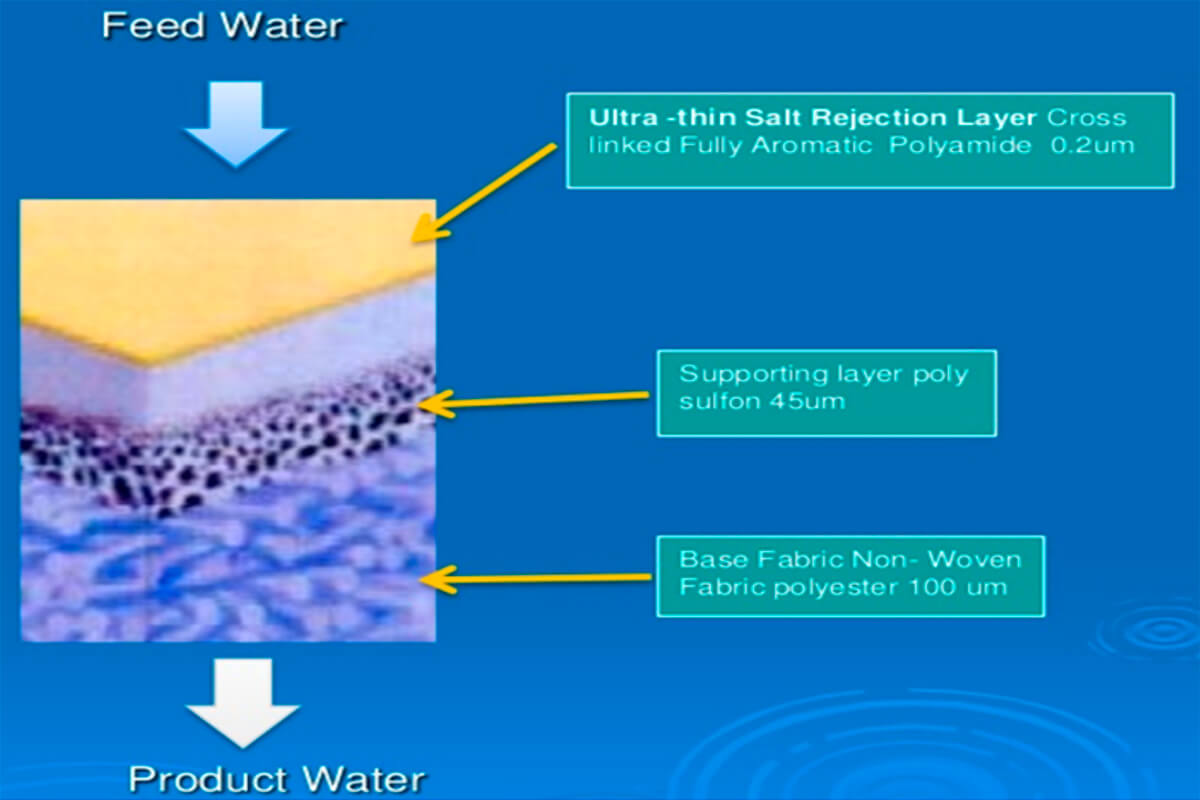
A double-sided RO membrane is usually deployed inside an infeed tube, wrapped spirally around a freshwater (permeate) outflow collection tube. The lower left part of Figure 6 nicely shows the way the brine is forced through the polyamide film on both sides of the double-sided membrane, and then is channeled spirally towards the centre collection tube. The salty water that does not pass through the membrane just continues down the outer inflow pipe as a salty brine effluent. Incidentally, the cylindrical filers you see below kitchen sinks are RO filters, usually combined with other stages of filters.
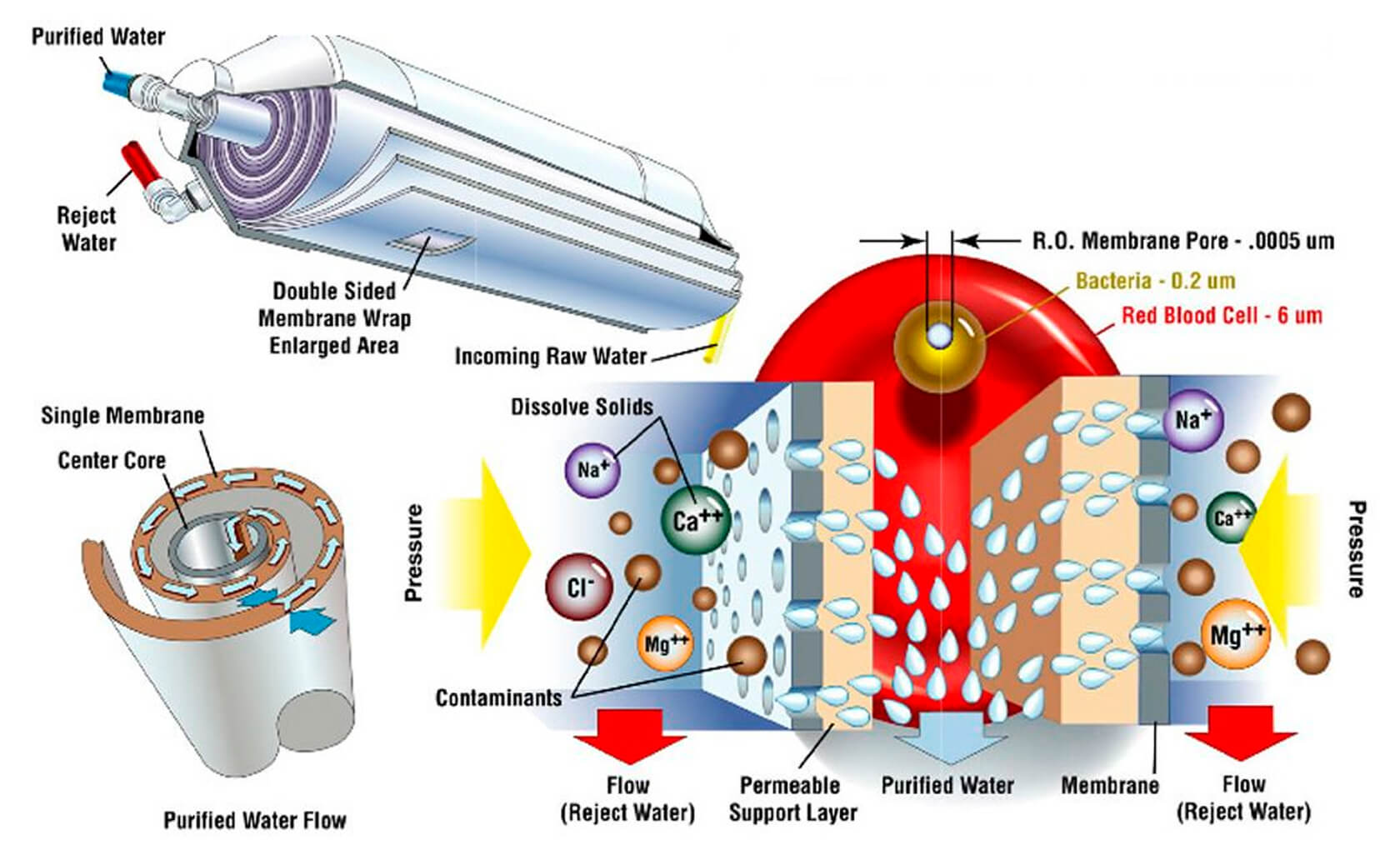
The RO process faces three main challenges. First, the polyamide films are susceptible to damage and impairment caused by scale (mineral build up) and oxides such as chlorine; second, they are prone to fouling by algae and other organisms; and third, they periodically need to be flushed and cleaned out. The first two problems are dealt with by various preprocessing steps such as mechanical, biological, and chemical filters, acid treatments, etc. The third challenge is just a reality that must be dealt with – typically RO desal plants shut down periodically to flush their filters with fresh water and special treatments. Note that flushing the membranes creates a toxic effluent, which is often piped directly into the nearby ocean or sea, causing significant environmental damage. A lot of research is going into ways to better overcome these three challenges, as the greatest cost and efficiency improvements are expected in these areas, not to mention development of more environmentally friendly processes.
The main advantage of the RO method is that it requires less energy than the thermal methods. It is amenable to the use of natural power sources such wind, solar or wave generated electricity. One interesting type of RO plant uses solar panels to generate its electricity. This electricity, when available, is used to pump sea water into storage tanks on top of nearby hills. Gravity is then used to provide the pressure required to drive the RO process, a neat way to store the intermittently available solar energy and allow the plant to run on a continuous basis. The downside of membrane desalination is that the plants are expensive to build, and the membranes, while dropping in price, are expensive and need to be replaced quite often.
Electrodialysis membrane
This method uses a different type of membrane technology that is the opposite of all the other distillation methods – instead of pulling fresh water out of the briny inflow, the salt is pulled out, leaving freshwater behind. Salts are either positively charged (e.g. sodium) or negatively charged (e.g. chloride). The system is made up of a stack of electrodialysis cells that use a potential difference to pull the charged salts out of a brine (diluate) input, through an ion exchange membrane, into a concentrate. The input liquid flows through this stack which alternates between cells with a positively charged anode – which pulls the negative chlorides through an anion exchange membrane – and cells with a negatively charged cathode – which pulls the positive sodium molecules through a cation exchange membrane. The membranes prevent the salts from flowing back into the progressively fresher water. After passing through several of these alternating cells, the desalination is complete.
The electrodialysis method is not as energy efficient as RO, but it has certain advantages. It is more robust and can handle contaminated input water better than RO, making costly preprocessing less important, and a more robust solution for places where the quality of the input water is questionable. In order to prevent scale buildup, the polarity of the cells is typically flipped on a periodic basis. However, it must be mentioned that certain substances such as calcium and magnesium do tend to foul ion exchange membranes, so often preprocessing is required. Recently, desalination plants that integrate the RO and electrodialysis methods are being built, designed in such a way that the inherent weaknesses of each method are minimized, and the strengths maximized.
Membrane distillation
I wanted to mention this method briefly, because it is an interesting hybrid approach that utilizes both membrane and thermal components. A polyamide membrane sits between a cold input tank and a warmer output tank. The temperature difference creates enough of a pressure difference to push (or pull depending on how you look at it) the water through the membrane.
Wave-powered desalination
There are numerous off-the-grid coastal communities that face freshwater shortages. A number of protype wave-powered desalination systems suited to such places have been developed, and I will describe two
The Wave2O system (Aquatech, 2019) developed in Boston uses seafloor mounted paddles to drive water pumps. The paddles are located at a depth where the back and forth wave action imparted on the paddles is at a maximum. The pumps take sea water up to shore for some preheating, and then on to a RO desalination system. The designers claim to be able to produce freshwater for USD 1.30 per m3, without the need for any electricity. I assume the preheating is done with solar power, and or the paddles also generate some electricity.
The CETO system (Verdict Media Ltd., 2020) (Figure 7) is actually designed to produce electricity, but it can also be used for desalination. Buoys fully submerged in 20 m to 50 m of water move up and down with ocean swells, driving large pumps. The pumps push high pressure water through shore-based turbines, generating electricity. Alternatively, the water can be pushed through a RO system similar to the Wav2O one. I believe an Australian naval base on an island near Perth is using this to provide freshwater. I suppose the power could be used to supplement or drive entirely, some of the other methods discussed here.
Conclusion
To wrap up, two main points to consider. Desalination technology is likely to be critically needed by future generations of humans. There are two main ways to create freshwater from salty sea water – distillation (thermal) and filtering (membrane) – with lots of room for improvement, so this should be an active area of scientific R & D.
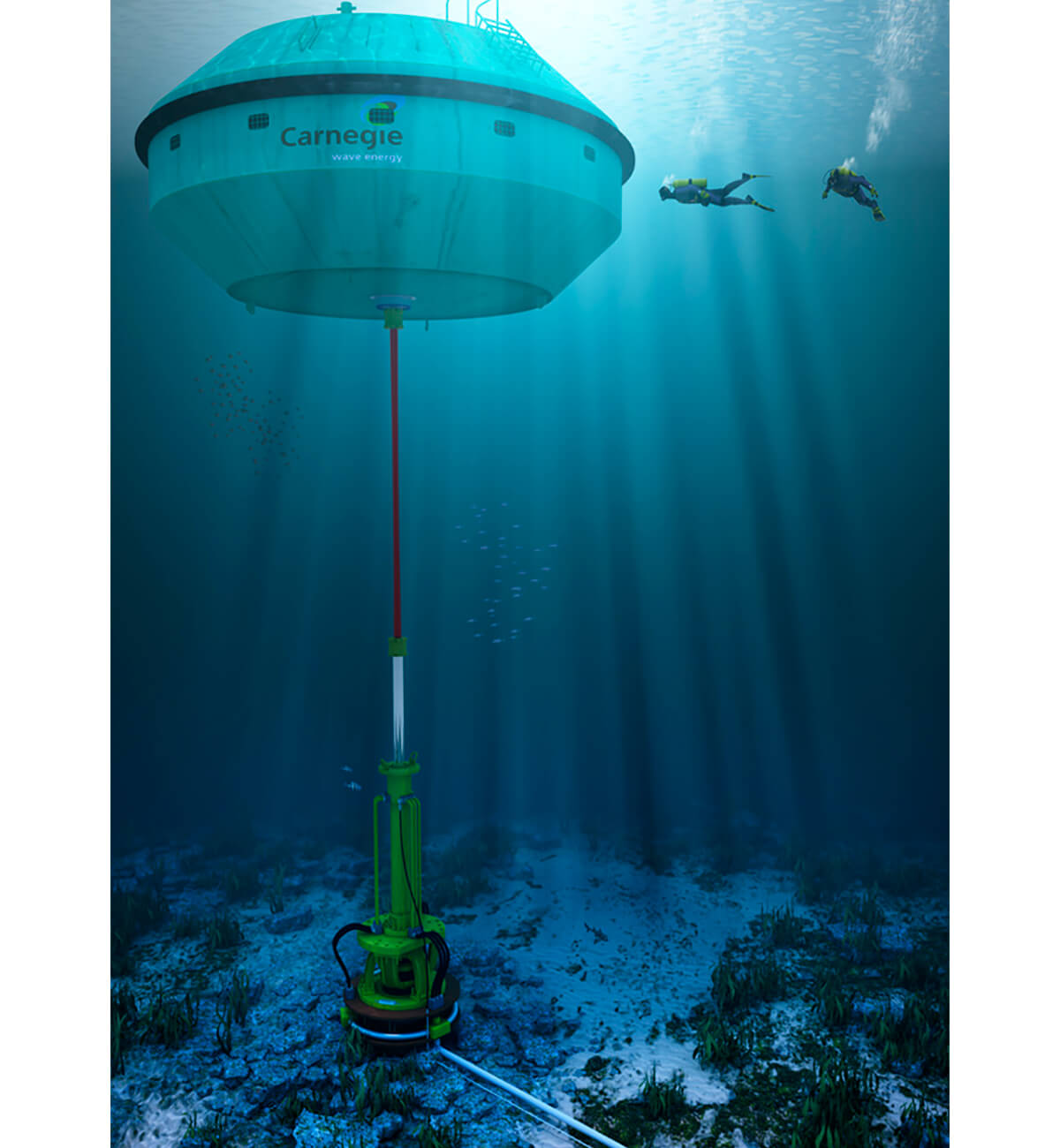
Share This Column