While some discoveries in astronomy rely on light that has crossed the observable universe to reach us, another epochal endeavor is unfolding much closer to our home planet: the cataloging of Solar System objects that could actually hit the Earth.
Asteroids and comet impacts threaten our planet, but what kind of danger depends on the timescale we consider. Given enough time, impacts on an apocalyptic scale are inevitable. The Chicxulub impact wiped out the dinosaurs by triggering massive tsunamis and wildfires, and by plunging the Earth into an ‘impact winter’ produced by a pall of stratospheric dust that shut out the Sun. The Shoemaker-Levy 9 impacts on Jupiter in 1994 released the explosive energy of about a million hydrogen bombs, and bruised the giant planet’s cloud tops with slowly fading ‘impact scars’ larger than planet Earth (Figure 1).
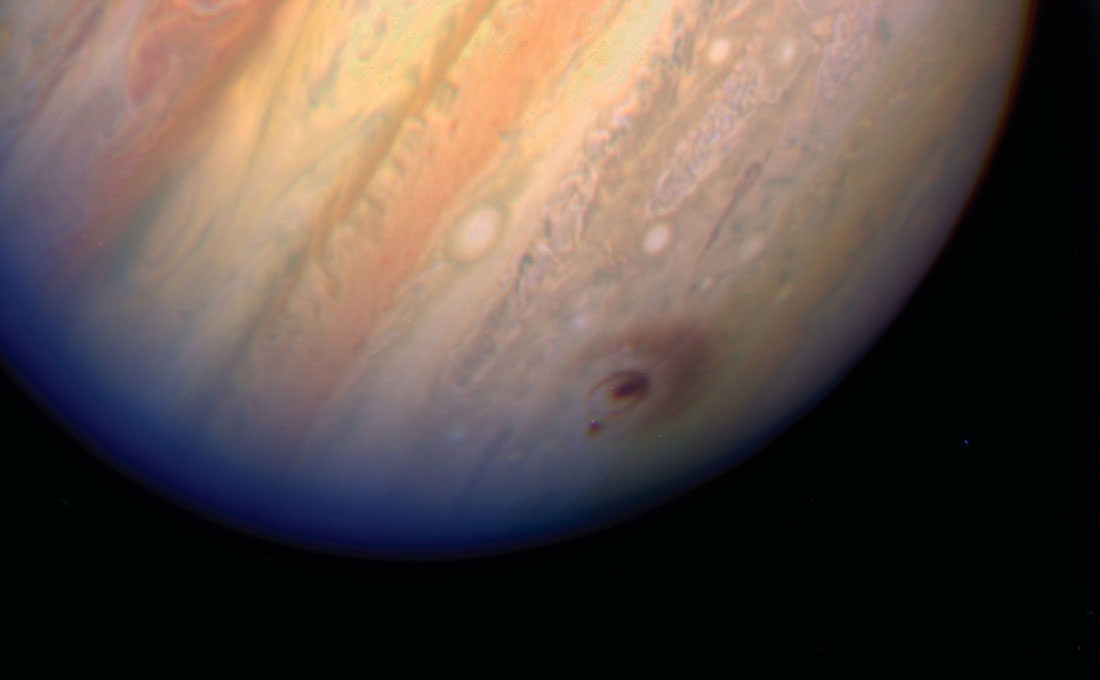
On Earth, on a human timescale of centuries or millennia, events like these are extremely unlikely. It has been 65 million years since the Chicxulub impact, and the mean interval between impacts of comparable scale appears to be even longer. Jupiter’s size, strong gravity, and location nearer to the typical orbits of comets and asteroids make impactors such as Shoemaker-Levy 9 thousands of times more common there than on Earth. It is objects smaller than the dinosaur-destroying impactor (estimated at 10 km) or the largest fragments of Shoemaker-Levy 9 (2 km) that pose the most realistic near-term threat to Earth.
Success of NEO Surveys
The objects that pose a danger to our planet reside not in the main asteroid belt between Mars and Jupiter, but rather in a much smaller population of asteroids called the near-Earth objects, or NEOs. Unlike main belt asteroids, NEO orbits are unstable, evolving chaotically due to gravitational effects from close encounters with Earth and the other inner planets. The NEO population would have vanished soon after the birth of the Solar System if it were not replenished by a trickle of asteroids and cometary objects that depart their formerly-stable orbits and move inward due to subtle interactions with sunlight and the gravity of the giant planets. The timescale for this process is millions of years, so only the current members of the NEO population are of immediate concern in protecting Earth. Although chaotic on a longer timescale, NEO orbits are predictable over decades to centuries. To protect our planet, we must find all the NEOs, accurately predict their orbits, and take action if one is found that will impact Earth.
The human quest to discover NEOs had no well-defined beginning, but a major breakthrough came on October 27, 1989 when David Rabinowitz of the Spacewatch survey made the first discovery of an NEO using a charge-coupled device (CCD). CCDs were a then-new imaging technology that offered better sensitivity and more accurate measurements than photographic plates. In 1992, a NASA-organized workshop comprising two dozen experts in various asteroid-related disciplines produced the historic Spaceguard Survey report (January 25, 1992; edited by David Morrison), which noted that cataloging NEOs had become technologically feasible but would take centuries to complete at then-current rates. The report recommended increased efforts (collectively and informally referred to as the Spaceguard Survey) to find 90% of all NEOs larger than 1 km within 25 years. This endeavor was characterized as a reasonable ‘insurance policy’ against global catastrophe.
By the year 2011, CCDs of ever-increasing size and efficiency had revolutionized astronomy, 8,000 NEOs had been discovered (911 of them larger then 1 km), and NASA announced that the original Spaceguard goal had been surpassed: only an estimated 7% of all NEOs larger than 1 km remained to be discovered. Meanwhile, in 2005 the US Congress had passed the George E. Brown, Jr. NEO Survey Act, directing NASA to catalog 90% of all NEOs down to 140 meters in size by 2020. Asteroids this size are dangerous (a 140-meter impactor could devastate a region the size of small nation), and detecting them had already become feasible – thus, the 2005 directive could be considered an unusual instance of good sense on the part of the US Congress. The goal will probably not be met until a few years after 2020, but surveys continue to discover NEOs at increasing rates (Figure 2). More than 13,000 NEOs are now known, and none appears to be on an orbit that will impact Earth in the next century.
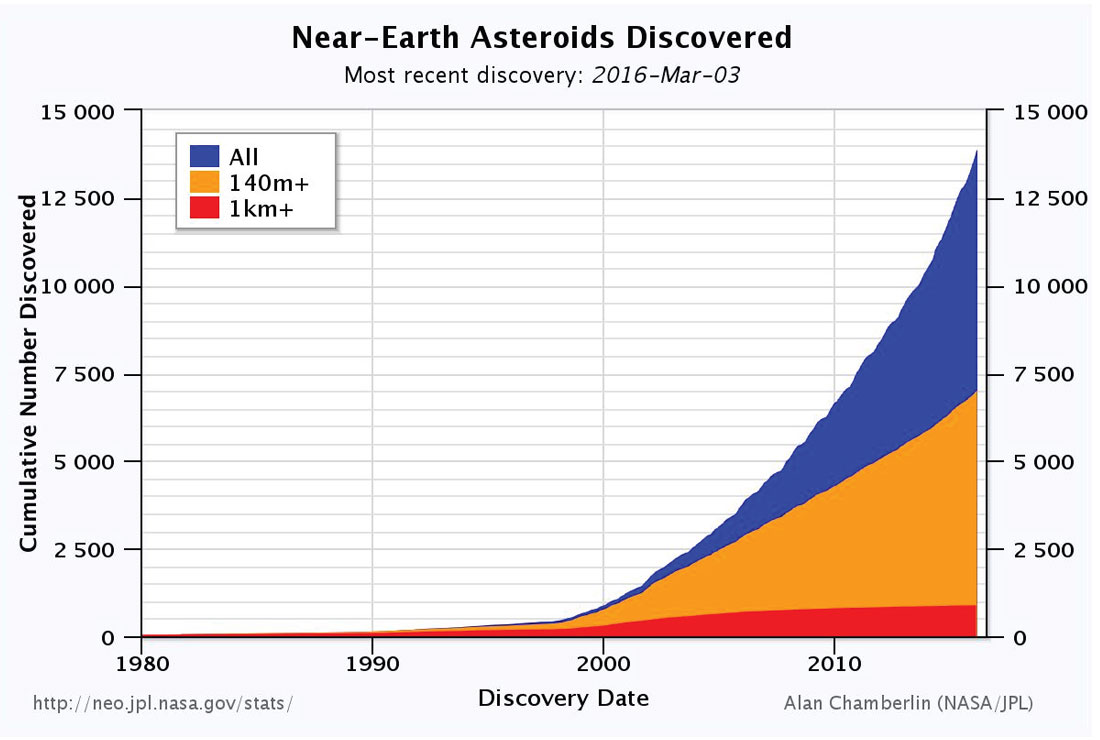
If an asteroid larger than 140 meters is discovered on an orbit that will impact Earth, we will likely have decades to centuries of warning. This will be long enough to deflect the object using one of several ingenious methods that have been considered. These include painting or shadowing the asteroid to change its interaction with sunlight, modifying its orbit with a ‘gravitational tractor’, and ‘nudging’ it with a nuclear explosion a few tens of meters above its surface. If less warning were available (from a few months to a few years), the more challenging option of burying a nuclear charge beneath the asteroid’s surface would still deliver the required, larger momentum change. Alternatively, for all but the largest plausible impactors a bigger but still-feasible buried nuclear explosion could disrupt the whole asteroid, leaving only an expanding cloud of boulders. Although some of these fragments might still strike Earth – especially if the destroyed asteroid had been within a month of impact – they would be far less dangerous than the original, intact object.
The Gap in Our Defense
Thus far, the outlook appears rosy. Asteroid surveys have greatly reduced the risk of an unknown asteroid causing widespread devastation to our planet, and viable deflection strategies exist for any future impactors discovered with sufficient warning. A few nagging worries remain. A long-period comet could strike with far less warning than an ordinary NEO, but such objects are rare. More seriously, existing surveys provide very limited protection against NEOs smaller than 140 meters, which could still produce local destruction. On February 15, 2013, a dramatic event warned us not to discount the danger of these tiny objects.
On that frosty Siberian morning in Chelyabinsk, Russia, the sky unexpectedly lit up with a meteor as bright as the newly-risen sun (Figure 3). Its brief flight ended with a spectacular airburst at an altitude of about 30 km. Two minutes later, while thousands of eyewitnesses were still admiring the dramatic cloud-trail lingering in the sky, a deafening shock wave from the high-altitude detonation hit the ground. Windows shattered in thousands of buildings over a region inhabited by more than a million people. Hundreds sought medical aid for injuries from the broken glass. One building – a poorly constructed warehouse – actually collapsed. Although no one was killed, the damage totalled millions of dollars. The asteroid responsible was only 20 meters in size, and arrived on a shallow trajectory that caused it to explode at higher altitude and reduced the damage on the ground. An object only slightly larger (30-50 meters) on a steep trajectory could level a city – and such impacts are far more frequent than those of the 1-km objects that would cause a global catastrophe.
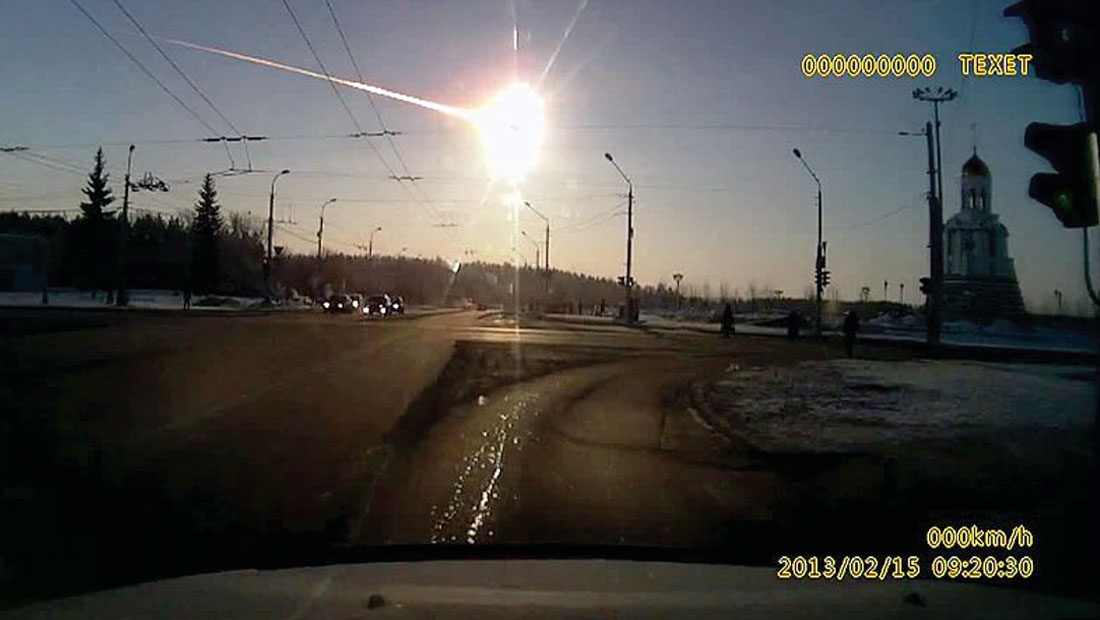
The paradigm of planetary defense that is proving so successful with larger NEOs – cataloging and predicting their orbits for decades in advance – simply will not work for small asteroids in the 20-50 meter range. Such objects are often discovered by current NEO surveys, but they remain bright enough to be observed only for a few days during close passes by Earth. After this – and long before data sufficient for long-term orbit prediction have been acquired – they recede and are lost in the immense volume of the Solar System. Decades may pass before a particular object comes close enough to be spotted again, and it is impossible to be sure that the next close pass won’t be an impact.
A New Paradigm for Small Impactors
A remarkable series of events five years before the Chelyabinsk impact – and involving an even smaller asteroid – points the way to protecting Earth from NEOs too small for long-term orbit prediction. On October 6, 2008, Richard Kowalski of the Catalina Sky Survey – one of the most productive efforts to discover and catalog NEOs – was searching for asteroids using the 1.5 meter telescope on Mt. Lemmon, Arizona. His CCD images showed a new object moving across the sky at a rate typical of NEOs, but showing acceleration and curvature in its track that indicated it might be unusually close to Earth. He reported it promptly to the International Astronomical Union’s Minor Planet Center (MPC), making his data immediately available to others. This prompted astronomers at three other observatories around the globe to make (and immediately report) further observations of the interesting object. Based on 26 measurements spanning only 8.5 hours, the MPC designated the object 2008 TC3 and officially announced that it would impact the Earth, but that it was small (about 4 meters) and would explode harmlessly in the upper atmosphere.
Why was an impact prediction possible using just hours of data? Because 2008 TC3 would hit the Earth not decades in the future, but on the following day! The MPC announcement triggered hundreds of additional observations from dozens of observatories, which sufficed to predict the time and location of the impact – a remote desert region of Sudan – with an accuracy of only a few seconds and a few kilometers. Several satellites and other instruments recorded 2008 TC3’s high-altitude explosion right on schedule and in the predicted area. A scientific expedition led by Peter Jenniskens of the SETI Institute traveled to Sudan two months later and collected numerous fragments of the meteorite, which had dark colors that made them easy to spot against the bright desert sand (Figure 4).
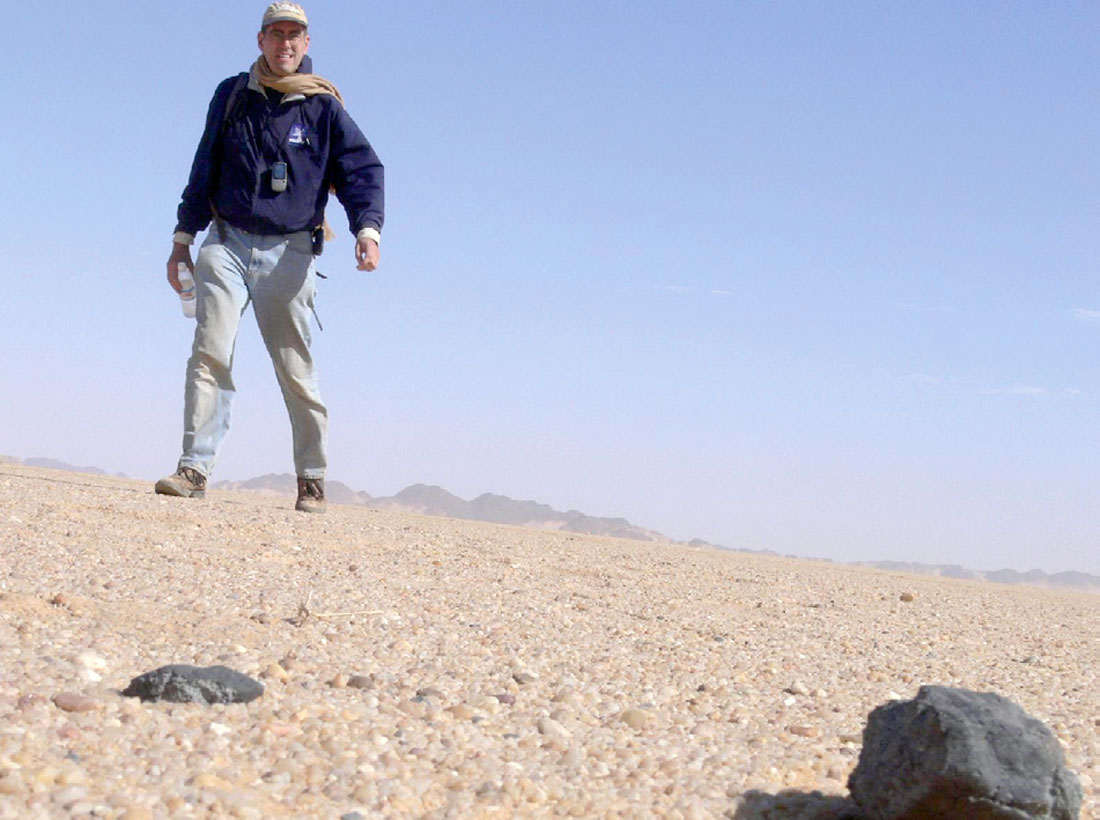
The message of 2008 TC3 is that we can treat the impact hazard from these smaller NEOs as we do another class of natural disasters: hurricanes. We cannot prevent or deflect a hurricane, but we can predict it and recommend that people evacuate or take shelter. For this, we do not need decades of warning – a few days is enough. The time and location of an asteroid impact can be predicted far more accurately than those of a hurricane, and a valuable post-impact scientific expedition can be organized in parallel with the evacuation of imperiled civilians.
The discovery of 2008 TC3, however, was in some ways a lucky fluke. Current surveys are not well optimized to provide the required warning for small-asteroid impacts. They are designed instead to complete the still-important task of long-term orbital prediction for larger NEOs that could cause a global catastrophe. To do this, they make sensitive observations of one region of the sky per night, taking many nights to cover the whole sky. A 50-meter NEO, incoming for impact, could easily appear in a region of the sky that no survey was currently observing. The surveys would look there within a few days or weeks, but by then the object could already have struck the Earth. This would have happened on a smaller scale with 2008 TC3 if Richard Kowalski had pointed his telescope in a different direction. We need a new type of survey that looks everywhere, every night.
The Asteroid Terrestrial-Impact Last Alert System
The Asteroid Terrestrial-Impact Last Alert System (ATLAS) is intended to do exactly that, using custom-designed 0.5 meter telescopes with 100 megapixel CCD imagers to cover huge areas of sky. ATLAS began survey operations with its first telescope in October 2015, near the summit of Haleakala, a dormant volcano on the Hawaiian island of Maui (Figure 5). A second telescope is currently being completed in Colorado, and will soon be installed on the upper slopes of Mauna Loa, another volcano on the neighboring island of Hawaii. Ultimately at least four telescopes are planned, in different locations around the globe to cover the sky as thoroughly as possible.
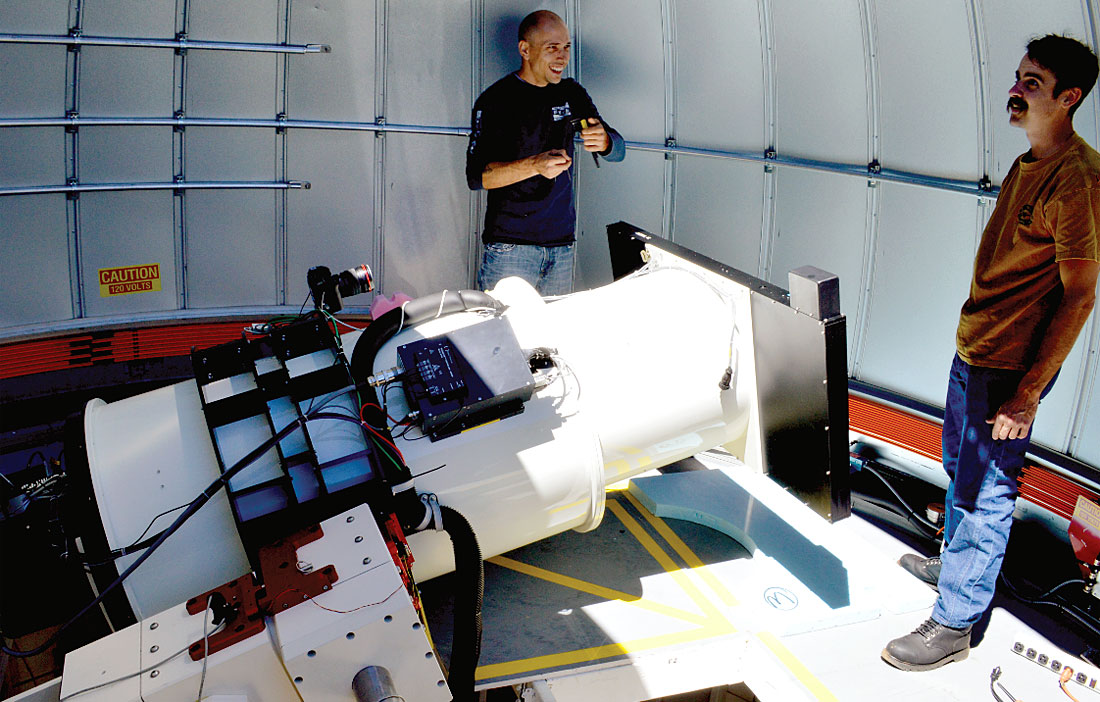
The ATLAS imagers have a field of view of 29 square degrees with a pixel size of 1.86 arcseconds.
At present, Telescope 1 is taking four images of each field per night, with an exposure time of 30 seconds and mean interval of about 90 minutes between the first and last exposure of a given field. With these parameters, Telescope 1 covers about one quarter of the accessible sky each night – far more than any other survey of comparable sensitivity – and scans the entire accessible sky every four nights. Current automated detection software finds asteroids moving up to ten degrees per day (1500 arcseconds per hour), which are not significantly trailed in the ATLAS images.
Telescope 1 is operating efficiently and mostly robotically, with thousands of asteroid measurements reported each night to the MPC. Nevertheless, the ATLAS survey is still effectively in commissioning mode, with many aspects of the software and even hardware undergoing continual improvement. Telescope 1 was delivered with a preliminary corrector lens that does not function as well as a perfect corrector would, reducing its single-exposure sensitivity by a factor of three (from magnitude 20 to about 18.7). This corrector will be replaced within the next few months. Even sooner, the nightly sky coverage for Telescope 1 will improve through the reduction of observing overheads (time to re-point the telescope, rotate the dome, and readout the CCD). Streak-detection software will be deployed to find asteroids with angular velocities up to at least the 360 degree/day motions of the geostationary satellites (the software will include automatic orbital analysis to reject the artificial satellites themselves).
With the replacement of the Telescope 1 corrector lens and the installation of Telescope 2 in mid-2016, ATLAS will cover more than half the accessible sky each night. Sites far from the Hawaiian islands will be selected for Telescopes 3 and 4, in order to provide weather diversity and (ideally) access to the far southern sky that cannot be seen from Hawaii. One of these telescopes will likely be sited in South Africa, while multiple locations are being considered for the other. By the installation of ATLAS telescope 4, the survey will cover the entire sky every night.
Despite its current sensitivity limitations, ATLAS Telescope 1 has discovered seven NEOs and one comet in its first five months of surveying. A recent discovery illustrates the system’s unique capability. On March 2, 2016 – just two days ago at this writing – ATLAS detected a bright, fast-moving NEO within 40 degrees of the north celestial pole (Figure 6). Other surveys had not found it because they were not scanning the right part of the sky. The data were submitted to the MPC, triggering follow-up observations from three other observatories on March 3. Based on these, the MPC designated the object as 2016 EH1, and calculated a size of 100 m (for an albedo of 15%) and a distance of only 0.04 astronomical units (6 million km). The object is now heading away from Earth and poses no danger in the foreseeable future.
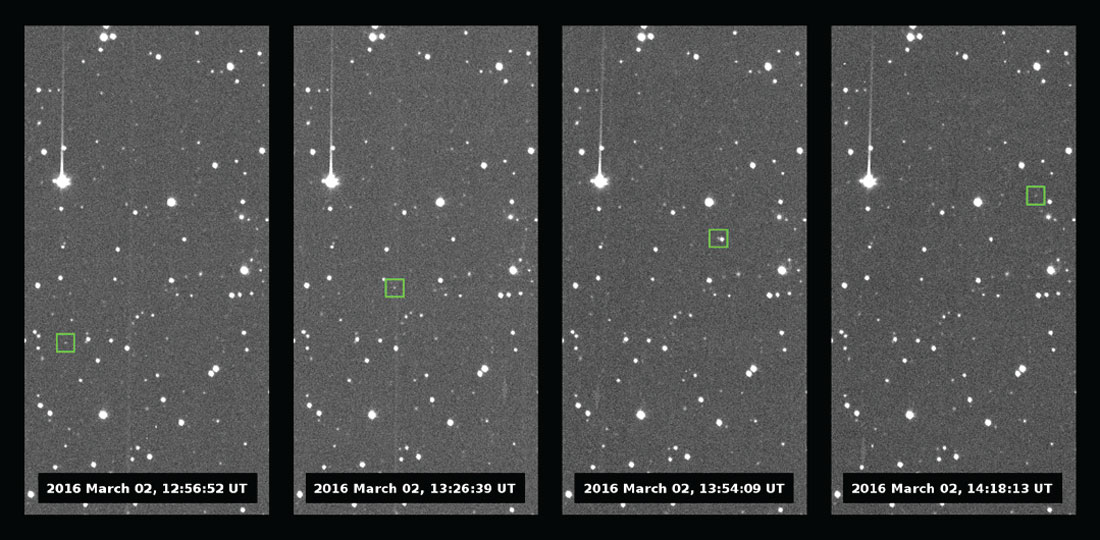
If 2016 EH1 had instead been inbound for impact, it would have struck Earth about 10 days after its discovery, causing local devastation on a scale not seen since the Tunguska event in 1908, when an explosion believed to have been caused by a similar-sized impactor leveled 2000 square kilometers of uninhabited Siberian forest. Orbital calculations sufficient to begin evacuations would have been available several days before impact, and depending on the target zone millions of lives might have been saved. When Telescope 1 receives an improved corrector lens and Telescope 2 begins operations, ATLAS will find NEOs at ten times its current rate, protecting humanity from small asteroids such as 2016 EH1 that no other survey is optimized to discover.
Join the Conversation
Interested in starting, or contributing to a conversation about an article or issue of the RECORDER? Join our CSEG LinkedIn Group.
Share This Article