The threat of a modern-day plague is definitely real. Every day brings more news on super bugs, antibiotic-resistant bacteria, and the non-development of new antibiotics. And glimpses of what might be just around the corner, such as outbreaks of Legionnaires' disease on cruise ships, or hospital wings being shut down due to mass staph infections, help stoke our worst fears. It is certainly something I worry about – why wouldn’t I? Apocalyptic fears are mostly generated within our imaginations, but plagues are a very real and well documented threat to the human species. It is clear how significantly past plagues shaped the course of human history, especially since we began living within larger, higher density communities in the last 5,000 to 10,000 years.
Plagues may seem like a thing of the past, but they are really quite recent. My own father was born in 1919, right in the middle of the Spanish Flu plague, which infected half a billion people, killing somewhere between 50 and 100 million of them, 3-5% of the world’s human population at the time. That’s two or three times Canada’s population dead in a couple of years. Since then, it is only the discovery of antibiotics that has provided a temporary defense against similar outbreaks. On the one side is a horde of constantly mutating bacteria evolving into new resistant forms, on the other billions of pounds of a single species, often tightly packed, ripe for invasion. Our only hope is in the hands of big pharma, who are more interested in addressing erectile dysfunction and other afflictions of aging baby boomers than developing new antibiotics.
Recently medical research is looking back towards bacteriophages (Greek – bacteria eaters) as an alternative to antibiotics, in the form of what is known as phage therapy. This type of treatment is 100 years old, but outside of the former Soviet Union, and a few little pockets here and there, research on it largely stopped when penicillin was discovered in 1928. In this article I will explain what bacteriophages are, how they are used in phage therapy, and I will touch on the interesting history of phage therapy.
What is a bacteriophage?
Bacteriophages are viruses that attack and replicate within bacteria and archaea (both single celled microorganisms, the latter being distinguished by their lack of nuclei). In appearance they resemble lunar landing modules, probably because they perform a similar function. A bacteriophage will “land” on a much bigger prospective host and inject its genome into that host’s cytoplasm (Figure 1). The genetic processes of the host bacterium or archaeon are then hijacked to replicate the attacking bacteriophage. The net result of each of these attacks is more bacteriophages and one less bacterium or archaeon. Bacteriophages are the most common organic entities on Earth – even more of them exist than bacteria – with their numbers estimated at ~1031. Of course they are not all the same, as each type of bacteriophage is “designed” to exploit a specific type of bacterium or archaeon.
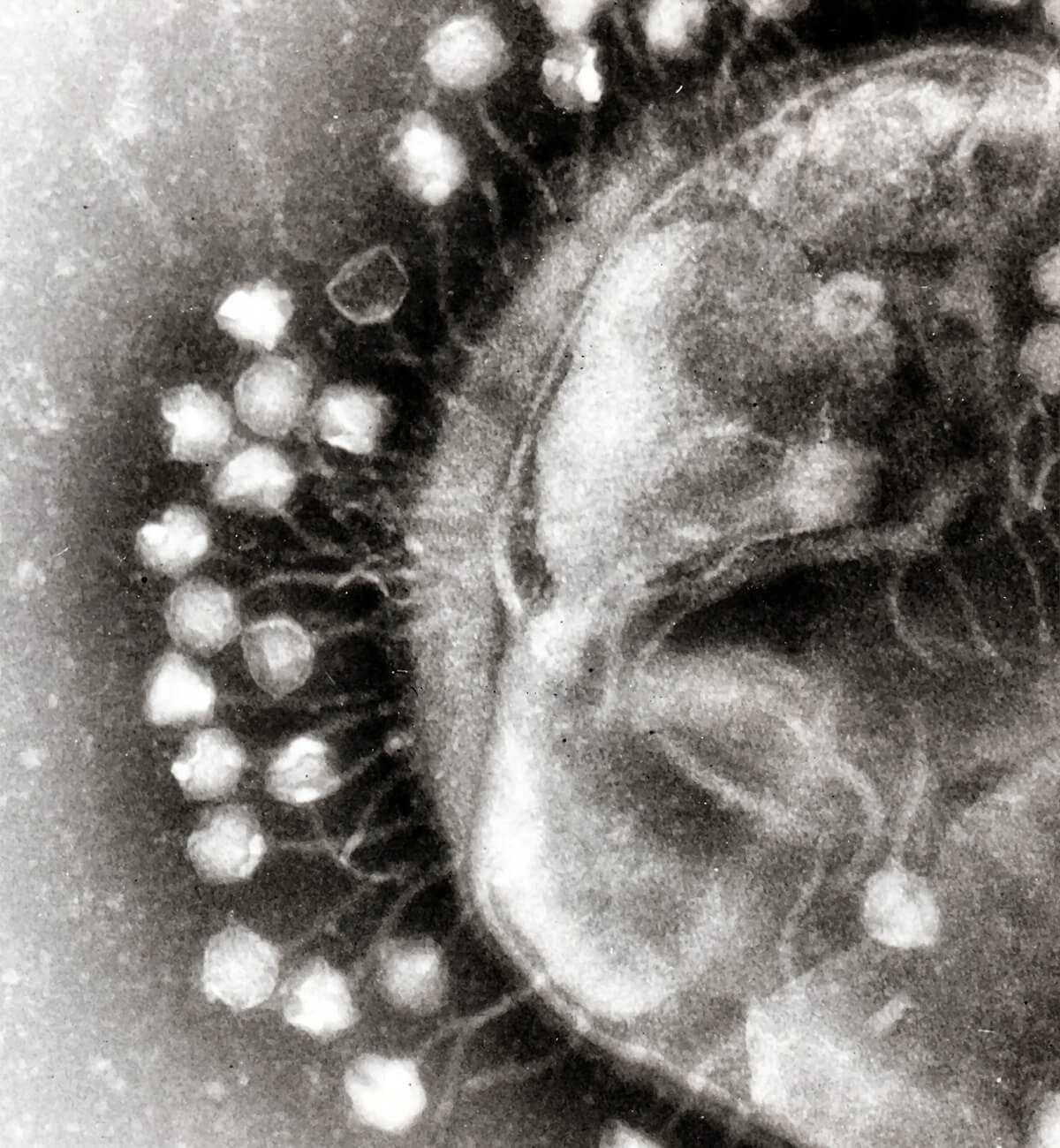
Figure 1. Bacteriophages (likely Coliphage T1) attached to a bacterium, at 200,000 times magnification. Used under Creative Commons license 3.0 (Beards, 2008).
Replication
Figure 2 shows the anatomy and life cycle of a T4 bacteriophage (more precisely, an Enterobacteria Phage T4). The T4 is a double-stranded DNA (dsDNA) contractile-tailed virus that infects E. coli (Escherichia coli) bacteria. It is a lytic phage, meaning that upon replication it destroys its host. The term lysis refers to the breakdown of a cell’s membranes. It is only lytic phages that are useful for phage therapy, for obvious reasons. The other main type of phage, lysogenic, does not destroy its host unless its condition deteriorates; until such time, the phage resides and replicates within its host, and indeed as its host cell itself replicates, phages are carried along in its offspring. This brings me back to my very first Science Break article “Parasitoids” (Kuhn, 2008). There I contrasted parasites, which do not kill their hosts, with parasitoids, which do. Lysogenic and lytic are analogous terms, albeit at a microscopic scale.
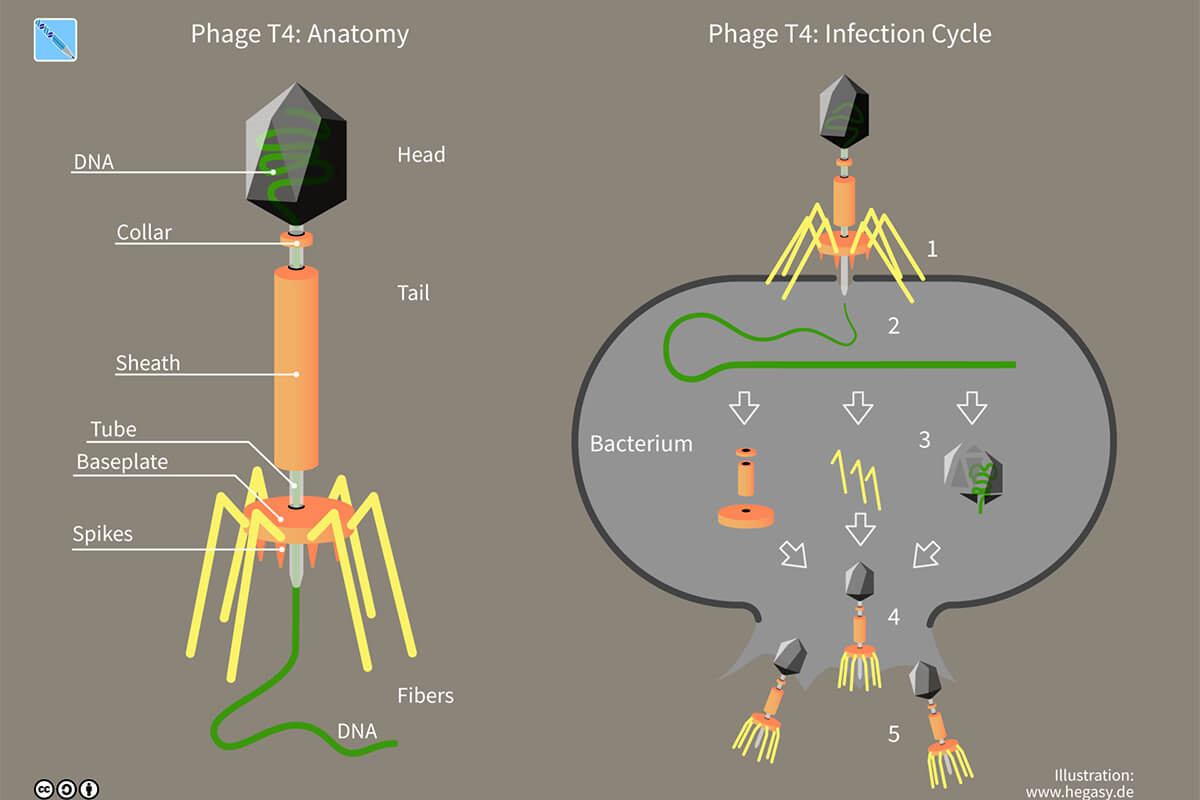
Figure 2. Anatomy and infection cycle of phage T4, used under Creative Commons license 4.0 (Wikimedia Foundation, Inc., 2017)
The five steps indicated in Figure 1 are detailed below. The entire T4 infection cycle, from attachment to release, takes about 30 minutes at 37 C temperature.
Attachment
Bacteriophages rely on pure chance encounters to attach to suitable hosts. They float around in whatever medium they happen to be in – blood, sewage, sea water, mucus…. they are literally everywhere – and when they bump into a bacterium or archaeon with suitable receptors, they attach. They key point is that each type of phage requires a very specific type of receptor to attach to. The desired receptor could be a physical feature like the flagella used by pro- and eukaryotes for propulsion, or features with certain chemical properties found on outer cell walls or membranes. For example, many staphylococcus and streptococcus bacteria have unique teichoic acid structures in their outer cell walls, and specific types of phages latch on to these acids.
Injection
Once attached, there are various mechanisms used by the huge range of phages to inject their genomes into their hosts. Myoviruses (family Myoviridae; the T4 is one) use their tails to inject. They push their base plates down to lock onto the host’s surface, flex their tails to pierce the host’s cell wall, then inject their genome much like a hypodermic needle. Podoviruses on the other hand lack tails, and so they have teeth-like appendage at their bases which grasp onto the host surface. Within the ring of “teeth” an enzyme is secreted which breaks down the cell wall of the host, and the genetic material oozes in. There are many interesting variations, but the end result is that the phages transfer their genomes into their hosts.
Synthesis
Ribosomes found within the host’s cytoplasm immediately start following the instructions from the phage’s mRNA, in addition to those of its own mRNA. Ribosomes are like little workers that assemble raw amino acids into specific proteins. The first proteins constructed by the viral RNA are often designed to alter the host RNA polymerase so that it gives higher priority to following viral mRNA instructions. Once the initial “hijacking” is accomplished the viral mRNA instructions are followed to create all the components required for replication of the invading phage. Phages are relatively simple structures – for example the T4 consists of not much more than the components labeled in Figure 1 – so the replication of parts happens quite quickly.
Assembly
As components are constructed within the host cell, they are assembled into complete new phages, often with the assistance of helper proteins. Assembly, indeed all the steps, involve many interesting biochemical steps and processes that I will not get into here.
Release
The T4 injects enough material into its host to create between 100-150 new phages. Once the final number is complete, the host cell bursts (and is destroyed in the process) and the newly replicated phages are released to each repeat the process, thus there is an exponential aspect to the cycle.
Phage therapy
History
There is a very interesting geopolitical aspect to phage therapy. The discovery of bacteriophages (~1919) coincided roughly with the Russian revolution (1917). By the time penicillin was discovered (1928), the Soviets were firmly established, and they viewed penicillin and its association with the West with suspicion. A politically-derived fork in the road of medical research was established, with the West pursuing the development of antibiotics, and the Eastern Bloc countries focusing on phage therapy. So, while phage therapy may appear to us in the West as a new and exciting alternative to antibiotics, the reality is that it has been used and researched in Russia and some former Soviet bloc countries for almost 100 years.
There is a Canadian component to the discovery of bacteriophages. In the early 1900’s various scientists had observed what they called “factors”, which killed bacteria. It was an Englishman, Fredrick Twort, who first formally suggested that the factors were viruses which were infecting and killing the bacteria. Two years later at the Institut Pasteur in Paris, a Canadian-born microbiologist, Félix d'Hérelle, was the first to propose bacteriophages as a way to treat bacterial infections in humans. In 1919 he and his team did just that. After ingesting phages themselves to ensure that to do so was safe, they administered phages to a 12 year-old boy on the verge of death due to dysentery...he quickly recovered. Although d'Hérelle did not formally publish these results until 1931, the larger medical research community, especially in eastern European countries, was aware of phage therapy and actively researched it. With the discovery of penicillin, western researchers largely abandoned bacteriophages, but the Soviet bloc stuck with phage therapy, probably due to partly political pride, partly lack of access to penicillin. Centres of phage therapy expertise developed in Russia, Poland and Georgia (the Phage Therapy Center in the references is located in Tbilisi, Georgia).
Eli Lilly and Co. made an effort to market phage therapy products in the US in the 1940’s, but this failed mainly due to shortcomings in transport and storage, and the need to supply exactly the right type of bacteriophage to kill the unwanted bacteria. The very general effectiveness of antibiotics, especially evidenced by its use among the Allies in WWII, crushed phage therapy, which didn’t work unless you used exactly the right type of phage.
The Cold War effectively established a barrier between the West and East bloc phage therapy research, which remained until the late 1980’s. Today, the use of phage therapy is an extremely active area of research, especially in light of the evolution of antibiotic-resistant “super bugs”.
Advantages
The specificity of bacteriophages that I referred to earlier – in many cases a particular phage can only attack a very narrow range of bacteria hosts, often only a single species; some are even limited to specific strains within a species – represents a big advantage of phage therapy over antibiotics. Whereas antibiotics often attack all sorts of good bacteria such as those found in our digestive systems, bacteriophages used for therapeutic purposes can be chosen to be extremely specific in terms of the bacteria they attack and destroy, thereby minimizing unintended side effects.
As we are finding out, a bacterium can evolve to become resistant, even immune, to antibiotics. Once this happens it can take years to develop new replacement antibiotics to effectively combat the super bug. Bacteriophages are able to evolve just as quickly as bacteria, and in fact have been engaged in such Darwinian struggles with bacteria since time immemorial. If a particular bacterium evolves and develops a resistance to a particular bacteriophage, then it is likely that the bacteriophage itself will evolve new strains in response, in a matter of hours, days or weeks. The new bacteriophages will be found in the same environment as their prospective hosts, and it is simply a matter of identifying and isolating the new bacteriophages in order to develop an effective phage therapy treatment.
Also, bacteria that turn into super bugs tend to be resistant to a broad range of antibiotics, whereas bacterial resistance to phages tends to be very specific – a bacterium’s developed resistance will usually be limited to just one type of bacteriophage and it will likely still be susceptible to other bacteriophages with an overlapping target range. So, the type of concerns we have around the overuse of antibiotics and the development of broad resistances is simply not an issue with phage therapy.
Process
The first challenge in phage therapy is finding the right kinds of phages. As previously mentioned, they tend to be found right among the very bacteria they require to replicate, understandably. This means researchers look for phages in the places the target bacteria are found – sewage, infected patients’ stool, etc. So that part of the process is nasty work, but the payoffs are worth it. Note the use of phages is not limited to treating humans. For example, phages can be administered to farm animals, salmon farms, beehives, sprayed on food, etc. The uses are almost limitless, since harmful bacteria (and their corresponding bacteriophages) are so ubiquitous.
A simplified description of the process is given by the following example. Let’s say there has been a cholera outbreak in a region (technically, the bacterium Vibrio cholerae). First, samples of water would be taken from obviously filthy streams in the area. The lab technicians would add these collected samples systemically to samples containing the target Vibrio cholerae. If they observe the cholera bacteria dying off, then they know they have a winner. After centrifuging the mixture to collect a concentration of the phages, they would determine which are lytic and which are lysogenic. That done, they would amplify the numbers of the desired lytic phages by feeding them on a culture, then filter and purify this larger batch, and distribute the samples in usable sizes to the clinics participating in the phage therapy campaign.
The form of treatment depends on the target. In the case of outbreaks like cholera, oral doses would be the most common form of treatment; in the case of localized infections such as wounds, or internal abscesses, applying the phage sample directly to the location would be the norm. Injections are rarely used as the immune system tends to fight all viruses entering the bloodstream.
In practice the process is more complicated, in large part due to the problems encountered by early attempts, such as with Eli Lilly in the US. Care must be taken to store and transport the phage samples. Great advances have been made in this area. For example, it is now possible to store phages in a wide variety of forms such as freeze dried, pills, and liquid in refrigerated vials. All the advances in modern supply chain management and logistics have made the transport of phage treatments much easier and cheaper. Oral treatments often contain antacids to combat the destructive effects of stomach acids on the phages. Improvements such as these are continually being made as the phage therapy appears to be on a trajectory towards being a commonly used method.
In most cases it is rare that only a single type of bacteria is the problem. For example, a wide variety of bacteria will find their way into an open wound, and all sorts of nasty bacteria found in a village’s drinking water can lead to dysentery symptoms, so at the collection stage technicians need to identify a range of bacteria and the phages which use them to replicate. Thus the doses used for phage therapy are more likely to contain a cocktail of bacteriophages rather than one specific type. Because phage replication happens so quickly and is inherently exponential, successful phage therapy results are equally prompt.
Risks and Challenges
There are certain bacteria for which corresponding lytic bacteriophages have not yet been identified (e.g. Clostridium difficile). There are risks beyond the difficulty of identifying and isolating the exact types of phages required. When phages successfully attack and kill bacteria, endotoxins are often released. These can cause fever and even toxic shock in the patients. Researchers are working on ways to address this potential risk.
The health industry is ultimately profit-driven, the same as any other sector, and this business side also poses challenges to the successful development of large-scale phage therapy expertise and use. Under the current patent system, it is difficult for labs to protect phages they isolate and develop, because of the constantly mutating and evolving aspect of the bacteria-bacteriophage contests. Contrast that with static antibiotics which can be accurately described, patented and protected. The medical regulatory system is generally against the use of self-replicating biological agents capable of mutation, probably for good reasons, given the risk of uncontrolled exponential growth of a rogue agent. Bureaucratic inertia is also a real challenge – the entire huge health industry heavily relies on the use of antibiotics and is comfortable with the status quo; at times it appears to be loath to accept and incorporate alternatives.
Recent success
Phage therapies are being routinely used in Russia, Poland and Georgia, and over the counter phage cocktails are available in most eastern European pharmacies. The labs which create these cocktails are continually modifying the types of phages in the doses, to address the most current and common bacterial outbreaks in a particular region. In the West phage therapy is still at the experimental stage, with various countries such as England, the US, France and Belgium all recently approving different clinical trials.
One recent high profile case in the US made the rounds on TV talk shows and resulted in a book. In fact, it was a CBC interview with the successfully treated patient and his wife which inspired me to investigate bacteriophages and write this article. Tom Patterson, a 69-year old professor of psychiatry at the UC San Diego School of Medicine, was infected with a multidrug-resistant strain of Acinetobacter baumannii while on vacation in Egypt. After conventional antibiotics proved ineffective, a last resort treatment involving the antibiotics meropenem, tigecycline and colistin stabilized his situation, although the bacteria developed a resistance during treatment. Before he could recover, an internal drain designed to localize the infection slipped, allowing bacteria to spill into his abdomen and enter his bloodstream. He immediately went into a septic shock coma. The doctors had run out of ways to cure him, so he entered an inexorable drift towards death.
At this point, his wife Steffanie Strathdee really sprang into action. Being an infectious disease epidemiologist and Director of the UC San Diego Global Health Institute, she well understood his situation and the dire straits he was in. Knowing conventional approaches offered no further hope, she looked into alternatives, and stumbled upon phage therapy, which she vaguely remembered from medical school. Pulling together an ad hoc team from various US universities with the necessary expertise and phage samples, she lobbied for and received approval from the FDA to conduct a clinical trial with Tom as the patient. After 3 days of phage therapy he emerged from the coma, and 5 months later he was discharged infection free.
While appearing to be a miracle cure, Patterson’s treatment was fraught with challenges and setbacks, and the health industry still needs to do a lot of work before phage therapy becomes routine. During treatment the pathogens continually mutated and developed resistances to the phages used, but the medical team was able to isolate and replicate new strains of phages to successful counterattack. Antibiotics were used throughout to combat numerous other bacterial invaders who waltzed into his body unchallenged by his weakened immune system. Even after the super bug was eliminated, it took months of therapy to recover his lost musculature and reverse the other debilitating effects of his brush with a killer strain of bacteria. Patterson’s case and the ability of Strathdee to effectively articulate the hope offered by phage therapy has no doubt helped accelerate public awareness and advance its use in the West, and we should expect to see it available in our hospitals before too long.
Share This Column