Over the years I have seen intriguing references to tardigrades and the unique properties these small organisms possess. Recently there have been headlines floating around claiming researchers had been able to entangle tardigrades at the quantum level. That just seemed too interesting to pass up – I had to look further into this. Before getting to quantum entanglement, I will describe some of the unique properties of tardigrades, which should explain why they were chosen to be participants in quantum experiments.
Description
Tardigrades are small, but not tiny – their typical size is in the range of 0.5 mm, with some varieties growing as big as 1.5 mm, so visible as small dots to the eye (like this comma), and very easily viewed under even a low power microscope. One of their hallmarks is that they can survive almost anywhere, from the bitter cold of antarctica to the extreme heat of hydrothermal vents, in very dry deserts and lush tropical ponds; they have even been shown to survive in outer space with no oxygen. In short, they can survive environmental extremes.
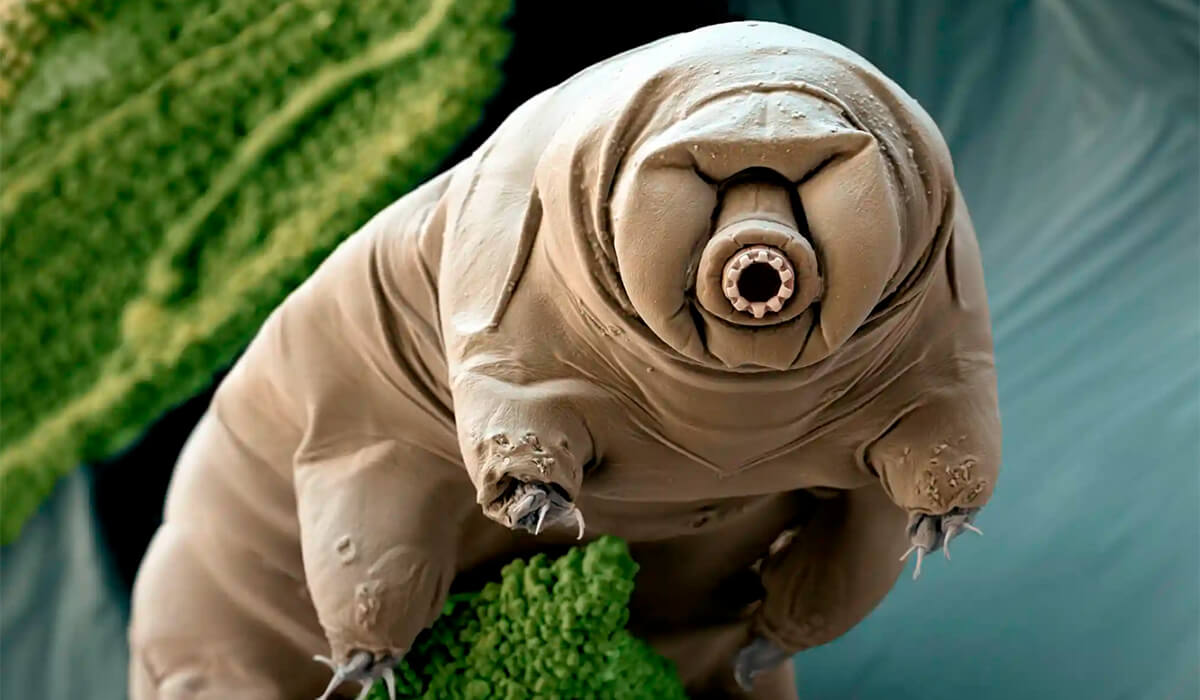
They are appealing to the human eye, resembling small stuffed animals, or perhaps characters in toddler anime videos (Fig. 1). The German who first described them in 1773 called them kleiner Wasserbären, or “little water bears”, and their scientific name derives from the Italian for “slow walkers”. They trundle along on three pairs of legs, with a fourth pair at the rear typically used to hold onto the lichens and mosses upon which they feed. Most species have claws at the end of their legs, but some employ suction cups. Tardigrades lack the genes which in insects are associated with the abdomen and thorax, so they are essentially like insects whose heads make up their entire body. Not surprisingly, tardigrades have featured in several sci-fi films and TV shows, but my favourite tardigrade appearance is in a South Park episode entitled Moss Piglets, in which they dance to a Taylor Swift song. Figure 2 shows a tardigrade’s basic anatomy.
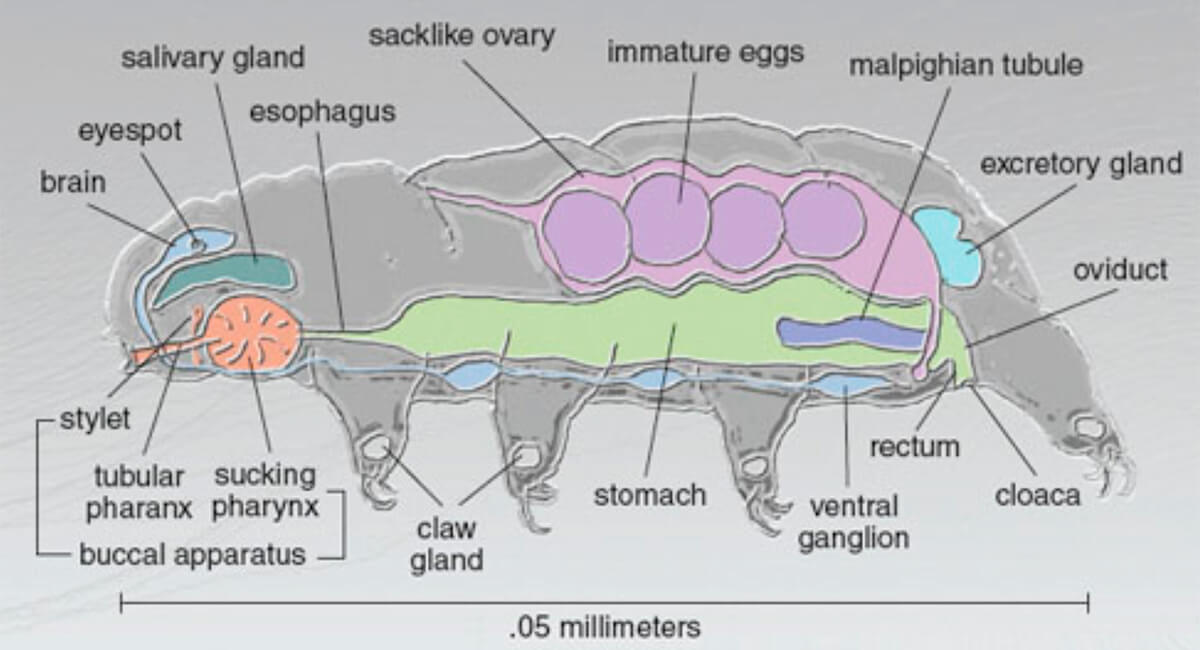
Tardigrades are not picky eaters. Their mouth openings contain stylets which are used to pierce animal or plant cells. A tube-like pharynx then sucks the nutritious juices out and sends them along to a more or less conventional digestive system.
There are approximately 1300 tardigrade species which make up the phylum Tardigrada. This phylum is part of the superphylum Ecdysozoa, which contains creatures that grow via ecdysis. Ecdysozoans have rigid exoskeletons or cuticles, and growth is achieved sporadically when moulting. The old cuticle splits open, and while the creature’s new exterior surfaces are still soft and pliable, fluids and sometimes air flow into the extremities causing the body to expand. The exterior soon hardens into the new exoskeleton, which then remains the same size until it is discarded in the next moult.
Most of the tardigrade species are considered terrestrial, but they require water to survive. This sounds contradictory but is explained by the fact that besides the ~150 species that live in marine saltwater environments (and generally do not possess the ability to survive extremes), the rest live on continents – in lakes, ponds, rivers, and anywhere that provides at the very least a film of water in which the tardigrades can live, even sand dunes. If this sounds like a very tenuous existence, it would be without a very unique collection of survival mechanisms, described in greater detail further down.
Reproduction occurs during a moult. The female typically lays her eggs inside her just discarded exoskeleton, and the male secretes his sperm onto the eggs. The parents then go their separate ways, leaving the exoskeleton as a nest of sorts for the eggs to hatch in. Note that depending on the species and circumstances, tardigrades are also capable of parthenogenetic and hermaphroditic reproduction, self-cloning and self-fertilisation respectively. Just an interesting sidenote here not related to tardigrades. Female spiders have a tendency to eat their male partners following mating. In some spider species the males escape this fate, as mating occurs while the female is in a callow state having just moulted and is unable to eat the male.
Survival mechanisms
Tardigrades are sometimes referred to as extremophiles, but strictly speaking this is inaccurate. Extremophiles have evolved to live their normal lives in an extreme environment, whereas tardigrades have evolved to survive extreme conditions on a temporary basis. Prolonged exposure to extremes will eventually kill them, but that said, they are remarkably hard to kill. They have been around since the Cretaceous and have survived the five major extinctions that have occurred since then. They have been capable of this due to their ability to switch between active, diapause, and quiescent states.
Figure 3 further breaks this down. The active state is what we would consider “normal”, where the tardigrades move around and engage in all the activities of their lives. Diapause is a dormant state, which in tardigrades, in response to a hostile external environment, involves the development of one or more extra cuticles which wrap around the original exoskeleton and form a protective armour around the dormant occupant. The other four states in the diagram are cryptobiotic: anoxybiosis at the top left, and three varieties of tun in the bottom row.
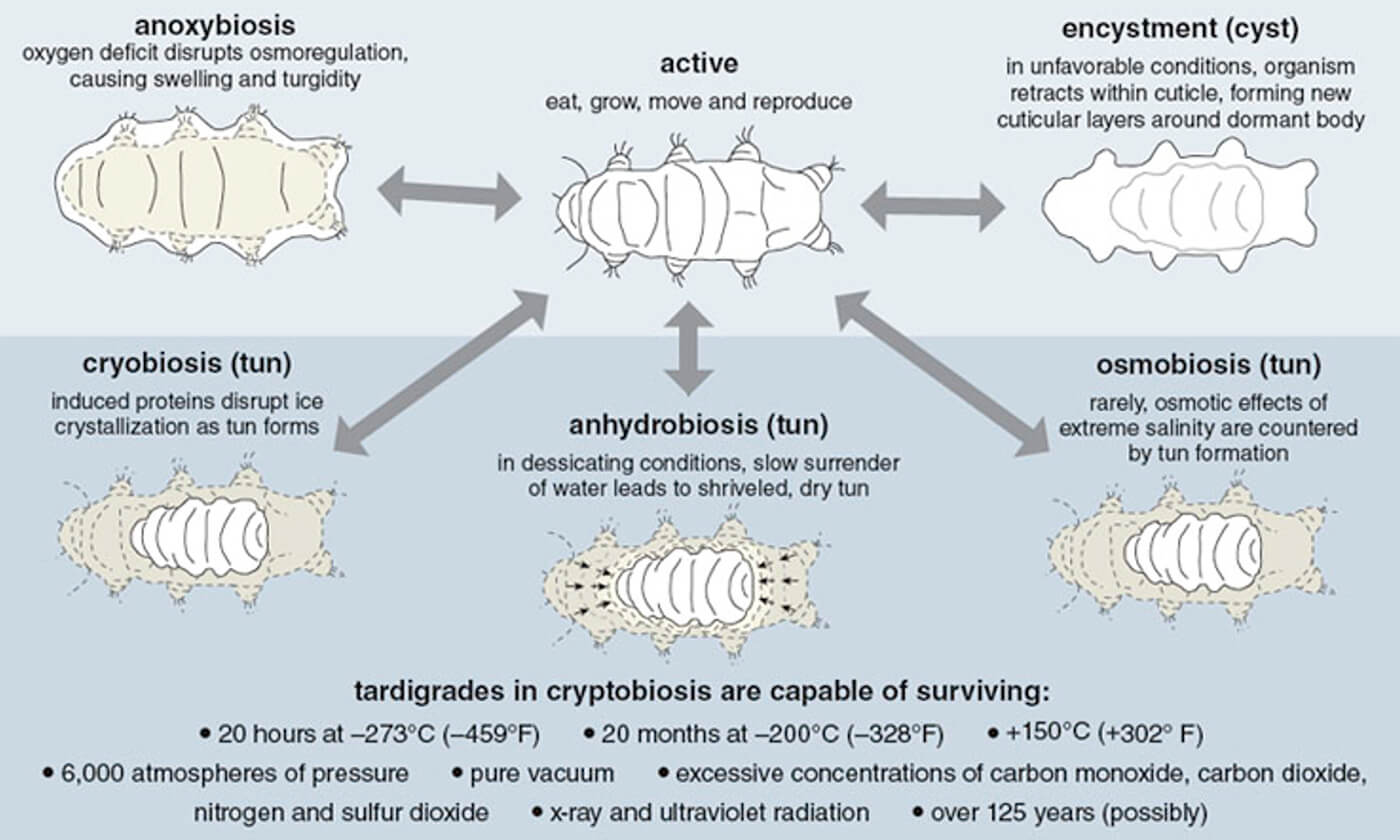
When in tun, tardigrades’ metabolic activity levels drop to less than 0.01% of normal, and their moisture content less than 1%. In this extremely desiccated state, it’s hard to say whether a tardigrade is dead or alive. Really, it’s more like its life has been put on pause while its body is in an entirely different state. The normal life span of a tardigrade is just a few weeks, but those few weeks can be interrupted by very long periods of tun. In one known case, moss from a museum display documented to have been totally dry for around 100 years was soaked in water, and tardigrades in it came back to life! This flip flop between states is normal for tardigrades, and allows them to live in almost every corner of our planet, no matter how hostile.
Cryptobiosis
The metabolic activity levels of an organism in a cryptobiotic state drop to extremely low levels, almost to zero. There are many variations of this in the animal kingdom, and tardigrade species seem to employ some or all of them.
Anoxybiosis
Anoxybiosis is the ability to survive in a turgid state in an extremely low or zero oxygen environment, which some tardigrade species are capable of. In some tardigrade species this is achieved by taking on excess water. Tardigrades in this state become bloated and appear dead, but when oygen returns to their external environment, they come back to their active state. Some scientists believe this state to be more like a form of dormancy, with higher levels of metabolic activity, but most experts believe it impossible for dormant organisms to prevent cellular damage while in an anoxic environment, and that in tardigrades this is actually a form of cryptobiotis, with all functions shut down.
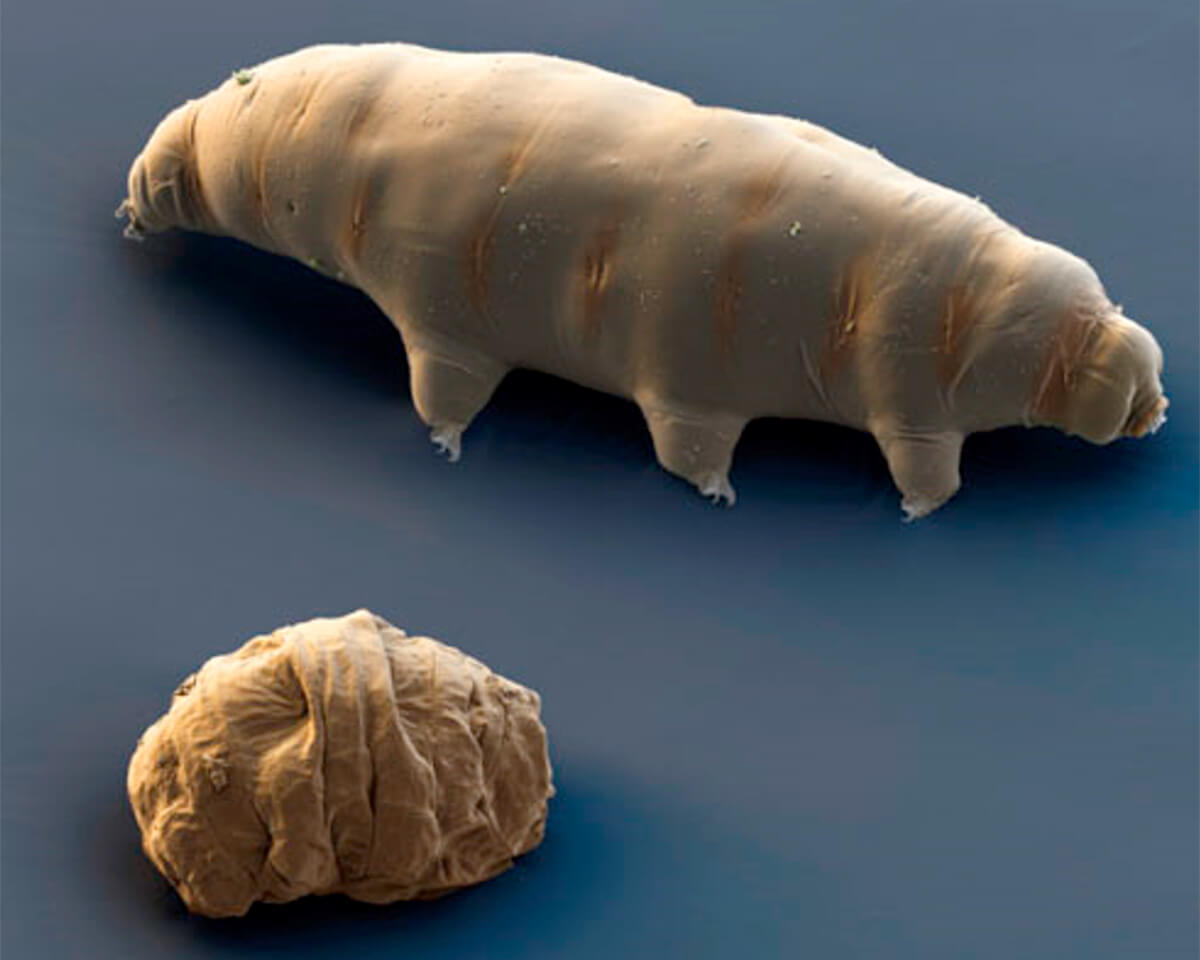
Anhydrobiosis
In response to an extremely dry environment, the tardigrade simply dries up. Anhydrobiosis is very common among many small organisms and plants, simply because there are so many environments on earth that experience regular, sometimes extreme droughts. Once sufficient humidity returns, the plant or organism rehydrates and returns to its normal state. It is believed the earliest forms of tardigrade were aquatic. At some point certain species ventured onto land and developed really excellent abilities to survive dry conditions, which has allowed them to thrive in terrestrial environments ever since.
There appears to be a collection of mechanisms that many species use to prevent the fatal damage that would otherwise occur with extreme desiccation. To do so requires protecting key proteins inside cells, and the DNA inside cell nuclei.
For the former, this includes the creation of protective sugars – trehalose in organisms, sucrose in plants – and the deployment of intrinsically disordered proteins (IDPs). Most cellular proteins can only perform their functions if they are in their properly folded forms. Water within the cells allows them to keep these forms, but once dehydration occurs, proteins will begin to lose their shape, eventually becoming irreparably damaged. As tardigrades undergo anhydrobiosis, they produce IDPs which fill the spaces vacated by the water, eventually forming a highly viscous glass-like substance that protects cellular proteins as the cells undergo a ~70% reduction in volume.
For the latter, a protein unique to tardigrades has been identified, coined Dsup, short for damage suppressor, which appears to protect the DNA and is probably the key to tardigrades’ extreme survivability. In eukaryotes, something called chromatin forms an envelope around a cell’s DNA. It appears that the Dsup, using electrostatic attraction and actually altering its molecular shape to fit tightly, forms a high energy cloud around the chromatin envelope, and protects tardigrade DNA from damage.
On another sidenote, the use of IDP’s in a number of applications is being researched. For example, it may be possible to create dehydrated vaccines held in IDP’s. This would greatly extend vaccine shelf life, and perhaps allow a slow release in the body, as the IDP-encased vaccines would gradually rehydrate by design, thus eliminating the need for boosters. In general, the many mechanisms species use to achieve a tun state could be very valuable, especially in medicine where the possibility of keeping organs, cells, fluids and other vital components of life in a dormant state and ready to be deployed when needed would be a tremendous step forward.
Additionally, there are other, lesser understood tun mechanisms that are actively being researched, which some species of tardigrade may use, including defense mechanisms against reactive oxygen species and xenobiotics (dioxins, biphenyls and other external pollutants concentrated by desiccation), heat shock proteins (proteins which help repair and protect regular proteins damaged by many extreme conditions including desiccation), and fatty acids and polyamines which appear to replace some of the functions of the missing water.
Cryobiosis
This is the tardigrade’s response to extremely low temperatures. Again, the tardigrade is far from alone when it comes to being able to withstand extremely cold temperatures. However, very few other species, if any, are able to withstand the -273K cold (absolute zero) that the tardigrade can. As with other cold temperature species, it does this by only allowing the development of ice crystals in parts of its body that will not be damaged by this, and by limiting the size of these ice crystals.
Chemobiosis and osmobiosis
Supposedly all five known forms of cryptobiosis are present in at least some tardigrade species, but these two are less common than the three covered in the previous sections.
Osmobiosis is the crytpobiotic response to osmotic pressure. In the case of tardigrades, presumably this would be the tendency for their internal fluids to exit through their cell membranes and exoskeletons when in a high salinity fluid environment. Perhaps this could be viewed as a subset of anhydrobiosis, but that is speculation on my part.
Chemobiosis involves the adoption of a reduced metabolic state in response to toxins in the external environment, typically high concentrations of dissolved metals. The jury is still out on whether tardigrades are actually capable of chemobiosis, or if they are just resilient. For example, (Hygum, et al., 2017) conclude that while the tardigrade species they studied showed higher than expected resistances to copper toxicity, most notably via the development of cysts, further study was required to determine whether this toxicity actually induced chemobiosis.
Tardigrade locomotion
Tardigrades are among the smallest creatures to possess legs and walk. Scientists are particularly interested in this as they believe tardigrade legs have not changed much over the millenia, and therefore represent the ancestral form of panarthropod legs. In other words, by studying tardigrade leg physiology and movement, they gain an understanding of what arthropod legs and movement evolved from, providing insight to a vast number of current insect species.
There is a key difference between how walking vertebrates and arthropods achieve different speeds of locomotion. A good vertebrate example to use is the horse – the gait and centre of gravity movement are entirely different between a walk, a trot, and a gallop. Humans are the same – the biomechanics of walking are quite different than those of running. Insects on the other hand only speed up or slow down their leg movements as needed, with the biomechanic details identical at any speed, save for which legs are moved at any given time. In this respect tardigrades fall into the insect camp, which gives a hint that their leg physiologies share a common ancestor. When a tardigrade moves slowly, it lifts one leg at a time; when it moves fast, it lifts 2 legs on one side and 1 on the other in unison, then alternates sides in this fashion.
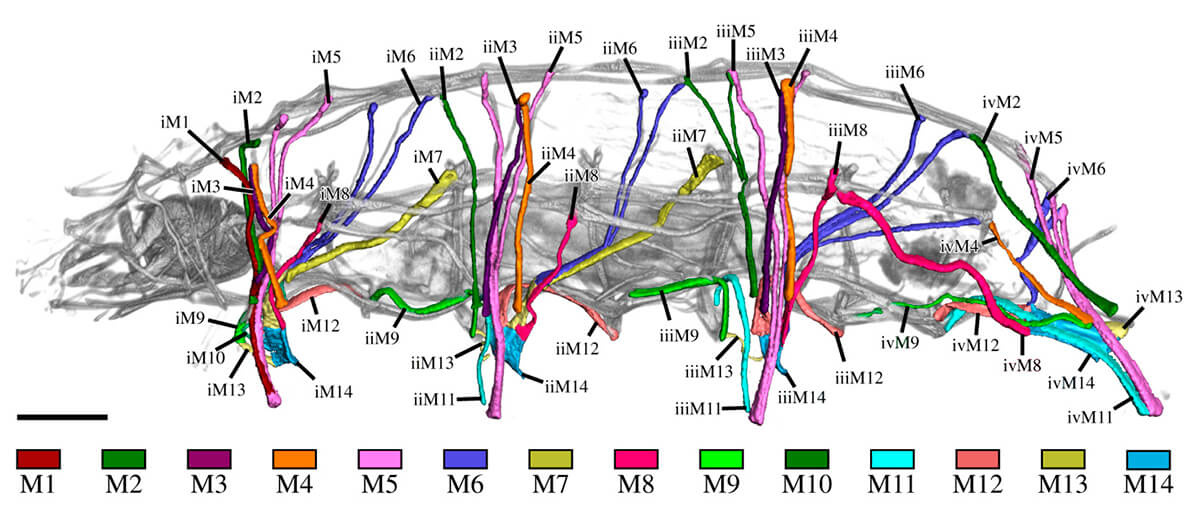
A paper by (Gross & Mayer, 2019) provides a glimpse at the level of sophistication possible in these kinds of studies. Figure 5 is from this paper and shows what’s possible with modern tools. In this case electron microscopy, confocal-laser scanning microscopy (CLSM), and fluorescent labelling of filamentous actin in muscle fibres are combined to produce stunningly accurate and detailed graphical descriptions of tardigrade leg muscles.
Scientific musings
Working on this article has me pondering the challenges involved in popular science writing. Ideally articles like this bridge the gap between scientific literature and everyday life, probably an important function…at least with topics like vaccines, perhaps not tardigrades. Yet there are several pitfalls along the way, not the least of which is how easy it is to misinterpret complex, dense, jargon-ridden peer reviewed papers. For example, a key concept in the (Gross & Mayer, 2019) paper is the investigation of serial homologies of tardigrade leg muscles. But what exactly do they mean by “serial homologies”? The Encyclopedia Britannica defines homology in biology as, “similarity of the structure, physiology, or development of different species of organisms based upon their descent from a common evolutionary ancestor” (Encyclopedia Britannica, 2022). Based on this, one might think they are looking at tardigrades to understand the evolutionary development of panarthropod leg musculature from its primitive tardigrade form to modern arthropod variations. In fact, they are using the words serial and homology in an entirely different way – they are referring to serial embryonic development, which in zoology can be referred to as metameric segmentation, with structures such as legs being developed in series, from anterior to posterior. That’s just one tiny example; any scientific paper might contain dozens of such conundrums which require concentrated thought and research to correctly understand.
A shortcut to avoid the difficulty of understanding key research papers is to go to derivative sources, such as non-peer reviewed articles, other popular science articles, or one of my favourite sources, Wikipedia. The risk here is that errors and misunderstandings abound. I came across several examples of this in reading about tardigrades. For example, almost every popular science article highlights their ability to survive in extreme conditions. One gets a picture of these cute little “bears” trundling around in hydrothermal vents, sand dunes, glaciers, even the moon for heaven’s sake! However, what almost every source fails to mention is that tardigrades only survive in these conditions while in their cryptobiotic tun state. That’s a key distinction.
One also gets the impression that tardigrades have evolved to survive all of these extreme conditions. In fact, they most likely evolved to survive extremely dry conditions, and high and low temperatures. The ability to survive intense pressure, high levels of radiation, lack of oxygen, etc. is a side benefit. Once in tun, they are almost indestructible and able to survive all those things, but that doesn’t mean tun evolved in response to each of those conditions.
Look at tardigrades yourself
It’s fairly easy to find tardigrades since they live almost everywhere. I’m planning on buying a cellphone mount microscope (many available online for as little as $20) and trying the following this summer.
- Find a clump of moss or lichen
- Soak it in a shallow dish in distilled or rain water for 3-24 hours
- Remove excess water
- Shake or squeeze the clump over a transparent dish to catch the water trapped in the clump
- View the water with a magnifying glass and identify that there are tardigrades
- Use a micropipette to transfer some tardigrades onto a slide, to view under a microscope
Quantum entanglement
Which finally brings us to quantum entanglement. Let me start off by saying that I long ago gave up trying to understand the leading edge of physics – I find concepts such as string theory impossible to understand – and I’ve steered clear of quantum entanglement. However, in order to close off this article I’ve given it a go! I found a suitable “for dummies” explanation at (Astrogeekz, 2018) which I’ll summarize here.
The term quantum denotes that we are talking about atomic scale and smaller. The concept of entanglement is related to wave-particle duality. This property was first discovered with light, that its behaviour is both wave-like and particle-like, something we should all remember from highschool physics. This is also true at the subatomic level. For example, an electron’s orbit around an atom’s nucleus is wavelike – it is everywhere as described by a probability or density function. However, as soon as it is measured, it behaves like a particle, i.e. it is in only one place.
Now, here is one example of quantum entanglement, which as you’ll see displays a similar type of duality. If a photon is sent into an atom, the electric field around the nucleus causes the photon to split into two particles, an electron and a positron. Note that a photon has zero energy and zero spin. As dictated by the law of conservation, the combined charge of the two particles formed by the photon split is zero, but by the same principle net spin must also be zero. This means that if the spin of the electron is up, then the spin of the positron is down, and vice versa. In fact, the two particles exist in a dual state, simultaneously being both spin-up and spin-down. However, as soon as they are observed, this dual existence ceases to exists – if the electron is observed to be spin-up, then the positron is immediately spin-down, or vice versa. The observation does not create a cause and effect reaction, the particles behave as two parts of a whole, even if far apart. In fact, even if the positron was a trillion miles away from the electron being observed, it would still simultaneously assume the opposite spin from its partner upon observation. That is quantum entanglement. This was something Einstein and other leading physicists were uncomfortable with. He dismissed it as, “spooky action at a distance,” but its existence has been confirmed and in fact is beginning to be commercially exploited.
Which brings us to tardigrades. Quantum entanglement is now used to create quantum computers, whose basic unit, similar to the 0 or 1 bit of conventional computers, is a dual state 0 AND 1 qubit. These systems only work at extremely low temperatures, as close to absolute zero as possible. So some researchers had the idea, since tardigrades in tun state are known to be able to survive such low temperatures, to try entangling a tardigrade with two quantum entangled qubits.
They took a tun state tardigrade and placed it on one (0/1) qubit, and then noted that its entangled, tardigrade-free qubit partner’s spin frequency dropped. They saw this as proof of “entanglement”, and they uploaded a not yet peer reviewed version of their paper to arXiv. Serious physicists immediately heaped scorn on their conclusions. The gist of the criticisms, I believe, is that while the insertion of the tardigrade affected the entangled pair of qubits, the tardigrade itself was not entangled – it did not achieve a state of entanglement itself, it was still the same little tardigrade in tun. A piece of dust, or Limburger cheese, or whatever, could have the same effect.
The quantum entangled tardigrade story however took off, with popular science writers around the world seizing on this latest opportunity to burnish the tardigrade’s rock star status. That is perhaps another pitfall in popular science writing, that even the researchers themselves at time succumb to the desire to hype their work. Anyway, while the quantum entangled tardigrade story turned out to be a letdown, at least it motivated me to look further into these fascinating little creatures.
Share This Column