Introduction
In the last twenty years land seismic acquisition has changed in many ways. The standard seismic crew twenty years ago was likely to be a 96-channel, 16 bit analogue cable system. Digitizing was done in the recorder and digital data written to tape. Field filters and gain settings were switched manually. The observer was entirely responsible for data quality, running the crew and writing the observer logs (OB’s). A typical geophone array consisted of 9, 14 Hz elements wired in a 3 X 3 series-parallel configuration. Winter bases were commonly used in frozen conditions. The data were recorded on 9- track tapes at 1600 bpi on 10-inch reels (~20 Mbytes). The programs were mostly acquired using wheeled or tracked vehicles. Most of the lines were cleared by literally bulldozing a line through the trees. Surveying was done using line-of-sight theodolites (or EDM’s). HSE standards were far less rigorous than they are today and the first 3D’s were just being shot in Canada using multiple systems slaved together.
Today’s crew looks quite a bit different. Health, Safety and the Environment (HSE) issues are of utmost importance. For example, Veritas uses onsite medics (one step below a paramedic), daily safety meetings, a stop, think and go program, near-miss/hazard reporting, HSE advisors, all terrain vehicle limiters (Quad limiters) and a VIMS (Veritas Integrity Management System) system to track all safety related information. Bag runners and GPS have eliminated the need for ground intervention for bag pick-up and deployment. Survey crews utilize a combination of conventional EDM’s, GPS, under-canopy GPS and inertial systems that allow for the reduction of timber costs and, of course, produce less impact on the environment. Seismic drills are much smaller than they used to be. Two pick heli-drills (as opposed to three) help to reduce helicopter time and costs as well as enabling them to set up in more difficult areas with less risk to people and the environment. Low Impact Seismic (LIS) drills help reduce the environmental impact as compared to the larger and wider drills.
The biggest change to the seismic crew however, has occurred on the recording side. Heli-portable seismic crews are very common these days since a large channel crew can acquire data faster and cheaper by using helicopters, especially in rugged environments. Slashing is minimized to the point where it is difficult to see the line from the air. If a line is cut, chances are it will be mulched and only 3m wide. Very large channel crews (~7000 ground stations) are common as is 3D. Digitization happens in a distributed sense, data transmission is digital and 24 bit technology is the norm. Data are recorded onto flash memory or high density, yet small tapes. Computers run the acquisition system through preplanned scripts, most of the system quality control (QC) is done automatically and OB’s are automatically generated. However, one of the most significant changes to the recording crew will be the routine recording of multicomponent data. By recording the three mutually orthogonal components, one can better understand the rock properties of the subsurface.
While the concept of multicomponent seismic has been around for many years, cost and quality concerns have limited its use in conventional exploration. Recent technological advances, such as reductions in sensor size and weight, while maintaining or improving performance and reliability, are helping to address these problems. Silicon accelerometers have been available for over a decade, yet only recently has technology allowed these miniature accelerometers to be manufactured with a noise performance compatible with seismic requirements. Both Input/Output (VectorSeis) and Sercel (DSU) have adopted this technology in the design of unique micro-machined digital accelerometers specifically targeting the seismic acquisition industry. The new VectorSeis® digital sensor and the Digital Seismic Unit (DSU) provide several advantages over coil analogue geophones.
The focus of this paper will be on the new VectorSeis® multicomponent digital sensor. The working principals and spectral characteristics of the I/O digital sensor, and data comparisons with conventional 1C and 3C geophones will be presented. This new technology and associated recording equipment are also helping to improve the cost structure of multicomponent surveys. Acquisition issues such as operational efficiencies, recording with single sensor vs. field arrays, and leveling/orientation requirements will also be discussed.
Digital Seismic Sensor
Input/Output’s, digital sensor has two principal components, a micro-machined silicon accelerometer with a small inertial mass, suspended by miniature springs and a custom designed, mixed-signal ASIC control chip. Force rebalanced feedback operation provides a 24-bit digital output directly from the sensor unit obviating the need for A/D converters in the recording system. The acceleration-proportional output shows a flat transfer- and phase-response from very low frequencies up to 500Hz (Figures 1). Implementation of the digital sensor is in an orthogonal 3-component arrangement forming the core of the VectorSeis® recording system. The data are acquired on a VectorSeis Remote Seismic Recorder (VRSR) platform, 6 x 3C stations per box. Sensor design started as early as 1986 with the first prototype field tests in 1998 following extensive laboratory testing. A significant development milestone was achieved with the rerecording of the PanCanadian (now EnCana), Blackfoot 3C-3D survey in 1999 with Veritas DGC. 2003 will see the regular operation of a 4000 station, multicomponent pilot crew, again operated by Veritas in N. America.
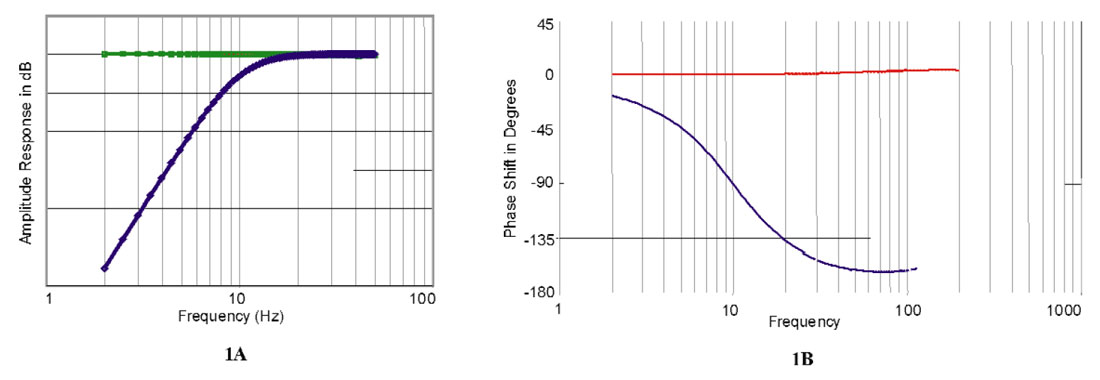
Operational Considerations (Acquisition)
For standard P-wave exploration, the analogue coil geophone has served the industry well for over 70 years. They are relatively inexpensive, rugged and reliable and they allow for flexible array designs. On the other hand, the natural resonance (for example, 10 Hz) limits the recorded signal fidelity at lower frequencies. Furthermore, the advent of 24-bit recording, improved processing options and cost constraints have lessened the concern if not the need for careful array design. For multicomponent applications the geophone’s limitations become more noticeable. The vector fidelity and response of one vertical and two horizontally deployed coils is severely impacted unless the geophone is planted within a few degrees of perfectly level. Even then, vector fidelity is a concern, since the horizontal and vertical coils have significantly different response characteristics. The operational advantages of recording multicomponent data with these new purpose built multicomponent sensors are substantial; They require fewer connections and less cable so, the overall weight of the equipment is reduced The sensors do not have to be levelled in the field, since this can be corrected for in processing. Figure 2 illustrates the reduction in cable, weight, and number of connections as compared to standard 1C and 3C systems.
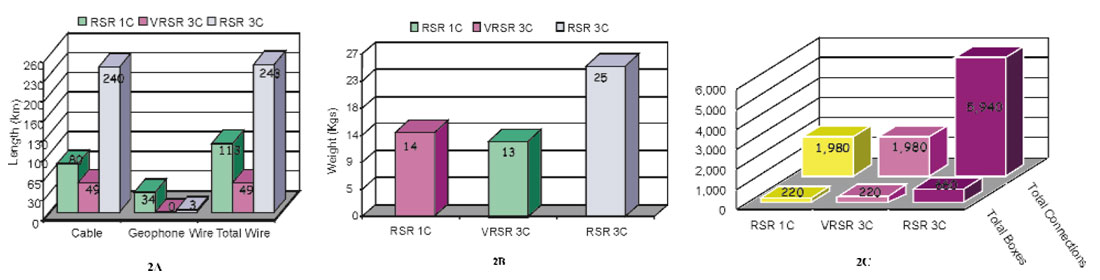
The ability of the sensor to work at all orientations increases the acquisition rate and improves coupling since the sensor is not adjusted for leveling purposes. The VRSR recording system utilizes a transcription process that separates the different components into individual files thus reducing processing time and uncertainty. The final result is accurate and affordable multicomponent acquisition.
Conventional P-Wave Data vs VectorSeis P-Wave Data
Veritas has acquired, processed and analyzed numerous programs with side-by-side comparisons of VectorSeis® sensors to conventional coil geophones. The comparisons have included climatic variations from -30 °C to 40 °C, geographical variations from Northern Canada to the US Gulf Coast, geological settings including carbonates and clastics at depth ranging from 100’s of meters to 4000 meters in both structurally complex and stratigraphically complex regimes. We have made single sensor comparisons, array versus single sensor comparisons and arrays in the field versus array forming in processing. We have considered the differences between recording in velocity units for the geophones versus acceleration units for the VectorSeis® data. We have also taken into account the geophone impulse response effects in these comparisons. Furthermore, we have investigated different source configurations in order to maximize the energy for converted-waves and compressional waves. And finally we have considered acquisition design configurations that are optimal for both the converted-waves and the compressional waves.
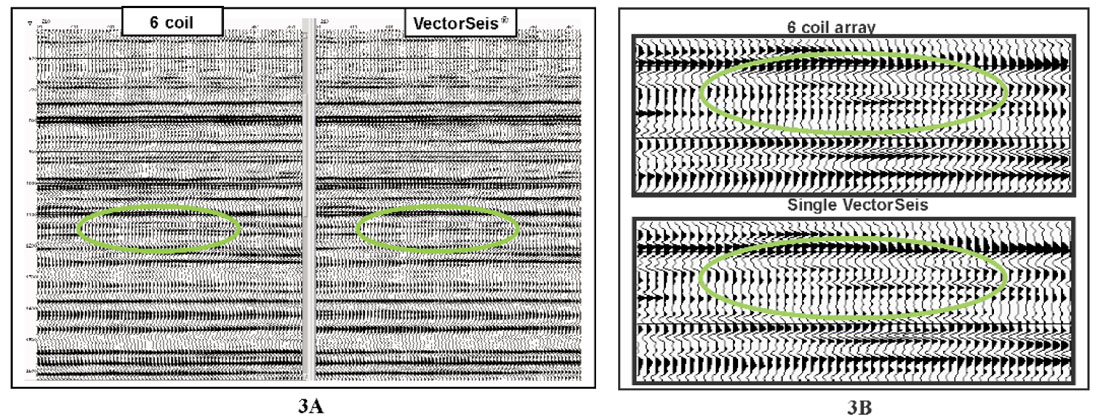
Figures 3-5 are side-by-side comparisons of vertical geophone array data versus single sensor VectorSeis® data. Figure 3 shows data that were acquired in SE Saskatchewan, Canada, Figure 4 shows data that were acquired in the Alberta Foothills of Western Canada and Figure 5 shows data that were acquired in the US Gulf Coast region. These examples clearly illustrate the similarity in sensitivity and data quality for conventional geophone arrays versus single sensor VectorSeis® measurements. However, this does not mean we are universally recommending replacing geophone arrays with single sensors. There will be environments where arrays are necessary. Furthermore, array forming in the field is not possible with digital sensors simply because each individual unit performs the digitization onboard. Independent of the efficacy of the ground roll attenuation, a field array always provides √n S/N improvement simply due to increased effective fold. With single sensor recording similar array forming, in fact more flexible adaptive array forming, can be applied during processing to get equivalent or better results. Even without such efforts, in most cases routine processing has produced remarkably similar results between 6-element coil array geophone data and single sensor VectorSeis® recording.
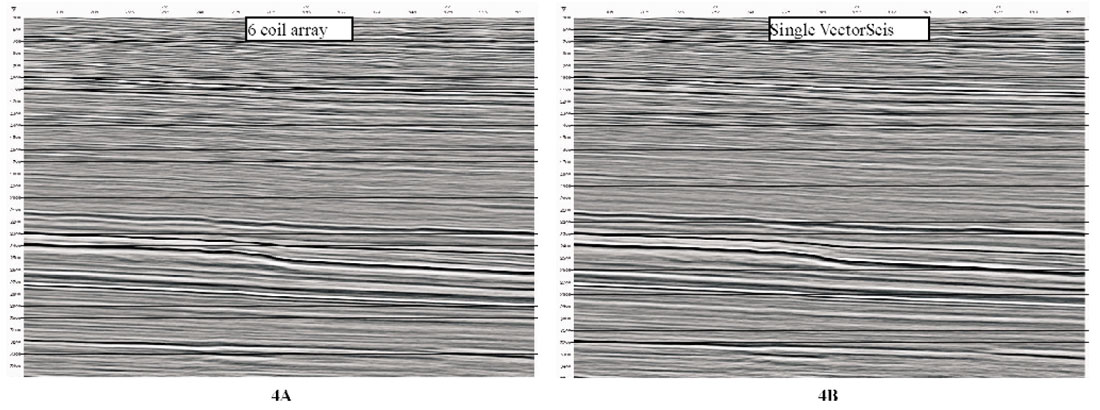
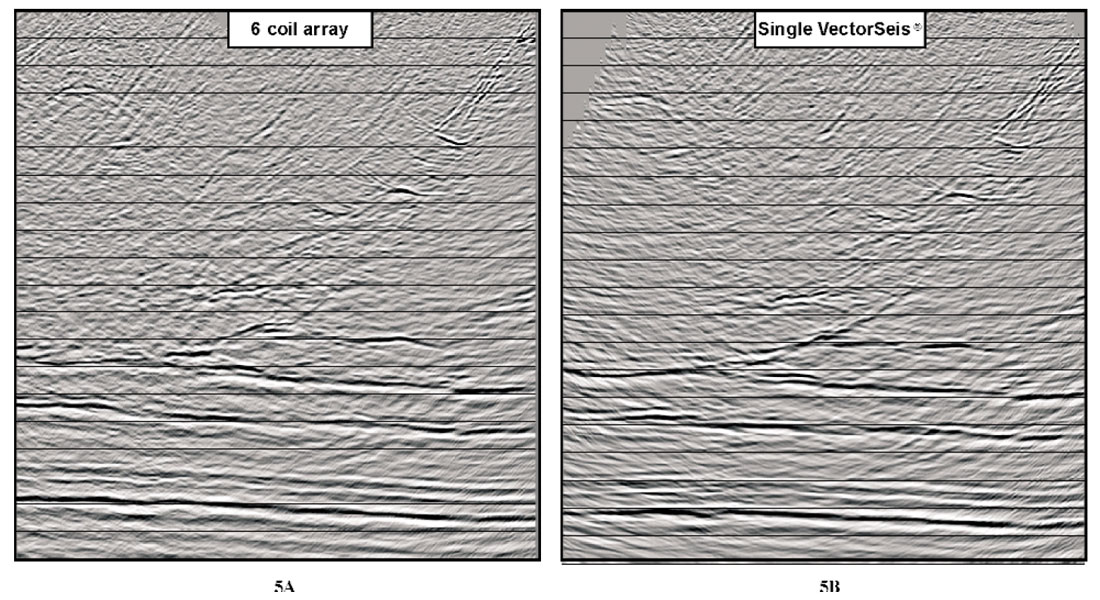
Converted-Wave Data Using VectorSeis®
Veritas have processed numerous converted-wave datasets that were acquired using VectorSeis®. In general, we are finding these converted-wave data are of better quality compared to conventional 3C data. We believe this is due to the many reasons listed above and in particular due to: the sensors ability to work at any orientation; the reduction in error due to wiring problems; fewer connection errors; separated components delivered from the field; improved vector fidelity and superior coupling. Furthermore, multicomponent recording has typically involved single sensors due to the added complexity of orienting and leveling conventional 3C geophones. Therefore, the single sensor versus geophone array issue is less significant than it is with P-wave data. Figures 6 and 7 show examples of converted-wave data that were acquired with VectorSeis®. Figure 6 shows a comparison of PS and PP data from SE Saskatchewan. The interpretation and further discussion associated with figures 3 and 6 can be found in the expanded abstracts of the CSEG annual convention (Kendall et al, 2002). Figure 7 illustrates the impressive converted-wave signal strength for a very deep target (4000 m) given a modest charge size (2.2 Kg at 20m) and relatively low fold (1500%).
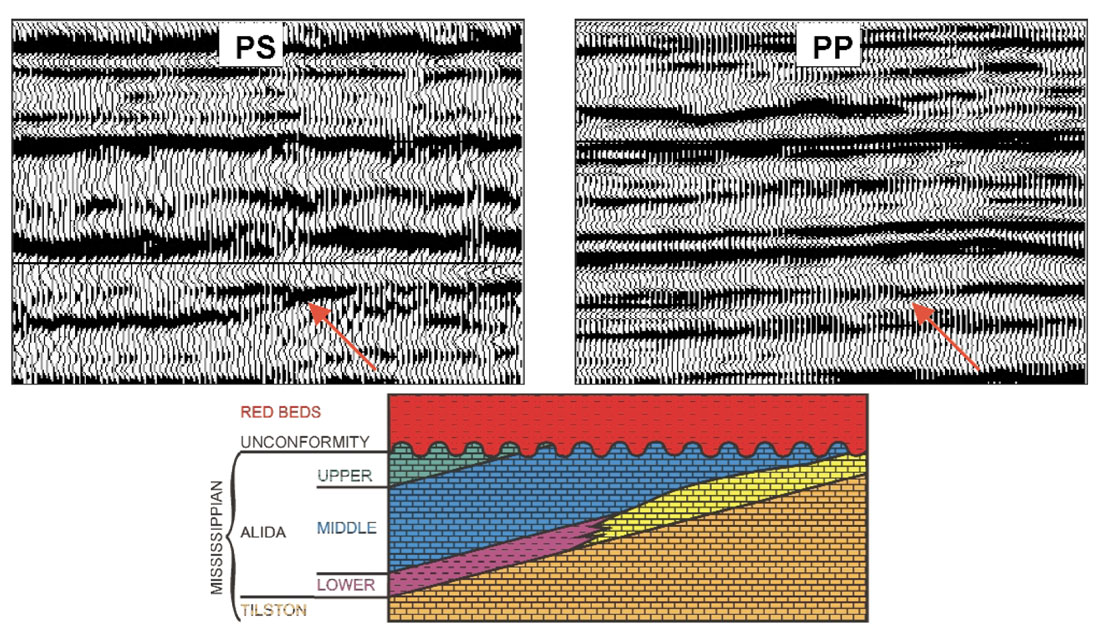
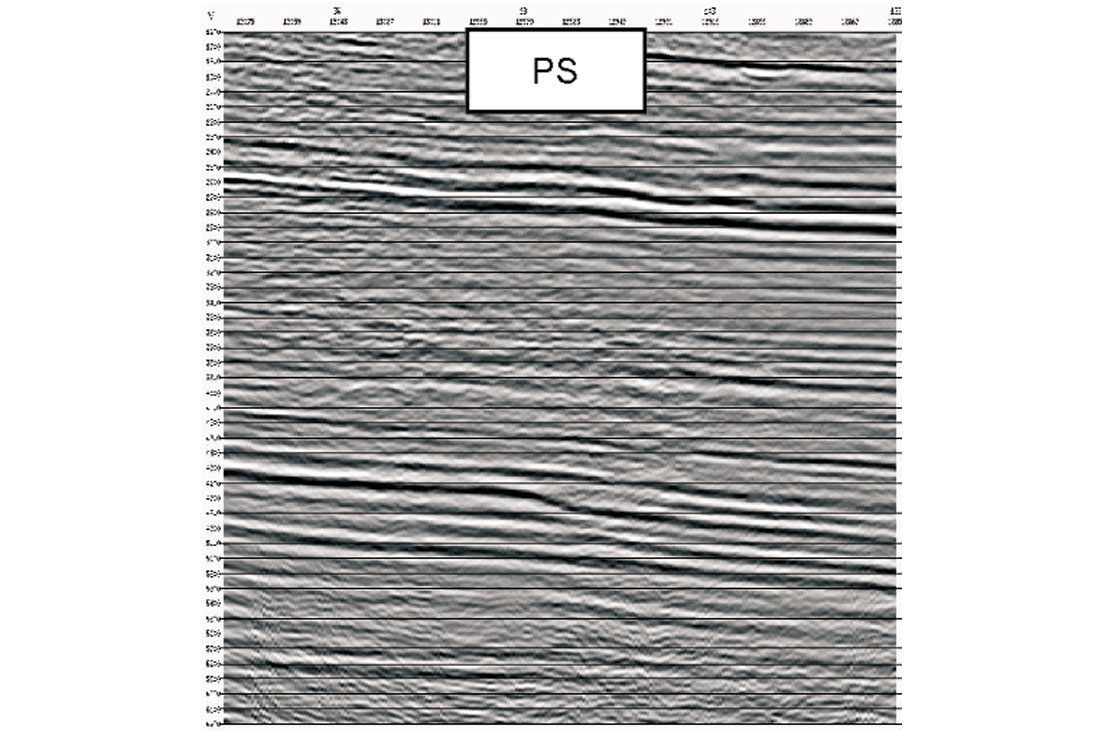
Conclusions
Many changes have occurred in the last twenty years with respect to land seismic acquisition. A typical land crew has seen changes in safety (HSE), surveying, seismic drills and the recording crew. One of the biggest changes we are experiencing now is the more frequent use of multicomponent-specific recording systems that take advantage of the latest advances in silicon accelerometers. The I/O VectorSeis® seismic sensor allows for more accurate and cost effective multicomponent acquisition due to reductions in weight, number of connections and the amount of cable. We have also shown, through example, the sensor performs as well as conventional geophone arrays in numerous geological and geographical environments. Furthermore, we have shown examples of the PS data that can be acquired with these new sensors.
Acknowledgements
The author would like to thank the following individuals for their valuable contributions: Roy Burnett, Howard Watt, Scott Cheadle, Wayne Nowry, Pete Scott and Placer Deguzemen from Veritas and Peter Maxwell, Doug Goble and Jon Tessman from Input/Output. The author would also like to thank Nexen, Petro-Canada and EnCana for the data examples.
Join the Conversation
Interested in starting, or contributing to a conversation about an article or issue of the RECORDER? Join our CSEG LinkedIn Group.
Share This Article