Our work demonstrates that positional data derived from the GPS receivers embedded in wireless seismic receiver nodes can achieve horizontal positioning quality which complies with the minimum standard required for most land-based seismic data acquisition operations. While the original purpose of the GPS receivers within seismic receiver nodes was to derive accurate timing data so that seismic data could be extracted from receivers to coincide with the timing of T0 for the seismic source, we demonstrate that it is also possible to derive the required level of horizontal position quality from these data.
Introduction
The advent of wireless nodal receiver technology opens up a host of new possibilities for seismic survey design and acquisition. These include potential step-change improvements in quality as well as opportunities for enhanced efficiency and a corresponding reduction in HSE risk. This method also acts as an enabling technology for 3D seismic surveys with significantly denser spatial sampling than that usually found in Western Canada. Denser spatial sampling delivers improved data quality and more reliable rock property estimates for unconventional reservoir characterization (Ourabah, et al., 2015; Thacker, et al., 2014).
We believe that the traditional method for determining receiver locations is flawed, because it is almost always performed “backwards”. That is to say, the survey crews first deploy numbered stakes (including stakes, tags, pin-flags etc.) ahead of the recording crew and subsequently – possibly days or even weeks later – the receivers are deployed close to these stakes by a separate group of people. As an additional operational and HSE issue, people then have to be sent back to the field to clean these stakes up.
Clearly there are many potential sources of error in this process. For example, stakes can go missing between the two stages of the operation, and there is no guarantee that a survey crew will be available to re-stake the missing points. Sometimes for safety or operational reasons, the recording crew may have to deploy the equipment away from the staked location. And if an array of geophones is deployed, then the variable earth coupling of each geophone will likely be sufficient to move the effective center of gravity of the array significantly away from the staked location. In short, the often quoted decimeter accuracy of the original staked location is very rarely indicative of the actual location of the receivers, which can easily be one, two, or even more, meters away from the stake.
This inherent inaccuracy is never “seen” by the geophysicists in the data processing center, but most likely becomes incorporated into the data processing flow as a very minor perturbation to the static corrections, the stacking velocity picks or some other process. And in any case, since the target reflection Fresnel zones are several tens, or even hundreds, of meters wide, these minor positioning errors are often of little geophysical consequence.
As we seek to improve both the quality of the data and the efficiency of the field operation, we have identified a new efficiency that also enhances data quality. This comes in the form of positions that are self-derived from the nodes themselves. It turns out that when properly configured, most wireless nodes on the market today are already capable of acquiring position data. Our work demonstrates that when this data is properly acquired and processed, the resulting positions derived are more representative of geophone placement in the field than using conventional survey positioning techniques. In addition to improving positioning and reducing risk, this also facilitates the use of fully stakeless receiver deployment.
Theory and Method
GPS receivers embedded in nodes were an ingenious way for the manufacturers of those wireless systems to solve the problem of timing. Because GPS is a time-based positioning system, wireless nodal acquisition systems use this GPS timing data to correlate the receiver data with the timing of the seismic source.
While these systems use positioning data as a QC tool, some acquisition contractors have continued to rely on conventional topographic survey crews to survey receiver positions because the GPS positioning data collected by the node is not corrected via either Real Time Differential (RTD) or Real Time Kinematic (RTK) corrections and is therefore not seen to be sufficiently accurate for establishing receiver positions.
While this assumption is true of any single position calculated by the nodes, we theorized that because the nodes are collecting positioning data continuously, typically 12 to 24 hours a day for many consecutive days on end, a high quality position could in fact be derived by using all of the positioning data acquired by the node for any given receiver location. We also theorized that the final calculated positions could be made increasingly accurate by processing the numerous records based on certain positional quality indicators. By combining the derived positions with LiDAR data and other geographical and field data, a reliable and robust position within acceptable tolerances could be derived. In some wireless nodes, the positioning data are contained within ‘engineering files’ that are not normally downloaded; in other nodes these data are more readily available.
Over multiple seasons from 2011 to the present, we have acquired a large quantity of wireless nodal data from several systems, both in the field and at static locations in Calgary. We have processed the nodal positioning data using an algorithm that was devised to make the best use of the available data from each node. It was immediately obvious that the positions were very robust.
To confirm our work, in some cases where seismic surveys extended over multiple seasons we were able to ensure that many of the receiver positions were identical to positions occupied in previous seasons by using the same hole in the ground to position the 3-component receiver. We have also sent surveyors to the field equipped with RTK GPS systems (which carry a theoretical antenna horizontal accuracy of 8 mm + 1 ppm RMS and a vertical accuracy of 15 mm + 1 ppm RMS) to survey the actual locations of the receivers. This work confirmed the positional reliability of the node-derived positions and also showed that the nodal positioning was superior in determining the final geophone position compared to the commonly used method of surveying receiver positions with RTK GPS ahead of receiver deployment.
Examples
Figure 1 shows the map view of a typical point cloud from a node that was deployed in northeast British Columbia. The two symbols at the center of the cloud show the nodal position derived from an analysis of the point cloud and the actual RTK surveyed receiver point. Clearly in this case the node-derived position is well within acceptable seismic tolerances.
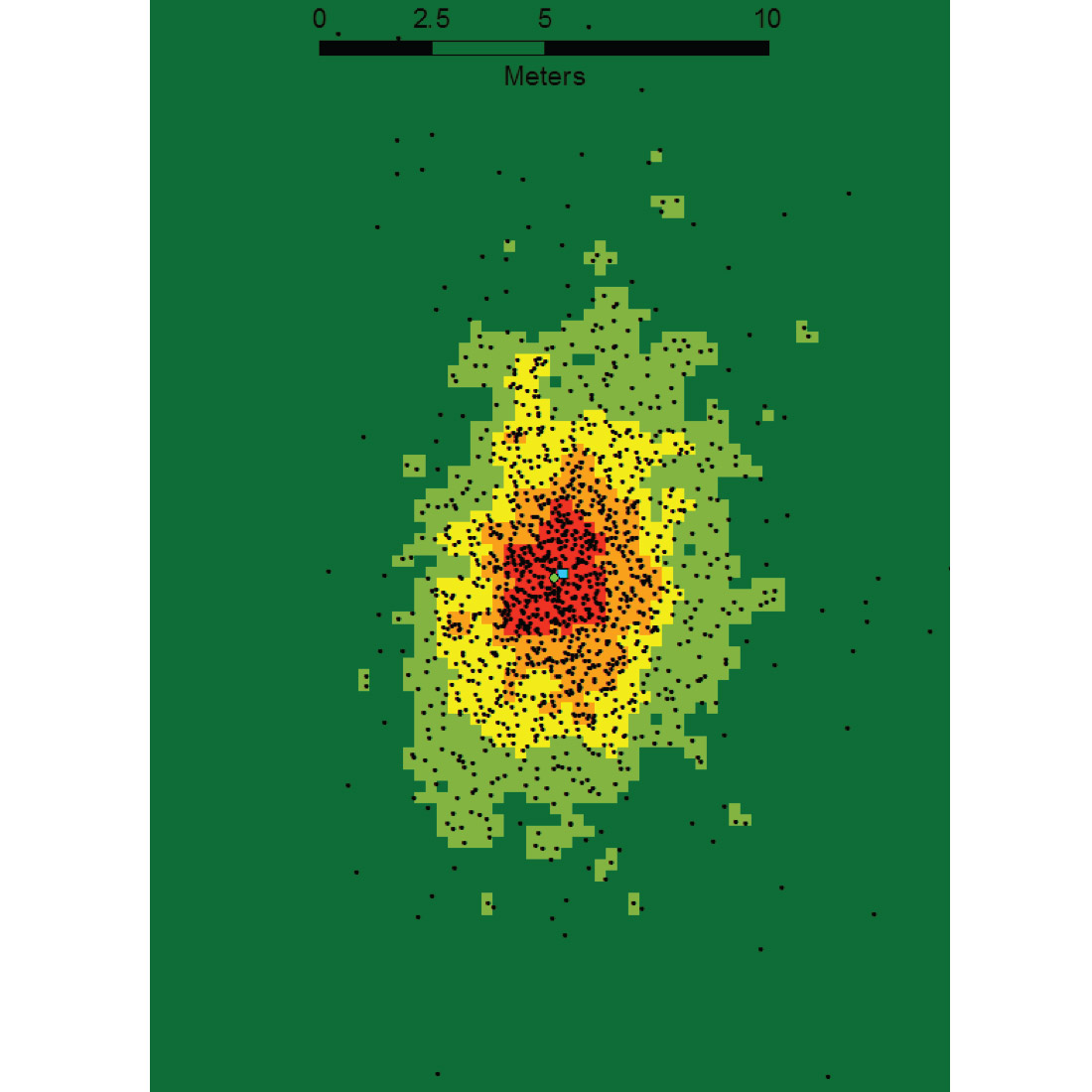
Figure 2 shows the map view of a short section of a 2D seismic line with the several node point clouds shown in plan view. The blue squares show the derived nodal positions, and the green triangles show the RTK surveyed locations of the single three-component receivers. In this example, we believe that the cable linking the receiver to the node is the most likely source of the minor positional differences between the two points.
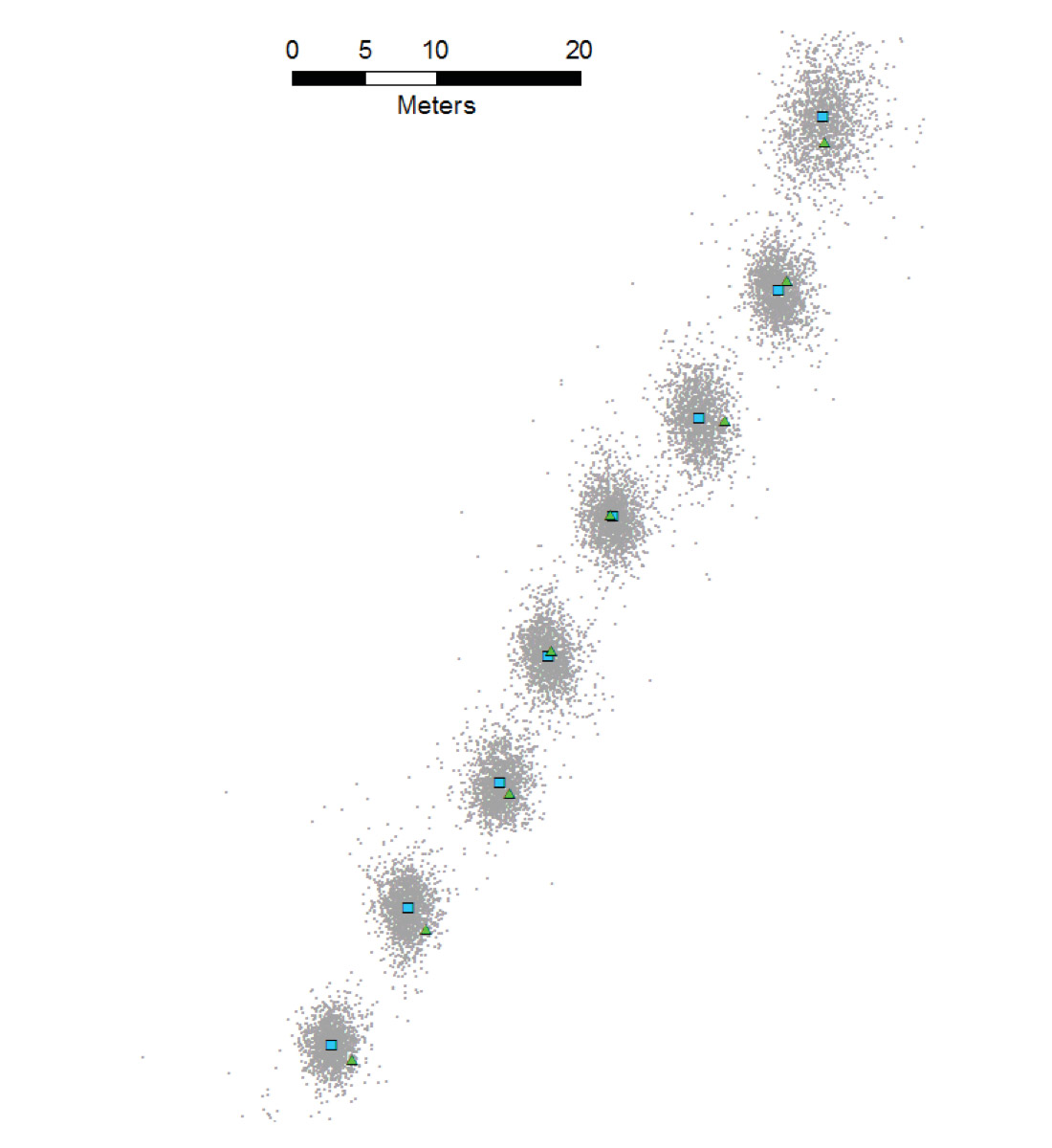
Figures 3 and 4 show the relationship between a bare earth LiDAR surface and the node point clouds. By integrating these two data types it is possible to derive accurate x, y and z values for each receiver location.
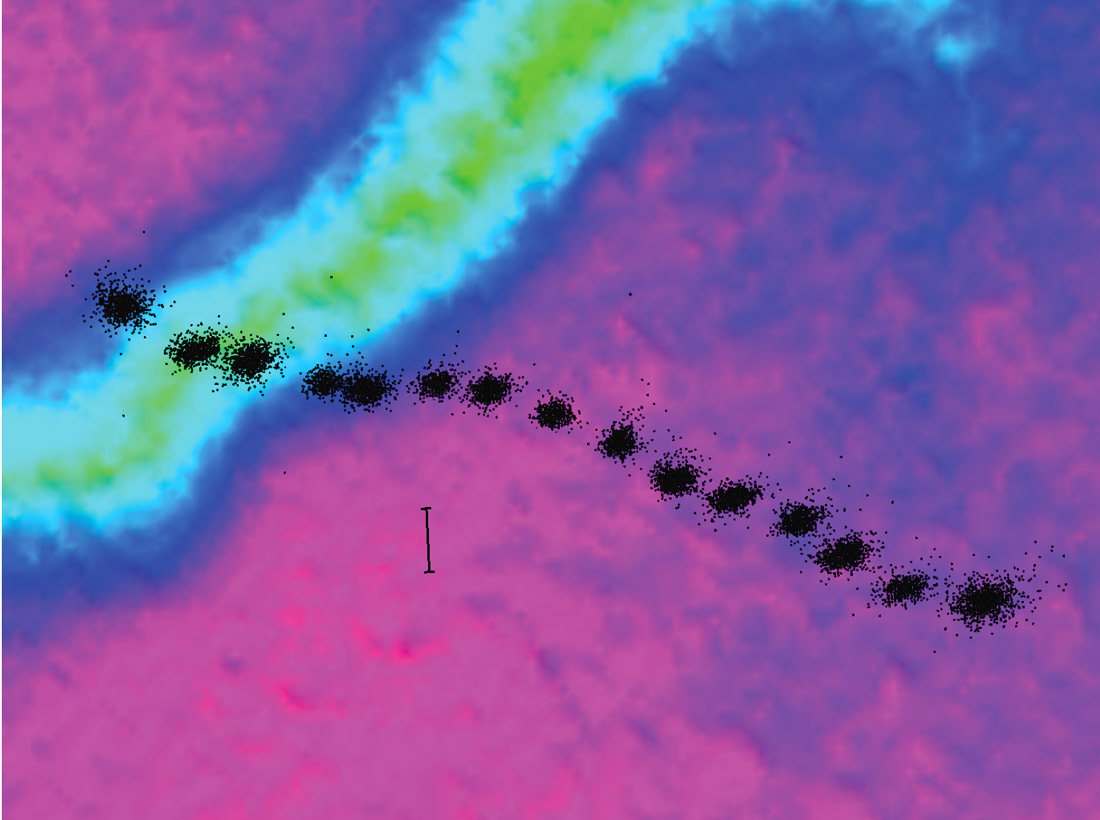
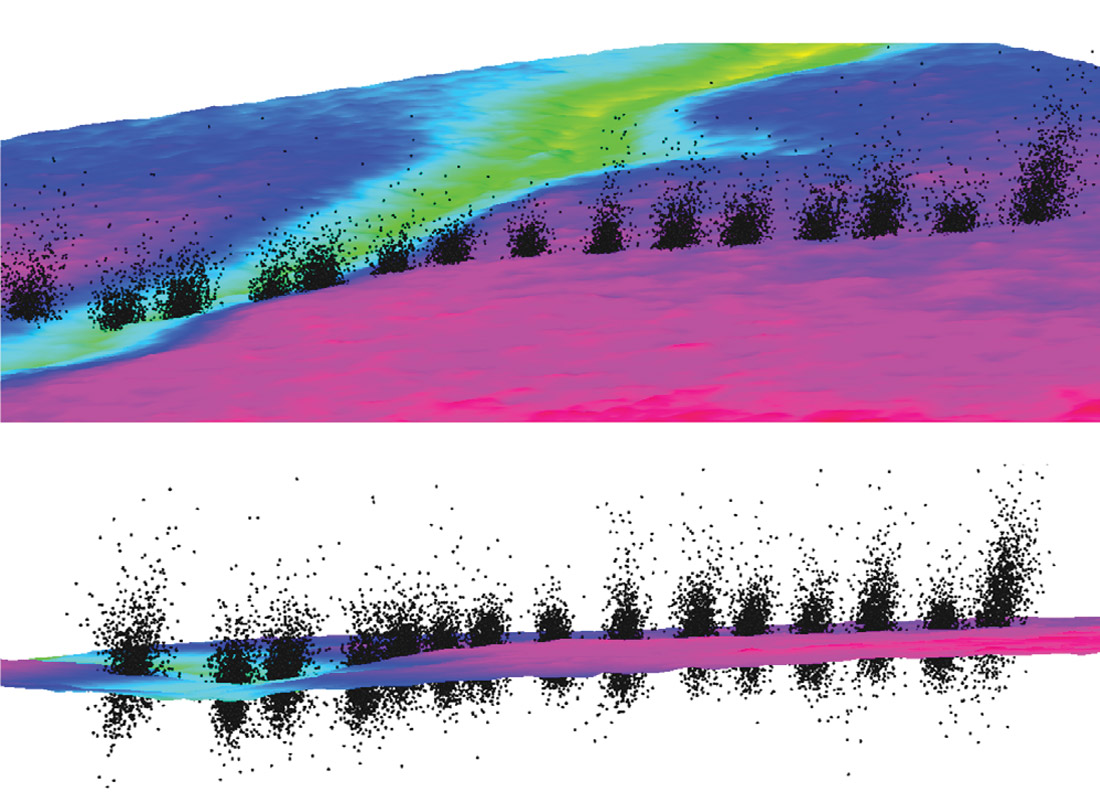
Figure 5 shows the statistical analysis for a conventional receiver deployment technique (ie RTK surveying of station stakes prior to the deployment of the receiver equipment) where the true locations of the receiver holes were re-surveyed by RTK after the receivers had been retrieved. The RTK re-survey showed mean differences of about 2.6 meters between the pre-deployment staked positions and the final, actual, receiver locations. This agrees with our statement above that “the quoted decimeter accuracy of the original staked location is very rarely indicative of the actual location of the receivers, which can easily be one, two, or even more, meters away from the stake.”
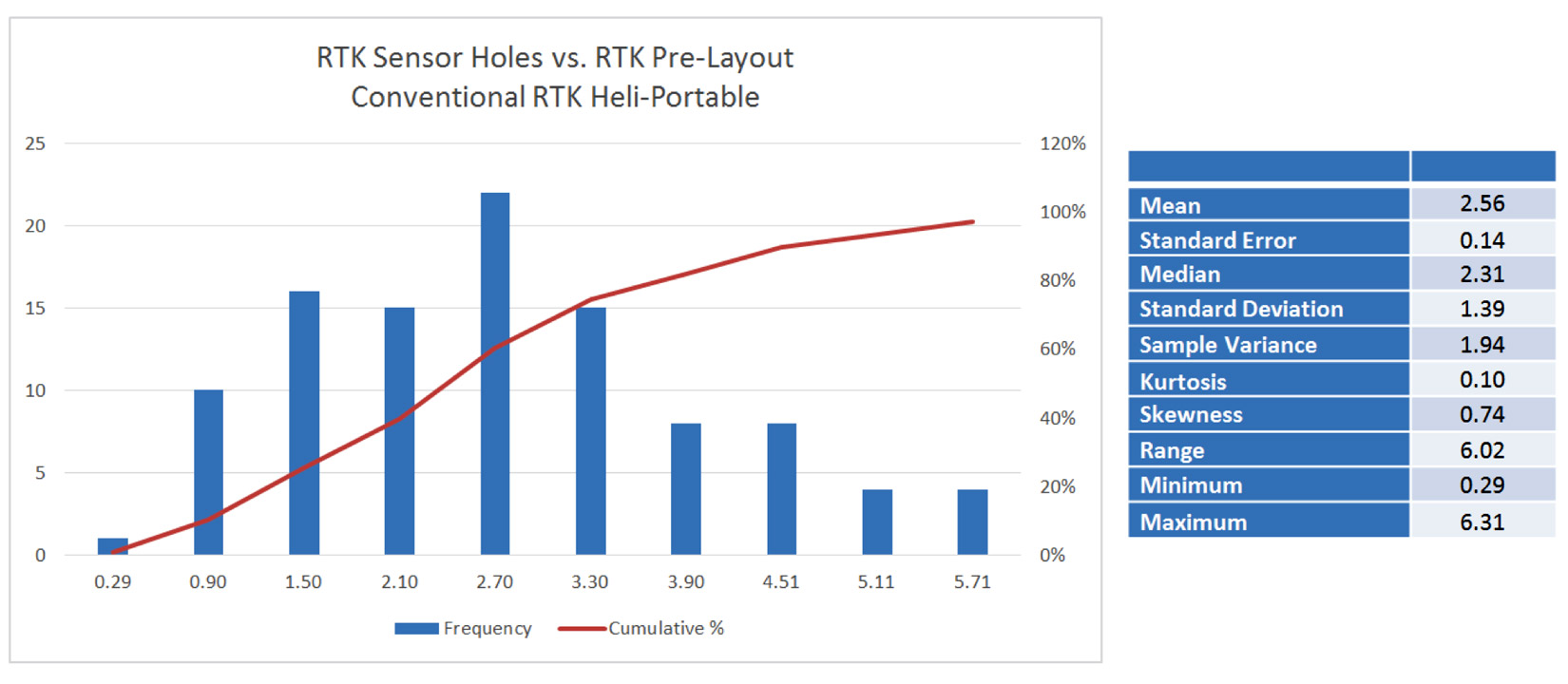
Figure 6 shows a similar statistical analysisof nodal-derived positions compared to the RTK survey positions of the same receivers. These data show a mean positioning difference of about 1.6 meters, with over 90% of the positioning differences being less than 3 meters.
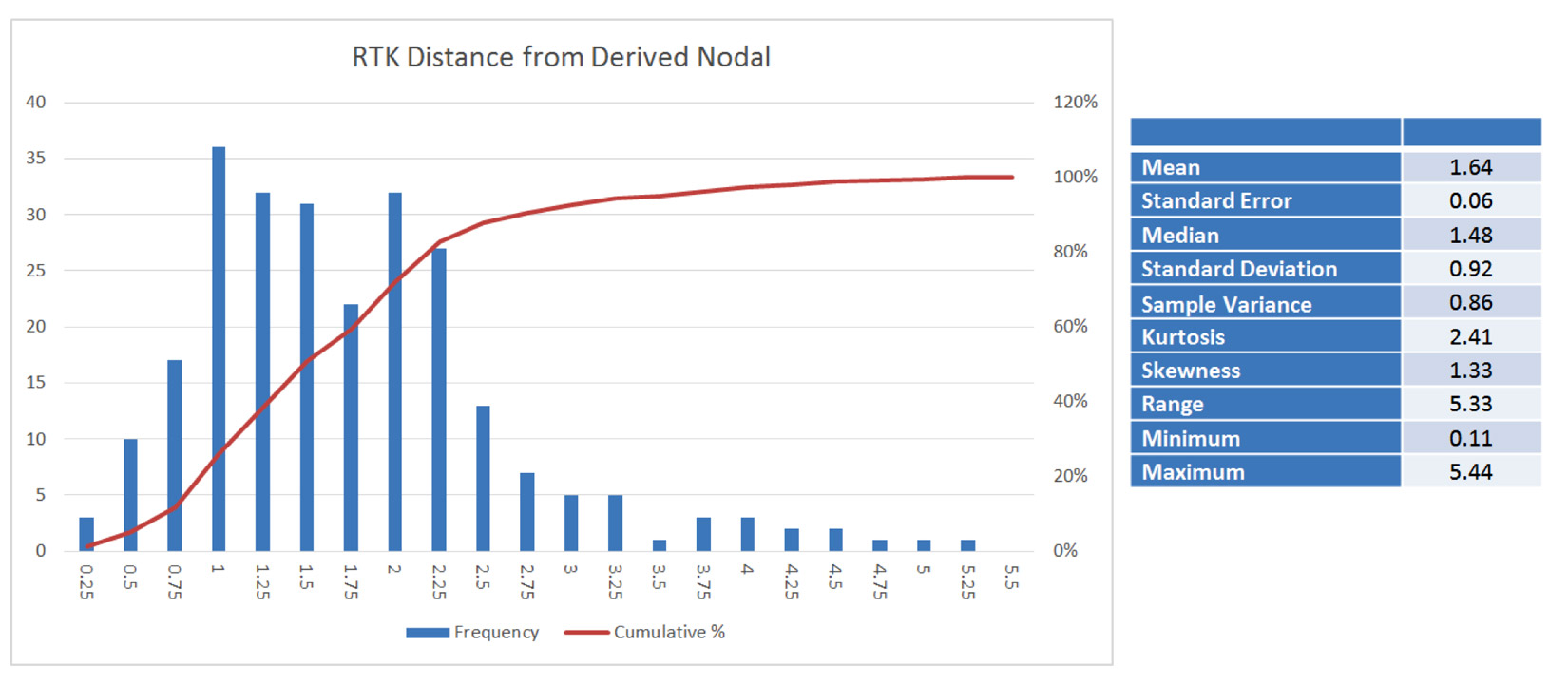
Conclusions
The currently employed practice of surveying receiver positions ahead of layout is rendered obsolete with the advent of wireless seismic receiver nodes with embedded GPS receivers. The quality of the horizontal positioning data for seismic receivers is improved with this method. By combining these horizontal positions with high resolution LiDAR data for vertical positioning, the quality of the receiver positioning solution is improved. While the importance of this qualitative improvement in receiver position relative to the resulting seismic image will be a function of seismic acquisition parameters and the required seismic resolution to image the target objective, the efficiency gains and risk reduction that flow from modifying field operations to incorporate this method offer that optimal result of improved quality and improved efficiency.
We are optimistic that manufacturers of nodal systems will consider this enhanced capability of their systems as well as inchoate opportunities for even more robust positioning features in future releases of data harvesting software and in future generations of land-based seismic receiver nodes.
Acknowledgements
Explor would like to acknowledge the co-operation and assistance of Site Energy Services, CGG and SAE over the several seasons of work that it has taken to acquire these data.
Join the Conversation
Interested in starting, or contributing to a conversation about an article or issue of the RECORDER? Join our CSEG LinkedIn Group.
Share This Article