Besides the Gulf Stream, ocean currents are likely out-of-sight, out-of-mind for most people. I don’t even recall ocean currents being part of the geophysics undergrad curriculum, even though they should definitely fall under physics of the earth, with temperature and density physics explaining their dynamics. The following article is a very brief summary of the topic. Ocean currents are somewhat fractal in nature, with the tiniest little flows and eddies being similar to the very largest ones that span entire oceans, the only difference being scale. I will mainly focus on the macro current systems.
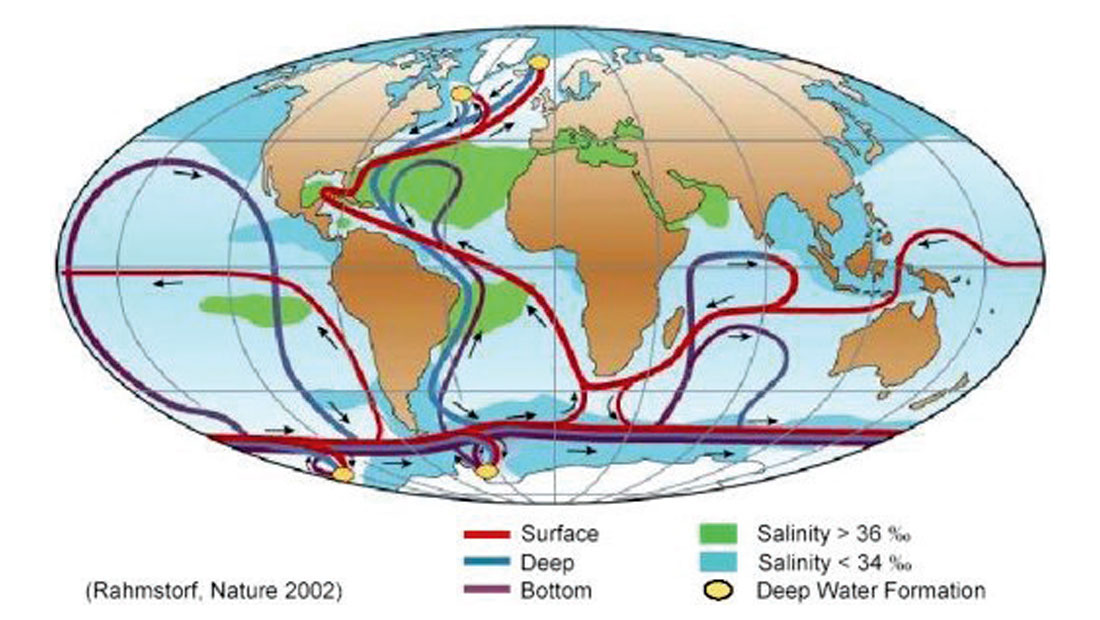
The world’s major ocean currents can be viewed as one system, colloquially known as the global conveyor belt, or more technically as meridional overturning circulation (MOC) or thermohaline circulation. The latter two terms hint at what drives the system: ocean density gradients created by variations in salinity and temperature cause distinct water masses to move. However, other factors also come into play, including winds and tides, although these are more related to local, more variable currents. Figure 1 neatly captures the basics of the system, and highlights three of its notable features – it is a closed, continuous system, it involves warm and cold currents, and it moves in all three dimensions including both deep and shallow water routes. The time a water molecule would take to complete one “lap” is on the order of ~2000 years. The conveyor belt is fundamentally driven by sinking dense water in the polar regions. In the Norwegian Sea, relentless cold winds cause evaporation from the relatively warm water (warm due to the Gulf Stream), which both cools the water and makes it denser (evaporation leaves the salt behind). This mass of cold, heavy water sinks quickly to the bottom, and then flows through established sea floor channels between Greenland, Iceland, the Faroes and Britain, and moving south descends to the Atlantic abyssal plain where it slowly glides towards Antarctica. Interestingly, the waters of the Arctic Ocean are hemmed in on all sides and do not contribute significantly to the deep Atlantic flow, and not at all to the Pacific (see Figure 2).
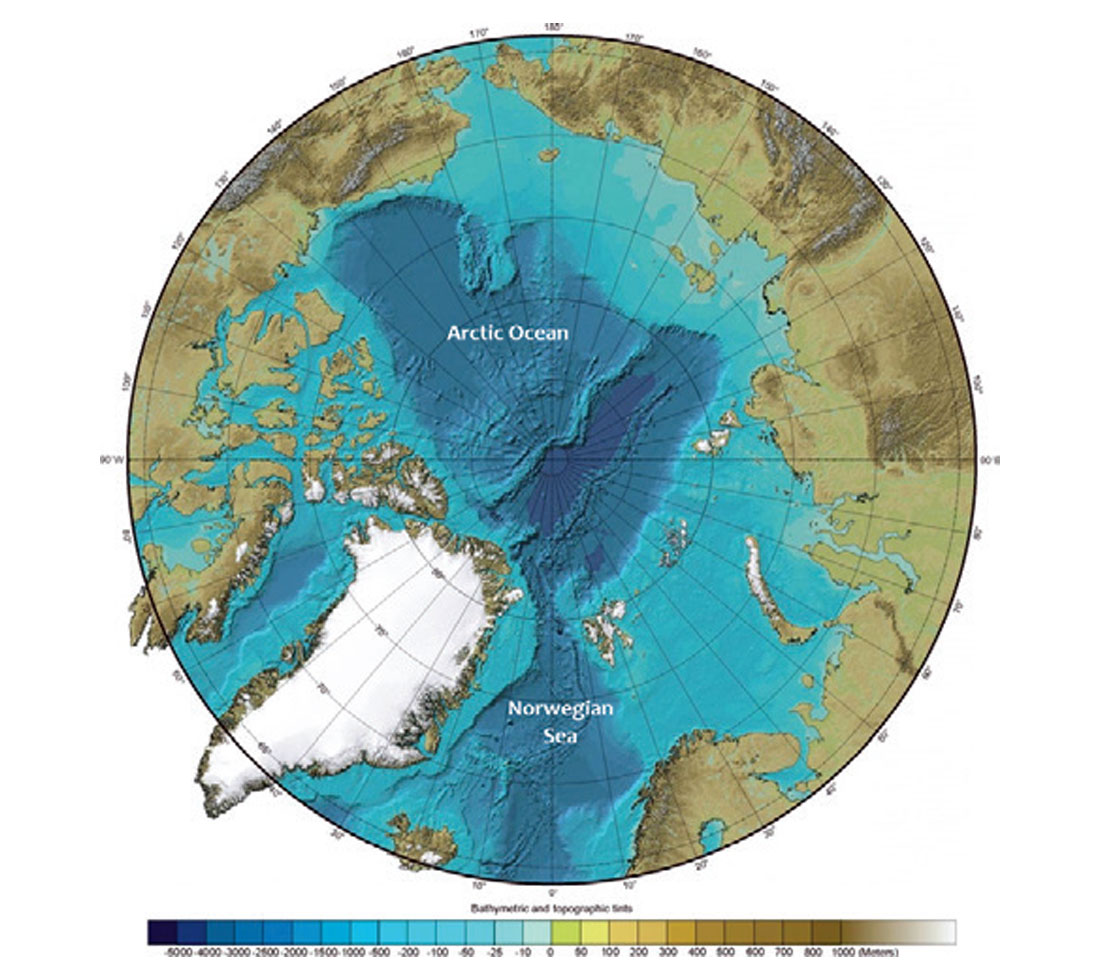
In the Antarctic the process is similar but different – cold winds from the frigid centre blow outwards over the continental shelf ice, especially in the Ross and Weddell Seas (Figure 3). This creates a cycle that results in cold, high salinity water masses. The winds blow chunks of newly formed ice out to sea, leaving areas of open water which experience enhanced evaporation and therefore dropping temperatures and increasing salinity. New ice is also continually forming, which in itself also raises salinity, since pockets of fresher water will freeze before saltier water (I covered the physics of eutectic freezing points in the context of alcoholic beverages in a previous article (Kuhn, Practical science, 2015); additionally, any pockets of saltier liquid brine locked in the ice matrix will tend to melt downwards and escape.
The cold water travelling south from the Norwegian Sea continues all the way to Antarctica, where it forms a circular, clockwise, deep water flow around that continent; it is joined by the deep water formed above along the Antarctic shelf. The deep water formed in the Weddell Sea flow joins this westward flow and then mainly splits off to travel north into the Pacific; similarly, the water dropping down from the Ross Sea flows mostly east and then north into the Indian Ocean. However, some water also flows directly north into the Atlantic, but because it is so cold it actually flows underneath the slightly warmer water moving down from the north. I’m assuming at some point it starts to warm enough to rise and curl around to join the southward flow.
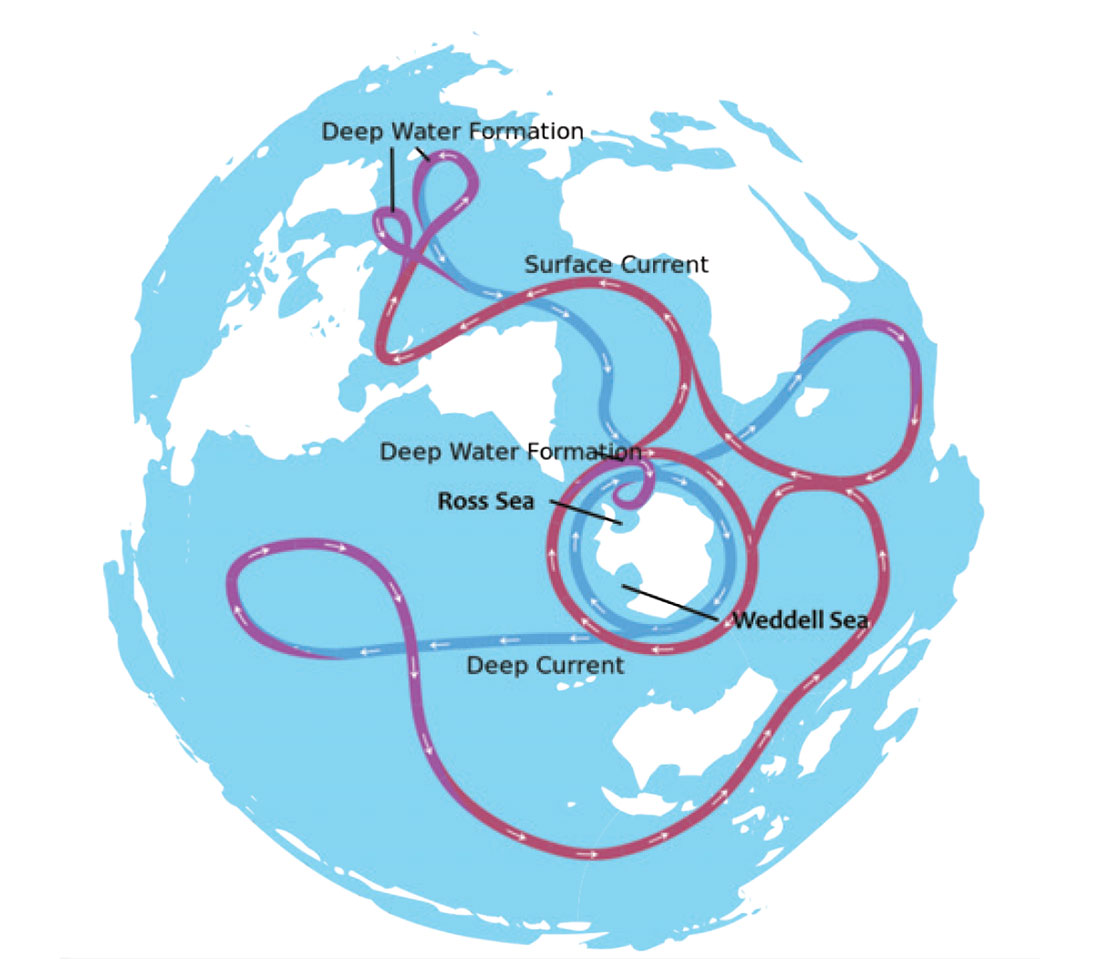
That covers the cold deep water part of the story, but we all know that what goes down must come back up, and what cools down must warm up again, or is it the other way around… It actually gets very interesting, as Figures 1 and 3 show. All this cold water sinking down and moving into the deepest parts of the Atlantic, Pacific and Indian Oceans must be displacing other water, and so where does that water go? The cold water sinking occurs in the two relatively small locations discussed (Norwegian Sea and around Antarctica), but the opposing upwelling is diffuse and occurs over such large areas that it is actually quite hard to detect; it is an area of active scientific debate. The use of silicon levels, isotopic ratios, and various other chemical tracers have helped locate areas of upwelling. Figures 1 and 3 reflect the general consensus on this point with upwelling shown in the North Pacific and Indian Ocean, but some scientists believe a significant amount of upwelling occurs in the Southern Ocean as well.
The Pacific and Indian Ocean upwellings are different, but their causes are connected. The huge volume of cold, dense, high salinity water leaving the Atlantic results in its surface being at a slightly lower level than the Pacific, and the Pacific has lower salinity as well. This means that the warmer and lighter Pacific surface waters flow down towards the Atlantic, continually replacing the water exiting the Atlantic at depth. This explains the large westward flow of water from the Pacific (Figure 1), through the Indonesian islands, across the Indian Ocean, diagonally across the South Atlantic into the Caribbean and the Gulf of Mexico, and across the North Atlantic and back to the Norwegian Sea, to complete the cycle. Note that the final leg northeast across the Atlantic is helped along by the Gulf Stream, which is really a separate surface current. Going back to the leg across the Indian Ocean, here the flow of warm water draws the deep northward flowing cold water up to the surface, where it warms and joins the western flow – this is the Indian Ocean upwelling.
The Pacific upwelling is much more gradual and diffuse. Besides the two (and possibly three) specific areas of upwelling, there are countless other areas around the globe where upwelling occurs, and so I will briefly touch on the mechanisms involved. There are five main types of upwelling – coastal, mid-ocean, eddy-associated, topography- associated, and diffusive – and all of these are created by a combination of three main factors – wind, Ekman transport, and the Coriolis effect.
Along coasts it is typical to experience convectional winds, and these create water movement. The Coriolis effect manifests itself in an apparent movement component at 90 degrees to the direction of the wind, to the right of the winds in the north hemisphere, the left in the southern. For an explanation of the Coriolis effect, see (Kuhn, Weather Maps, 2009). If this movement of water, known as Ekman transport, is away from the coast, then the departure of that shallow warm water draws in deeper cold water. There are five major currents of this type in the world: the Humboldt off Peru, the Benguela off southern Africa, the Canary off northwestern Africa and the obviously located California and Somali currents. These currents are all critical to the ecosystems they support because of the deep nutrient-rich water they bring to the surface, and major commercial fisheries depend on them. Further, major ocean currents of all types play an integral role in the earth’s weather systems.
Any currents in ocean basins, whether wind-driven or part of the MOC system, will be forced to the surface by topographical obstacles. A classic example of this is in the Southern Ocean where a large west wind driven current builds up speed and volume with no obstacles from South America until it hits Antarctica, where large upwelling occurs. Storm systems such as cyclones can churn up the water enough to cause upwelling. Slow and gentle upwelling occurs in oceans as warm and cold water masses gradually intermingle and exchange heat, helped along by more subtle doses of the above mentioned factors.
As with most of the topics I cover, I am left with a feeling of having inadequately covered a large, complex and fascinating topic, and must leave it to readers to further look into the details. But the takeaway overview for me, with which I will bore my family and unsuspecting victims at parties is that there is a huge closed loop of ocean currents that circle the world’s oceans. Cold salty water in the Norwegian Sea sinks and moves south to Antarctica where it is joined by similarly sinking water. The cold water spins around Antarctica, and flows mainly north into the Pacific and Indian Oceans where it warms and upwells. The warm, low salinity Pacific water is sucked back via the Indian Ocean (where it picks up more warm water) all the way through the Atlantic to the Norwegian Sea, where the cycle begins again.
Share This Column