Compared to our other sensory organs, the human ear has always struck me as odd. We are delivered touch, smell, sight, and taste in such a seamless, integrated way that it’s almost magical. In comparison, the ear seems to be a home handyman job! I can relate to that, and the ear’s weird looking parts have a curious mechanical appeal to me. In this article I’ll go over the basic parts of the human ear and how they all work together.
Basic structure
An interesting aspect of the human ear (and indeed all mammalian ears) is that each of its three main components – the outer, middle, and inner ear – is formed from a different germ layer at the embryonic stage. Following fertilization, zygotes form blastulae, which are hollow proto-cells of a sort (MacCord, 2018). These undergo a process called gastrulation, whereby three distinct layers form within the blastulae – the endoderm in the centre, the ectoderm on the exterior, and the mesoderm in between. Some creatures do not have the mesoderm layer within their cells (diploblastic), and these tend to possess radial symmetry, such as jellyfish and sea anemones. We humans are triploblastic, and all parts of our bodies develop from these three germ layers.
The inner ear is the first to develop, from the ectoderm, beginning around the 22nd day after fertilization. The middle and outer ear follow, from the endoderm and mesoderm, respectively. The embryonic steps involved in the development of the ear are particularly complicated compared to most other parts of the body, and therefore developmental problems with the auditory system are not uncommon.
Figure 1 shows the basic components of the ear. The outer ear can be considered the receiver, the middle ear the amplifier, and the inner ear the converter (from sound waves to electrical signals sent to the brain). The following sections cover these three main parts of the ear – their components and how they work.
Outer ear
In addition to the visible parts of the ear, known as the auricle or pinna, the outer ear also encompasses the ear canal which extends through an opening in the skull, and ends at the ear drum, technically known as the tympanic membrane. All the external parts of the ear have been given Latin names. There is the helix which is the outer rim, the inner rim called the anti-helix, the lobe, and the tragus which is the little flap forward of the opening to the external auditory canal, which is called the aperture. The pinna is made of skin overlying cartilage, while the external auditory canal (or meatus) is encased within bone. The lining of the ear canal contains sebaceous and ceruminous glands which secrete oils and waxes (cerumen), respectively.
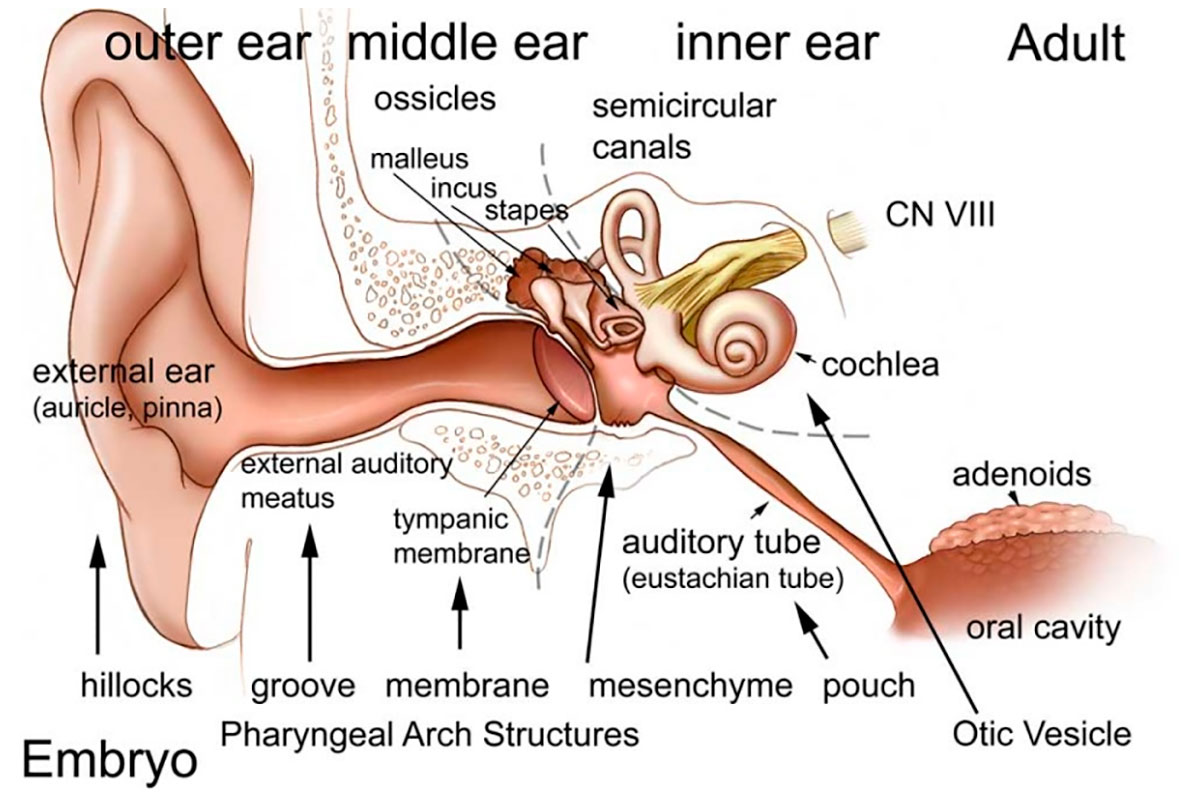
All the curious folds and creases of the pinna serve some signal processing purposes. Most fundamentally, the pinna collects sound waves over a larger area and funnels them into the ear canal, thus amplifying frequencies in the range of 1.5 to 7 kHhz by 10-15 dB, not surprisingly the frequency range of human speech. At lower frequencies the pinna mainly acts as an amplifier. At higher frequencies however, in addition to amplification, it also does some very interesting notch filtering. These higher frequency signals coming from above (as opposed to from the front) reflect off certain pinna surfaces, and bounce back off the helix, and therefore enter the canal after a slight delay. Depending on the direction of the sound and the unique structure of each person’s ear geometry, destructive interference creates a frequency notch anywhere between 6 and 16 kHz, but close to 10 kHz for most people. The relative presence or absence of this notch appears to aid in sound location. In addition, the pinna’s amplification has a directional sweet spot, which also contributes to our ability to detect where sounds are coming from; we slightly move our heads until sound is maximized. The comparison of the signals received from each of the two ears obviously is also part of our sound locating abilities. And, of course, the ear acts as somewhat of a physical barrier to protect the more delicate internal parts, Q-Tips notwithstanding!
The outer ear displays a few interesting vestigial or atavistic features. If you run your finger along the downward facing edge of your helix at the top of your ear, from front to back, you may feel a small bump. This is called Darwin’s tubercle. In some other mammals this develops more fully, resulting in pointed ears, for example foxes. Many mammalian species that rely on sound for survival have muscles that can move the ears, in some species over a quite considerable range. This allows them to maximize the sound locating abilities that we possess in a limited form, as described in the previous paragraph. Some people possess this ability to a certain extent. My paternal grandmother could wiggle both ears, independently even, and both my kids can wiggle theirs, although not one at a time; neither my wife nor I has even a remote ability to do this, so I assume this is controlled by a recessive gene. Proponents of the aquatic ape hypothesis, which holds that a semi-aquatic littoral stage early in human evolution helped shape us as a species, point to the tragus as a vestigial feature. I think the idea is that the tragus in most aquatic mammals develops fully into a valve that prevents water from entering the ear canal while the animal is diving or otherwise underwater. However, I don’t believe that to be the case; I think those valves develop some other way. The tragus is known to play an important echolocation role in bat ears, though.
The eardrum, or tympanic membrane, is found at the end of the auditory canal (meatus). The sound waves received and amplified by the pinna travel down the canal and vibrate the ear drum, which activates the components on the other side, within the middle ear.
Middle ear
The middle ear contains some very interesting mechanical parts (Figure 2): the malleus, the incus and the stapes, collectively referred to as the ossicular chain. The Latin names of these three small bones translate to hammer, anvil and stirrup in English, and refer to their distinctive shapes. They are the smallest bones in our bodies, with the malleus having an average length of 8 mm for an adult human. The malleus is connected to the inside of the eardrum at one end, and to the incus at the other. The incus is connected to the stapes, which is attached to its footplate, which is in turn attached to the oval window.
These three bones transmit the sound waves from the outer ear into the inner ear. It seems like a redundant step, but it makes sense once explained. The ear canal of the outer ear is obviously air-filled, as is the chamber of the middle ear, known as the tympanic cavity. However, the inner ear on the other side of the oval window contains a fluid, perilymph. As we know, the boundary between air and fluid will have a high acoustic impedance, meaning most sound wave energy is reflected. The ossicular chain overcomes this and allows the sound waves to be mechanically transmitted from the air-filled ear canal to the fluid-filled cochlea, and amplifies the signals in the process.
At ~55 mm2 the area of the ear drum is over 17 times greater than that of the stapes footplate at ~3.2 mm2, thus providing a multiplier effect to the force exerted on the latter. The ossicular chain also acts as a lever, providing a frequency-dependent lever arm factor of between 1.3 and 5. The malleus is the long arm, the stapes is the short arm, and the incus is the fulcrum. The peak factor of 5 is reached at around 2 kHz, which is right at the heart of the frequency range of human speech. I assume that the variable lever effect is achieved by the way the malleus is attached across a broad area of the eardrum. I’m fascinated that there are mechanical tricks at play inside our ear, similar to those that you might see in a motor, such as variable valve timing in a high-performance combustion engine.
The amplification of force is further modified by two other mechanisms. At higher input frequencies, above 3 kHz, the eardrum can vibrate in a disorganized, non-linear fashion; the ~0.5 cm wide attachment of the malleus to the eardrum acts as a mechanical smoothing filter, translating a chaotic signal into a linear one. There are also tiny muscles attached to the ossicular bones which reflexively contract when really high amplitude signals are received, which causes the connections between the bones to partially detach, providing a dampening effect. Interesting fact: the stapedius muscle, which stabilizes the stapes, is the smallest muscle found in the human body, with a length of just over 1 mm. Viewed as an integrated system, the transfer function of the outer and middle ears has a peak efficiency somewhere between 1 and 3 kHz.
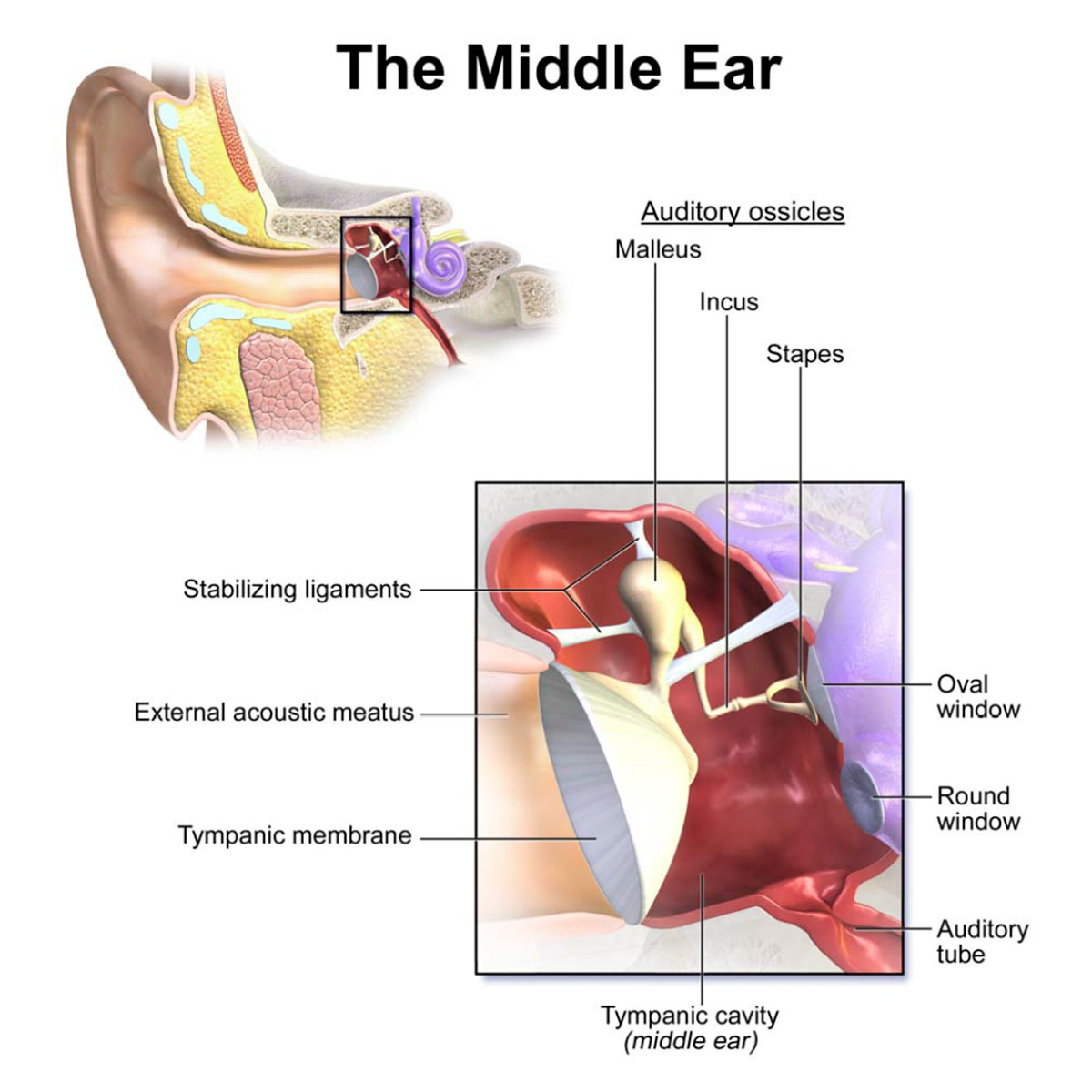
Two other components of the middle ear should be mentioned. First, the round window (or secondary tympanic membrane) is seen below the oval window in Figure 2. The fluid inside the inner ear is incompressible, so when the oval window is pushed into the fluid, the round window pops out, allowing the system to work. Second, note the auditory tube depicted in the lower right of Figure 2. These are more commonly known as the Eustachian tubes (plural, because there are two, one for each ear). Their purpose is to equalize differences in pressure between the outside world and inside the tympanic cavity, and they also allow any fluid buildup inside the middle ear to drain out. In Figure 2 a tube is shown in its resting, closed state. When subjected to pressure it opens up, and the pressure difference is eliminated. The other end opens to the back of the mouth, more or less at the same level as the nostrils, above the large opening to the pharynx. I’ve looked in the mirror at my mouth and I think I can see the openings (a flashlight helps), but I’m not 100% sure. Allergies or sinus infections can cause a mucus buildup inside the Eustachian tubes, and the resulting full or partial blockage can cause pain inside the middle ear due to pressure differences.
There is another component of our hearing that bypasses the outer and middle ear – sounds heard via bone conduction. The skull vibrates when sound waves hit it, and these vibrations are imparted to the fluid in the cochlea, therefore we hear the same signals via two different paths. Because the skull bones transmit lower frequencies better than higher frequencies, the signals received via bone conduction tend to be biased towards lower frequencies; this is why our own voice when we speak tends to sound deeper to us than when we hear recordings of it. Beethoven, after he went deaf, famously used bone conduction to continue to hear music, by clenching a metal rod between his teeth and touching it to his piano as he composed and played. Some modern technologies exploit the same thing, such as Google Glass, some hearing aids, and devices used by scuba divers to hear sounds in the water around them.
To summarize, when a sound wave vibrates the eardrum, this engages the ossicular chain, which vibrates the oval window. This system performs impedance matching – the signal imparted to the oval window is increased in force but reduced in velocity and displacement, resulting in tiny pressure waves being generated from the other side of the oval window, which then emanate out into the fluid contained within the cochlea.
Inner ear
The inner ear performs two critical functions, one obviously related to hearing, the other to our sense of balance. Both of these functions are covered in the sections below.
The inner ear holds two labyrinths, one inside the other. The bony labyrinth is made up of three main sections, basically voids that make up a miniature cave system within the skull bone next to our ears: the vestibule is immediately adjacent to the oval and round windows; to the front of it are the spirals of the cochlea; to the rear are the three semicircular canals (anterior, lateral and posterior). Held within these three main sections of the bony labyrinth are the corresponding components that make up the membranous labyrinth. All this is shown nicely in Figure 3.
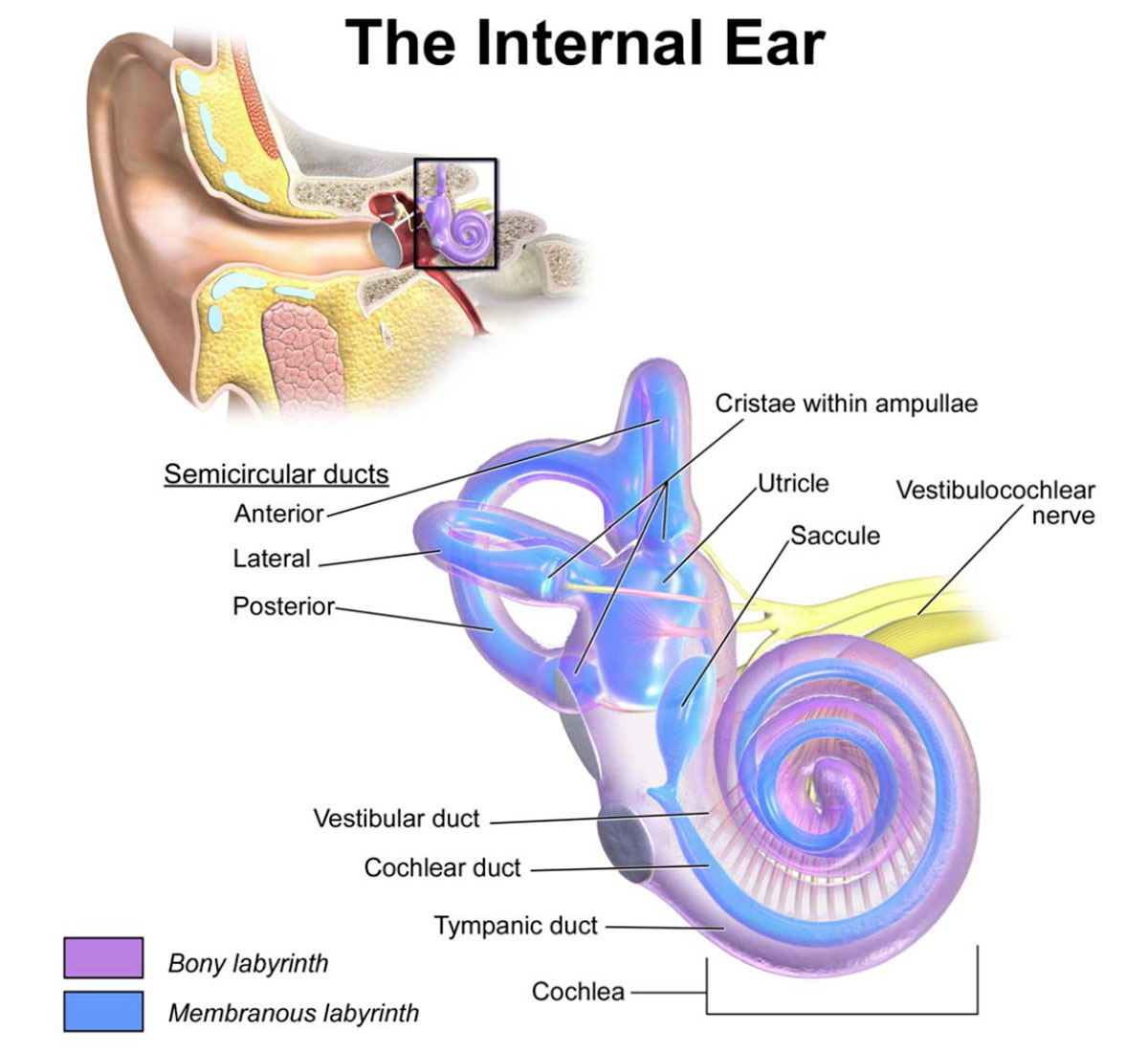
The bony labyrinth, depicted in purple, is filled with high sodium, low potassium perilymph fluid and the membranous labyrinth. The membranous labyrinth is depicted in blue and is filled with a liquid called endolymph (high potassium, low sodium); its components are the three semicircular ducts each in its semicircular canal, the saccule and utricle inside the vestibule, and the cochlear duct inside the cochlea. The cochlea provides the auditory function, the vestibular system static balance, and the semicircular canal system dynamic balance. These three functions all utilize cells that contain tiny little hair-like fibres that are attached to nerves. When deflected they generate or alter electrical signals that are sent to the brain. The brain processes these electrical signals and translates them into the neurological experiences we know as sound and balance.
Hearing
The conical, spiral structure of the cochlea resembles a snail shell, hence its name – cochlea is Greek for snail (Figure 4, upper left). The 30 mm long canal makes 2¾ turns around the central axis, known as the modiolus, which contains the cochlear nerve plus other connections to the body such as blood vessels. A bony ridge spirals up inside the main “pipe”, and in addition to providing the entry point for the nerves (spinal ganglion) and other connections, it also acts as the anchor for the membranous duct. The main part of Figure 4 shows a cross section of the cochlea. The membranous cochlear duct divides the “pipe” into three sections, with the scala vestibuli above, and the scala tympani below. The scala vestibuli starts at the oval window, and the scala tympani ends at the round window; the two are connected at the pointy apex of the cochlea (known as the helicotrema).
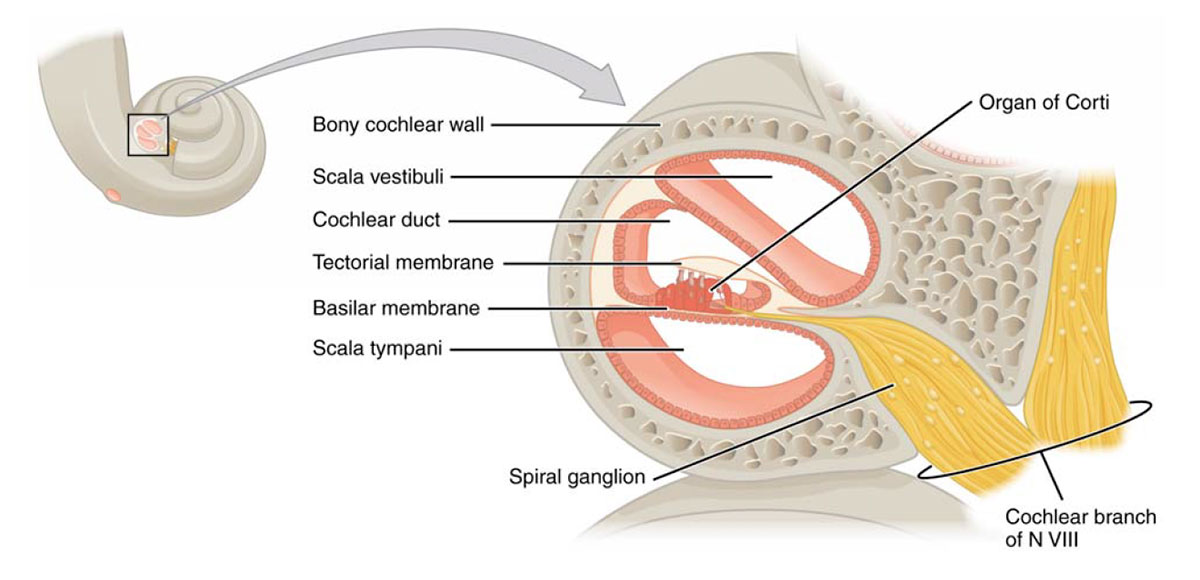
When the oval window is vibrated by the stapes, pressure waves travel up through the scala vestibuli to the helicotrema, then back down the scala tympani to the round window. As the waves are on the upward trip through the scala vestibuli, they ripple through the upper membranous surface (Reissner’s membrane), and into the endolymph fluid of the cochlear duct. It is biomechanical circuitry within the membranous cochlear duct, specifically in the organ of Corti (Figure 5), that converts the physical waves into electrical signals that are sent to the brain.
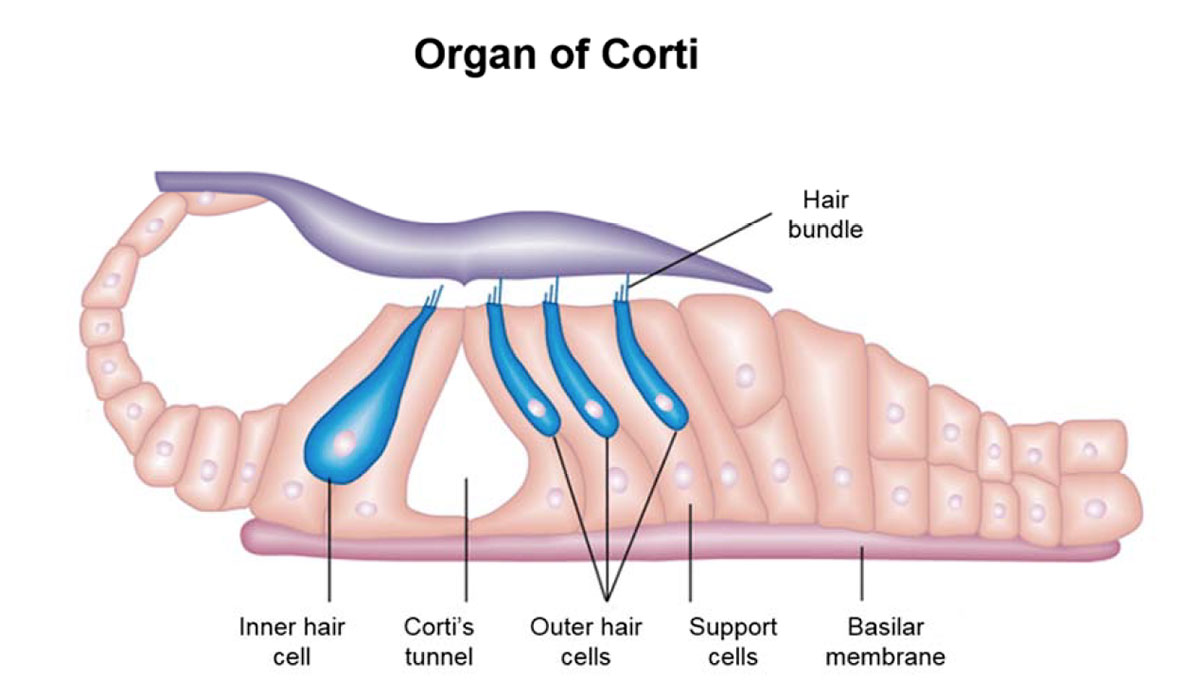
When the pressure waves travel into the perilymph within the cochlear duct from the scala vestibuli above, they cause the basilar membrane to ripple, and this causes the organ of Corti which sits atop it to move differentially relative to the temporal membrane. Three rows of outer hair bundles and one row of inner hair bundles (Figure 6) are in light contact with the tectorial membrane. These “hairs” are actually tiny, stiff protein filaments called cilia. When the basilar membrane ripples, the filaments deflect, allowing potassium-rich endolymph to enter the receptor cells that the hairs are anchored in. The bases of the receptor cells are surrounded by perilymph which is sodium-rich, and this difference in the ion content of the fluids on either side of the cell wall creates a potential difference. Voltage-gated channels in the lower cell walls open, allowing calcium ions to enter. Three things then happen.
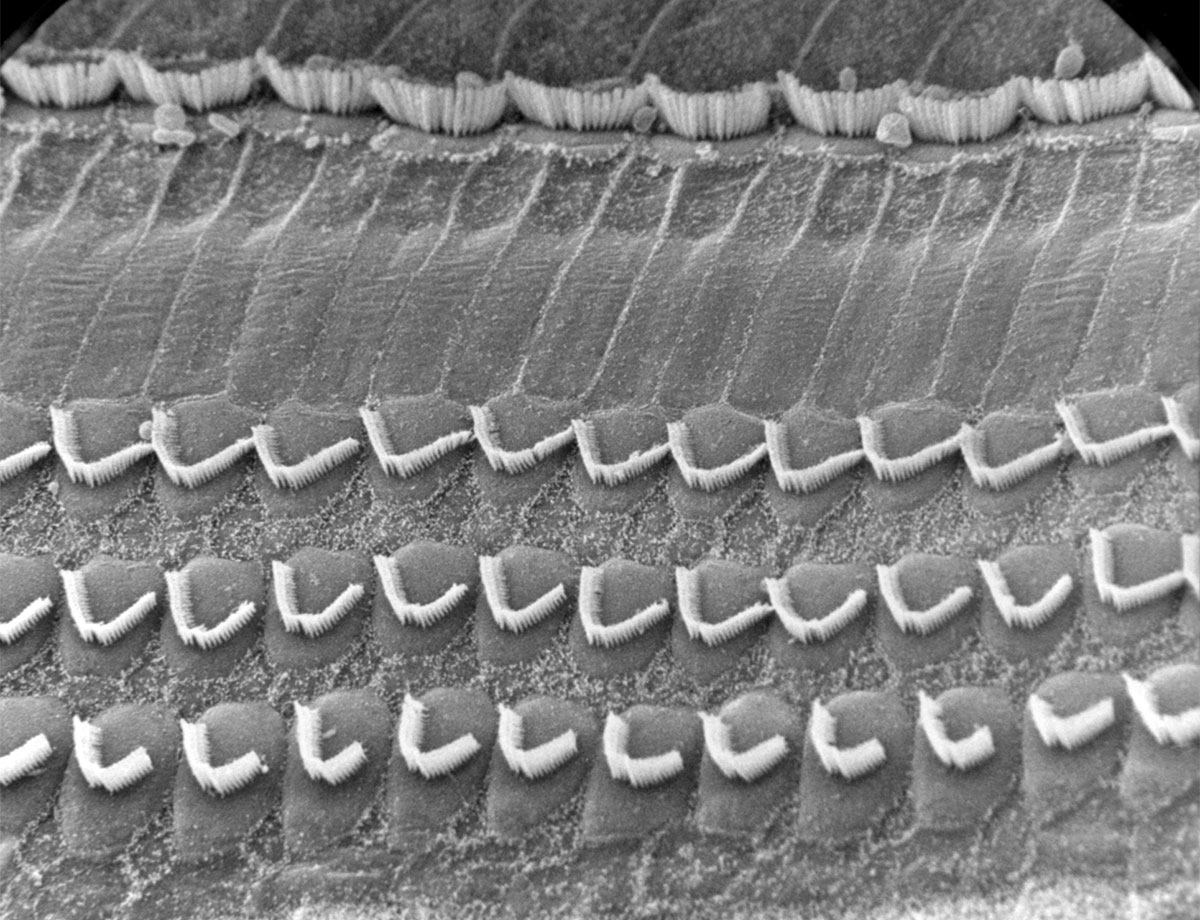
First, the presence of the calcium ions causes vesicles located at the base of the receptor cell to release an excitatory neurotransmitter (thought to be glutamate), which triggers afferent nerves immediately below the cell. Thus excited, the nerve cells create a pattern of action potentials. Without getting into too much more detail, an action potential occurs when the membrane potential within a nerve’s axon rapidly rises and falls, which causes the neighbouring nerve cell to do the same thing, and so on; it is the basic mechanism that transmits electrical signals through nerves. In this case the signal enters the spiral ganglion, then follows the vestibulocochlear nerve to the temporal lobe of the brain, which starts the process of translating the electrical patterns into our perception of sound. Second – and this is still an area of active debate – depending on the voltage potential inside the hair cells, a motor protein called prestin found inside the outer hair cells can contract, thus causing the cell to pull on the basilar membrane, amplifying the amount of ripple experienced by the inner hair cells. In some way that is not yet fully understood, the ear is thus able to amplify weaker signals. Third, calciumactivated potassium channels open, allowing the potassium ions to be drawn out into the potassium-poor perilymph, thus quickly repolarizing the receptor cell and leaving it ready for the next signals.
The organ of Corti, which travels up the entire length of the scala vestibuli, contains approximately 16,000 filaments and their corresponding receptor cells. The organ develops in such a way – organization, varying stiffness, size, etc. – so that each section is tuned to a band of frequencies. In particular, the basilar membrane is thick and stiff near the bottom, and loose near the top. Also, the scala vestibuli has a smaller diameter near its base, which increases towards its apex. Due to this, the cells near the base are tuned to the highest frequencies in our range of hearing (~20 kHz), and those at the top the lowest (~200 Hz). This makes sense as attenuation would be naturally biased towards the higher frequencies. There are many causes of hearing loss, but a common one is exposure to loud noises – this can physically damage the hair cells. There are many great images of healthy versus damaged hair cells available on the Internet (Figure 9), and they are a very graphic reminder of the dangers of exposure to very loud sounds, such as industrial noise, loud concerts or ear buds turned up too high. Because the sound waves hit the high frequency end of the cochlear duct first, damage due to loud noises tends to occur here rather than further along.
Static Balance
Static balance, basically a sense of the head’s position relative to gravity, is provided by the saccule and utricle, using mechanisms similar to the cochlear ones described above. The saccule and utricle are membranous organs, filled with endolymph, located within the bony vestibule. Within the saccule and utricle are hair cells, like those in the organ of Corti, whose purpose is to detect linear accelerations of the head.
The saccule responds to head tilts in the vertical plane. On its inner anterior wall there is a 2 mm x 3 mm oval thickening called the macula. A cross-section of a macula is shown in Figure 7.
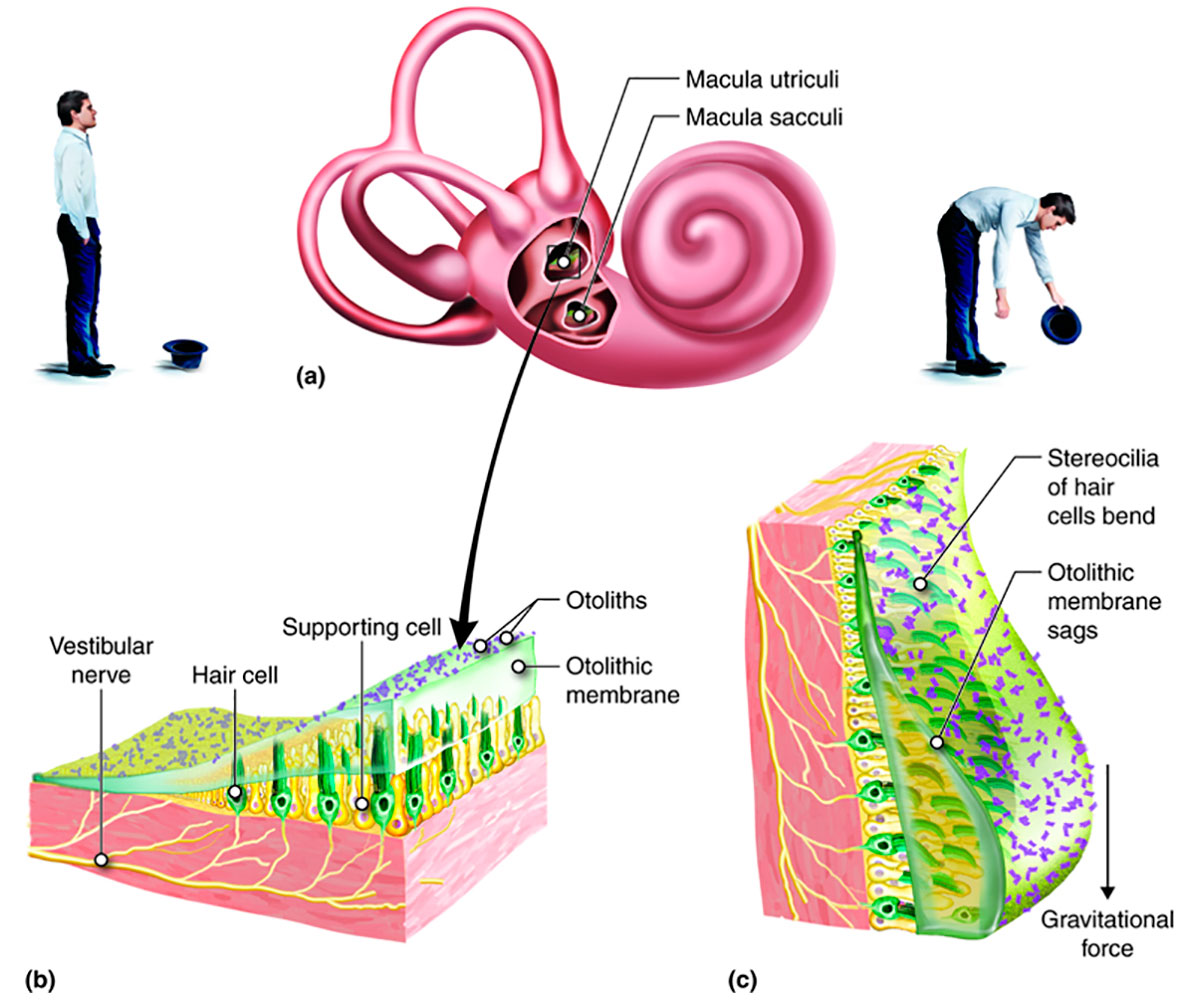
Each hair cell within the macula holds filaments: ~ 70 stereocilia, and a single longer kinocilium. Each cilium is physically linked to its own ion gated channel within the receptor cell. At their other end they project into a gelatinous layer known as the otolithic membrane. The top of the membrane is covered by a layer of calcium carbonate crystals called otoliths. These crystals are about 3 times denser than the surrounding endolymph, so when the head tilts the entire layer flops to one side. This deflects the cilia, triggering a response in the receptor cell similar to that described for the cochlea, resulting in electrical signals being sent to the brain.
A curved ridge called the striola runs down the centre of the macula and represents an axis of symmetry for the hair cells. Each hair bundle is arranged with the single kinocilium at one end, followed by all the stereocilia in decreasing order of height, and this polarity within the hair bundles is opposite on the two sides of the striola. The long kinocilium is the only filament which actually triggers a response within the receptor cell, but the other cilia are attached to it by tip links and this allows them to play a role in directional sensitivity. When the stereocilia bend towards the kinocilium they cause depolarization within their hair cells which excites a response; when they bend away from the kinocilium they cause hyperpolarization and an inhibitory response - this is how the brain detects the direction of tilt.
The utricle is very similar to the saccule, except that its macula is found on its floor. It responds to head tilts in the horizontal plane. Together the two vestibular organs provide the brain with enough information to gauge the head’s position relative to the gravitational field.
Dynamic balance
The three semicircular canals or ducts send information to the brain related to dynamic balance. As with the other membranous organs they are filled with endolymph. The three canals (Figure 8) are orthogonal to each other, the same as a 3C geophone! All three canals open to the vestibule at both their ends, but each has an osseous ampulla at one of its ends. An osseous ampulla is a bulbous, spherical sac, about twice the diameter of its semicircular canal. It is within the osseous ampullae that the mechanoreceptor hair cells are located, and signals to the brain are generated. At its base is a crest, the crista ampullaris; within the crest is a gelatinous component called the cupula, which contains hair cells (Figure 8).
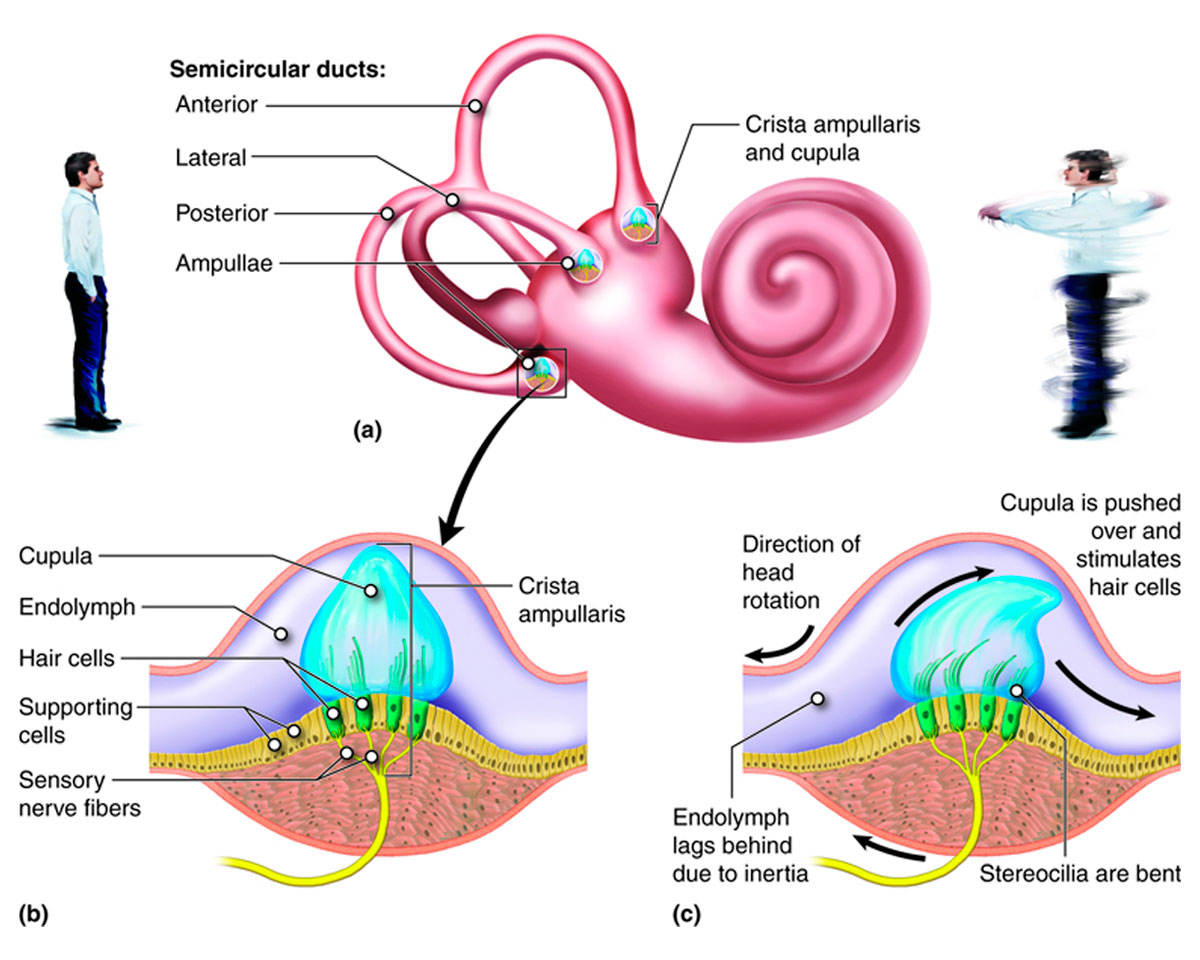
Each canal is associated with one plane of rotation – pitch, yaw and roll to put it into nautical or aeronautical terms. The movement of endolymph within the lateral semicircular canal (12-15 mm long) occurs when the head is rotated left or right, like a shoulder check when driving. Endolymph moves in the superior semicircular canal (15-20 mm long) when you nod your head up or down, eyes forward. Tilting your head left or right, eyes forward, as when moving an ear towards a shoulder, causes endolymph to move in the posterior semicircular canal (18-22 mm long). Obviously most head movements involve more than one plane of rotation, but the combined signals received from the semicircular canals allow the brain to figure out exactly how the head is moving.
When fluid flows across the ampulla, it perturbs it, thus deflecting the hair cells within. When the head moves, due to inertia there is a lag before the endolymph moves. This differential movement causes acceleration of the fluid relative to the duct/ampulla/cupula, and this is what ultimately triggers the response within the hair cell. As within the maculae, the cilia within each hair bundle are directionally organized with a single longer kinocilium always at one end, thus the signals to the brain indicate direction of acceleration, via the same inhibitory/excitatory mechanism described earlier. Note that the specific gravity of the cupula is identical to that of the endolymph, meaning that the responses within the semicircular canal system are unaffected by gravity, and thus only triggered by dynamic acceleration caused by movement of the head.
Common ear ailments
A full investigation of ear-related ailments is well beyond the scope of this article; however, a few common and/or interesting ones are covered here.
Hearing loss
The most common type of hearing loss is temporary. This can be caused by blockage in the ear canal, such as from water that gets in while swimming, or an excessive buildup of wax. Colds, ear infections, allergies and so on can all cause blockages and the collection of fluid within the middle ear, which can temporarily impair hearing.
Longer term or permanent hearing loss can be the result of many different things, including age (which could also be viewed as cumulative damage), genetics, trauma, exposure to loud noise, the effects of drugs, and illness (e.g. Ménière’s disease). To simplify things one can divide hearing loss into two broad categories: problems within the inner ear which are termed sensorineural, and problems within the middle ear, which are of a more physical or mechanical nature.
An example of inner ear hearing loss is when the organ of Corti stops working properly. Often this is due to damage to the hair bundles from exposure to loud noise, disease, age, or the effects of drugs (Figure 9). One way to restore hearing in this case is through the use of a cochlear implant. A small microphone located externally, usually directly behind the ear, picks up sounds and converts them into an electrical signal, which is transmitted via a tiny coil. A receiving coil (the implant) is located close by under the skin. The signal is then sent via a long, thin, flexible electrode which extends up the coils of the cochlea and attaches directly to the auditory nerve. The signals are not exactly like those generated by a healthy ear, so it takes the brain a while to learn how to translate them. This is why the effectiveness of cochlear implants varies from person to person.
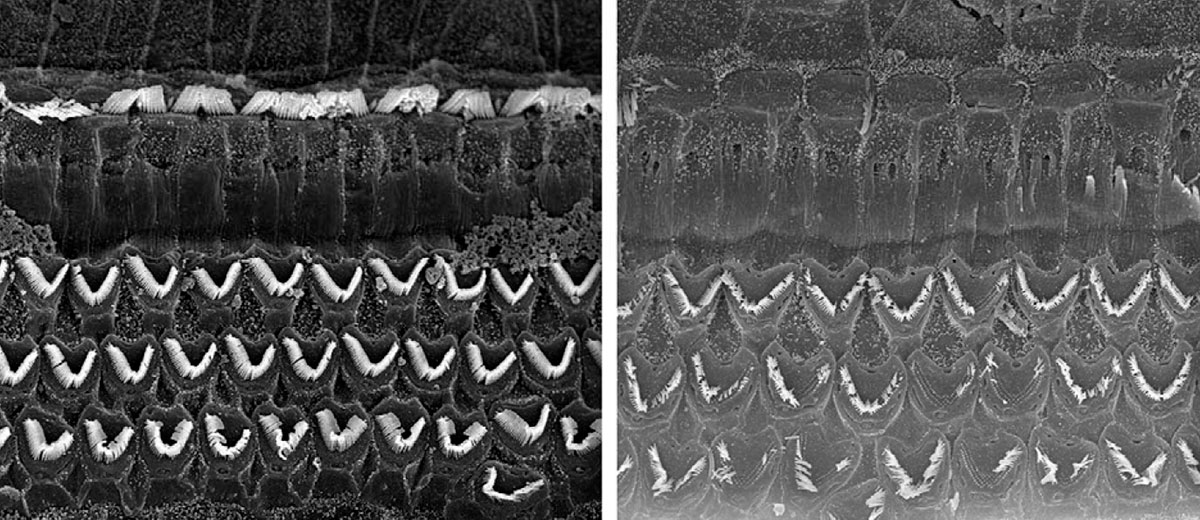
Problems in the middle ear are usually due to three main causes involving the ossicular chain. First, abnormal bone development (e.g. unnatural enlargement or fusion) at the fetal stage can occur. Second, otosclerosis describes a situation where abnormal bone growth impairs the chain’s ability to transmit vibrations from the ear drum to the oval window. This can be an inherited condition, or possibly caused by disease, or hormonal changes in pregnant women. Ossicular chain discontinuity, a third type of problem, can result from one of the joints between the incus, malleus and stapes being either dissolved due to a severe ear infection, or physically damaged, often by Q-Tips that penetrate the ear drum. There are surgical ways to correct these situations. Delicate operations can restore function to the ossicular chain. A more aggressive approach is to replace the entire ossicular chain with a mechanical piston-like apparatus that is inserted between the ear drum and the oval window that simulates the function of the ossicular chain – this procedure is called a stapedotomy.
I have heard of an interesting new type of hearing aid implant. It has the same external microphone plus battery as a traditional cochlear implant. The internal implant however is an electro-acoustic transducer that is fixed to the middle ear side of the round window. The connection between the internal and external components is wireless. When a signal is received by the transducer, it flexes and sends waves backwards up the cochlea, I believe 180 degrees out of phase. In this way it complements the impaired signal that may be coming the other way, but still makes proper use of the inner ear functions. An advantage is that the surgery to insert the implant is simpler and less invasive.
A third type of hearing aid should be mentioned. This is simply a device that is inserted directly into the ear opening. A microphone receives incoming sound waves, which it then amplifies and projects via a speaker directly towards the ear drum. This type of hearing aid has been around for decades, and simply turns up the volume.
Tinnitus
Tinnitus is a term that describes when a person hears phantom sounds that aren’t occurring in the outside world. This situation can be caused by a number of things, and the sound experienced by the sufferer can be anything between a high-pitched buzzing to a lower frequency roaring; the apparent volume can vary between high and low, and the sounds can be always present or intermittent. The most common cause is damage to the hearing hair cells, hence because the cells detecting higher frequencies are the ones most commonly damaged, the most common type of tinnitus involves the person hearing high pitched ringing or buzzing. In this case, the damaged hair cells send signals to the brain causing it to believe there are sounds being received when there aren’t really. Other, less common, possible causes include Ménière’s disease, TMJ disorders, tumours on the acoustic nerve, Eustachian tube problems, and medication side effects.
Barotitis
Barotitis is caused by external pressure changes and affects the middle ear. Symptoms include inflammation, ear pain, dizziness, ringing, and impaired hearing. Classic situations when barotitis tend to occur include airplane takeoffs and landings, scuba diving descents/ascents, and sky diving. It is usually associated with blocked Eustachian tubes, but some people’s middle ears, even in a healthy state, are naturally poor at being able to equalize pressure. For people like this, even pressure changes caused by high amplitude, low frequency sounds can trigger barotitis symptoms. Because the pressure imbalance is within the middle ear, there is no physiological explanation for pressure imbalances to cause balance problems within the inner ear. However, certain conditions can create such a cause and effect. Pressure changes experienced by migraine suffers can distort sensory inputs, including those related to balance. Perilymph fistula and superior canal dehiscence can both cause hydrodynamic problems within the inner hearing, affecting balance. The former is a tear or defect in either the oval or round window, which allows perilymph fluid to leak into the middle ear. The latter is a hole or thinning in the bone above the semicircular canal. Both these conditions can result in loud sounds affecting balance and creating vertigo-like symptoms.
Vertigo
Vertigo is the term given to a group of symptoms related to a few causes, some quite different and unrelated. These symptoms include dizziness, nausea, headache, and loss of balance.
Benign paroxysmal positional vertigo (BPPV) is one interesting cause of vertigo. What happens is that one or more otoliths become dislodged from their proper spot within the utricle, and due to proximity, find their way into the semicircular canals. As you can imagine, having these dense calcium carbonate crystals rattling around within the semicircular canals causes all sorts of conflicting signals to be sent to the brain, creating a sensation of not knowing how your head is oriented relative to the outside world. With one extreme type of BPPV the otolith adheres to the surface of the cupula, which then is not only affected by the static gravitational field, but also flops around in an exaggerated fashion sending wildly erratic signals to the brain.
Recently during a squash tournament, I noticed a friend of mine was not playing very well. He appeared to be lacking a sense of balance, and was mis-hitting the ball, sometimes missing it entirely. It turned out he was suffering from viral vertigo, sometimes known as labyrinthitis. It is caused by a viral inner ear infection, and the vertigo symptoms disappear once the virus has run its course. Vestibular neuronitis can also result in temporary vertigo symptoms. It is caused by inflammation of the vestibular nerve; the inflammation messes up the balance-related signals sent to the brain.
Share This Column