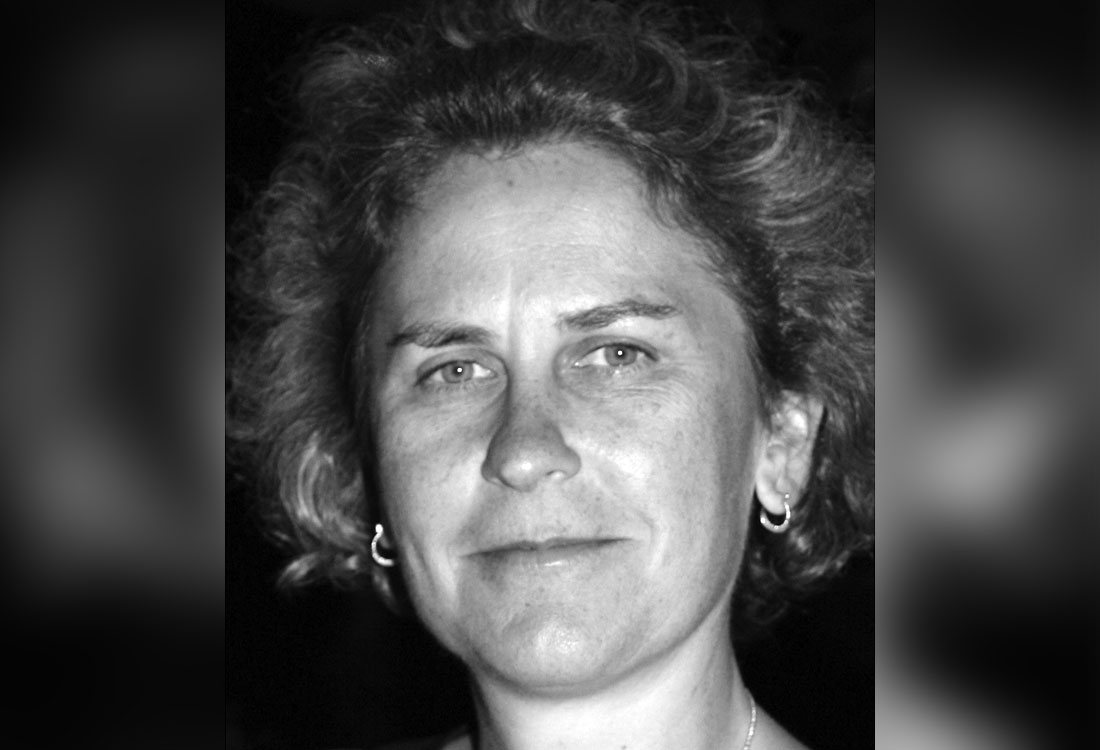
Mihaela S. Ryer has over 20 years of experience in the oil and gas industry. During her career she held numerous technical, research and leadership positions in exploration, production and applied technology organizations. She worked for Hess Corporation, ConocoPhillips, Marathon Oil Company, the ARIES Group., Inc., and Prospectiuni SA. She specializes in clastic depositional systems analysis from pore to basin scale, seismic sequence stratigraphy, integrated reservoir prediction, and worked in many sedimentary basins in the US and internationally. Mihaela received a Diploma in Geology from University of Bucharest-Romania, and a PhD in Geology from University of Colorado-Boulder.
Seismic stratigraphy is an approach that allows the geoscientist interpreter to extract stratigraphic and depositional environment information and insight from seismic data. One of the critical steps in any rigorous seismic stratigraphic workflow is the definition of key surfaces, which form the basis and the framework for any further qualitative or quantitative analysis.
Key surfaces are seismic reflectors of regional extent that can be defined and mapped on the basis of seismic reflection terminations, internal reflection configuration, and rigorous integration with any available well data. Five principal types of reflection terminations are associated with key seismic stratigraphic surfaces: onlap, downlap, toplap, truncation, and concordant. The internal reflection configuration is the seismic proxy for sedimentary stratal patterns; recording and documenting it gives valuable insight into depositional processes and products. Commonly, six types of reflection configurations are used for seismic key surface definition: parallel, subparallel, divergent, prograding, chaotic, and reflection-free.
Types of seismic key surfaces
Seismic stratigraphic key surfaces are of two types: sequence boundary and maximum flooding surface (MFS); several criteria are used to properly define them.
Sequence boundary – probably the most abused key seismic surface. Inexperienced seismic stratigraphic interpreters may call any strong and/or continuous reflector a sequence boundary. A correct definition is not only an academic issue; it has significant implications for depositional history and stratigraphic and depositional environment predictions. We use the following criteria to define a sequence boundary:
- 1. onlap of coastal deposits onto the boundary;
- erosion and incision on the shelf;
- toplap or apparent toplap below the surface;
- a major basinward shift in sedimentary facies across it.
Genetically, a sequence boundary is the result of an abrupt relative sea-level lowering, resulting in subaerial exposure, erosion, and fluvial incision of the and shelf. During this time, sediment generally bypasses the shelf area and is deposited on the slope or basin floor via gravity-flow processes.
Maximum flooding surface – a continuous draping event resulting from regionally extensive and significant water deepening. It is commonly recognized on the seismic because of downlap reflection terminations onto the surface. Maximum flooding surfaces are commonly overlain by a regionally extensive marine mudstone or shale and, as a result, abrupt changes in incremental overpressure are present across them. Genetically, maximum flooding is the result of a rapid relative sea-level rise resulting in the shoreline migrating to its maximum landward position.
Maximum flooding surfaces and sequence boundaries are the key bounding surfaces in a depositional sequence. They are used to define the systems tracts which, together with the depositional environment and sedimentary facies, are used to interpret the sedimentary evolution of a basin or area. If the sequence stratigraphic analysis is used in the context of a petroleum system evaluation, the proper key surface definition and interpretation plays a major role in the prediction of reservoir, seal, and source rock.
For lots more on seismic stratigraphic key surfaces, or seismic sequence stratigraphy in general, visit the SEPM Sequence Stratigraphy website at sepmstrata.org.
Q&A:
You have worked in positions where you have lead, managed and contributed to integrated teams of geoscientists and engineers. In fact, you specialize in leading integrated teams. Please tell us how you contributed, how these integrated teams functioned, and what kind of results were achieved?
An integrated team is like a puzzle: each piece of the puzzle has to fit all the others perfectly for the puzzle to make sense. A fully functional integrated team in my line of business – oil and gas industry – consists of professionals who contribute their expertise and experience to solve very complex problems, which could not be solved by an individual alone. Throughout my career I always worked in teams: at the beginning as a technical contributor, later on as a team leader or manager. I am a very hands-on professional, and even when I lead a team, I have some technical contributor role in the project. I always wanted to keep close to the technical side, it fits who I am.
I am a stratigrapher-sedimentologist by background, and the best teams I was part of were multidisciplinary: geologists, geophysicists, petrophysicists, engineers, land professionals, etc. When you are part of the team you have to know precisely how your expertise will help solve the problem at hand, but also you have to have a very good understanding of how your work blends with the other disciplines that are part of the project. If you want to contribute to your full potential, you have to stay on top of your own field, but also you have to learn from all the other disciplines.
In your expert opinion, do you think such integrated teams really contribute in a beneficial way? Please elaborate with examples.
When working in the oil and gas industry the problems one must solve every day are very complex. We are in the business of economically finding and producing hydrocarbons to fuel the world’s economy; the reality is that all the easy to find and produce stuff was found and produced long time ago. Our targets are at greater depths, in deeper water, in areas with very complicated subsurface geology, in places with as much ‘above ground’ risk as ‘below ground’. The complexity of our everyday work can only be solved by integrated and multidisciplinary teams anchored in fundamental knowledge of geoscience and engineering, enhanced by the most advanced technological breakthroughs, supported by numerous other experts – economists, EHS, legal to name only a few. Let’s take the example of reservoir presence and quality prediction. Sedimentology and stratigraphy plays a big role in it, but to really address the problem there must be a thorough understanding of the structural evolution at regional and local scale, of rock-physics to provide the link between the rocks and the seismic, of petrography and diagenesis, of the generation and migration of hydrocarbons, of the fluid types and how they interact with the rock frame, and the list could go on. I have been in the industry for over twenty years and did not meet one individual who is an expert in all of these subjects, hence the benefit of integrated and multidisciplinary teams.
Talking about reservoir analysis, which is a broad term, tell us what this term means to you and how you perform such tasks?
It is true, reservoir analysis is a broad term and many definitions exits for what it is, depending on who you talk to. For me reservoir analysis is a comprehensive study of the hydrocarbon reservoir system at all scales, from a static as well as dynamic point of view, performed with the goal of fully understanding the risks and uncertainties associated with such a system, and to ultimately make predictions to drive business decisions. Let me brake down this somewhat long definition. By reservoir system I mean the rock-pore-fluid system and their interaction in time and space. This complex system can only be understood if approached from multiple scales: the grain and pore scale, the flow unit scale, the field scale, the basin scale. This multi-scale approach must be maintained throughout the life span of a reservoir. A reservoir can be viewed from a static point of view (think geomodels), which is a necessary simplification we embark on at a certain point in our analysis. But more importantly a reservoir is a dynamic system, both at the geologic time scale (migration and entrapment of hydrocarbons) as well as at production timescale (primary or enhanced hydrocarbon recovery). Last but not least, in my line of work reservoir analysis is performed to drive business decisions and positively impact the bottom line. This requires a razor-sharp focus on what is important, embarking in fit-for-purpose work and innovation.
Reservoir analysis relies on the integration of multidisciplinary datasets, tools and measurements. Therefore core, wireline logs, seismic, pressure, geochemistry datasets, to name only a few, must be acquired, integrated and used in the analysis.
You have had the experience in leading applied research projects. What are some of the novel ideas that you may have worked on?
I can talk for a long time about this, as it is one of the subjects I am very passionate about, but for here and now I will narrow the list to two examples: stratigraphic forward modeling and quantitative reservoir prediction.
In my opinion process-based stratigraphic forward modeling is a field with a huge growth space and significant upside potential. For reasons I still don’t understand, forward models are lagging in stratigraphy, although they are accepted and became the norm in most other geoscience and engineering disciplines. Stratigraphers love doodles, but have difficulty turning them into digits, and are suspicious of anyone who tells them that something like this is possible. My view is that we are still close to stone age in the way we populate the three-dimensional Earth models with facies and reservoir properties. There is encouraging progress done in the last five years in this space (e.g. Multiple Point Statistics used in geomodeling), but we are still some distance away from building data-constrained, process-based stratigraphic models that can be linked to basin models, geomodels, structural models etc.
Quantitative reservoir prediction has to do with the integration of petroleum systems, reservoir quality and structural quantitative models with the goal of predicting reservoir performance at all scales. This is particularly important in complex plays (like most plays are now days) where competitive advantage may be gained by finding those ‘sweet spots’, which are your competitor’s ‘blind spots’. I mentioned above the fact that I view the reservoir as a system of pore-rock particles- fluid. Quantitative reservoir prediction as defined above can identify opportunities in a play where for example reservoir quality is not great, but is compensated by very good fluid quality, and hence the reservoir deliverability may be similar to an opportunity where both rock and fluid properties are average. The Tertiary play in the deep-water Gulf of Mexico, or most of unconventional plays are good examples where this approach should be strongly considered.
The three well-known principles of stratigraphy are superposition, continuity and original horizontality. You mention a very important starting point about picking the key reflections on the seismic data. In the light of this, could you briefly describe how interpreters perform stratigraphic interpretation on seismic data?
Seismic stratigraphy is a very powerful technique, which must be employed at all stages of the business cycle – exploration – appraisal – development – production – if the seismic data is of decent quality.
The first and most important prerequisite is to have the seismic data processed in a ‘stratigraphic-friendly’ way, for example amplitude preservation is a must. Another example – I have seen datasets where clinoforms were treated as multiples or noise and eliminated during the processing. In order to have the best seismic volume for seismic stratigraphic interpretation, the stratigrapher, the geophysicist and the seismic processor must work very close together throughout the duration of the project, not only during processing. This is not always the case.
The second important point is data integration. Well data (core, biostratigraphy, logs), rock physics and elastic data, analog data (outcrops, nearby fields, basins).
The third important aspect of seismic stratigraphic interpretation is what makes it somewhat different from the standard seismic interpretation, in which understanding the structure is the main goal. Seismic stratigraphy places emphasis on reflection terminations, reflection configuration and geometry of geologic bodies above all else. The seismic stratigrapher uses these elements to map the seismic and not necessarily the most continuous or the brightest reflector. This is why it is important for a seismic stratigrapher to be very strongly anchored in the fundamental of the seismic method, in the fundamentals of depositional systems, and to have significant experience in looking at rocks, so working with core and outcrops is a must.
In many areas when a hard formation overlies another not-so-hard target formation, the frequency content below is reduced. What can possibly be done to aid the stratigraphic interpretation in the target interval?
Assuming that everything was done geophysically (from the processing point of view), in my experience there are three other approaches that should be considered. First is becoming intimate with the data and paying attention to detail. In my experience, there are always ‘clues’ in the data that once observed or discovered they open up avenues for interpretation not thought of before. Secondly, detailed mapping combined with fit-for-purpose attribute analysis. This is time consuming and can be frustrating, especially when frequency content is reduced, but is an approach that pays off if done systematically. Third approach is the use of analogs, and I emphasize the plural. There is a tendency to latch on a single, favorite analog, and when the data is less than perfect this is a dangerous approach. Using outcrop analogs of similar depositional systems and seismic analogs of areas better imaged will help with the interpretation of low frequency seismic interval.
The other example is when coals overlay sandstone formations. The confounding effects of coals are well known. What according to you is the best way of handing the interpretation in such cases?
I am assuming the question refers to seismic interpretation. In this case I have three suggestions: 1) use (or become) an expert in coal geology. Coals form in very specific geological conditions, and understanding these conditions and the resulting lateral and vertical relationships will add tremendously in how one interprets seismic data. 2) beware of compaction rates: there are huge differences in the way coals compact compared to all other lithologies. When compaction is taken into consideration some interpretations become hard to defend; and 3) use outcrop analogs of coal-bearing settings, couple this with seismic forward modeling and compare the results with the real seismic data.
In my experience, significant insights and interpretation breakthroughs will emerge. The North Malay Basin is a very interesting (and challenging) case study where these approaches paid off.
A significant challenge in stratigraphic interpretation is the mapping of thin deep-water turbidite sands as their thickness and lateral character variation is difficult to perceive, due to the limited seismic resolution. One straight-forward way out would be enhancing resolution of the data in an amplitude-friendly way. The other option could be using spectral decomposition, but that restricts examining the data between the bounds of the existing bandwidth. How would you approach such a problem? If frequency enhancement has to be adopted, what method would you recommend?
It is difficult to answer this, as the approach may differ depending on the specific geologic setting. Having said that I would offer two suggestions: seismic inversion, including elastic inversion, and 4-D seismic.
On the lighter side Mihaela, it is usually said that people who fall in love with geoscience, stay at it and persistently do good work. How would you react to it? Is it true in your case?
I wanted to be a geoscientist ever since I was sixteen. My uncle, who did cave exploration in his spare time, took me with him to explore caves in the Southern Carpathians of Romania. As I was negotiating a shaft in the cave, I turned off my little head lamp for a moment, and not only experienced complete darkness for the first time in my life, but there and then experienced the mystery of the Earth – and I was hooked. For me geoscience is a lifestyle, not only a profession. I am one of those people who come back from vacation with more pictures of rocks than of people, or the pictures of people must have rocks in them to be worthwhile. I am one of those people who, when on the beach, is more interested in ripple and dune formation, or in wavesediment interaction than anything else. And yes, my napkins often have drawings of cross-sections if the dinner companions have similar interests. So yes, it is true in my case.
Share This Interview