The Catalina Sky Survey (CSS) is a NASA-funded survey to help quantify the potential hazard of impacts by Near Earth Objects (NEOs). However, the survey images contain many other scientifically useful data.
Near Earth Objects
Near Earth Objects are defined as asteroids (or minor planets) and comets that come within 1.3 astronomical units (or ~194 million km) of the Sun. The vast majority of NEOs migrated from the main asteroid belt (MB) between Mars and Jupiter through collisions, close passes to planets, and radiation forces. The MB itself is a result of planetesimals unable to accrete into a planet due to gravitational perturbations by Jupiter.
In spite of the vast distances between objects orbiting the Sun, collisions in the Solar System are a fairly common and natural phenomenon of the accretion process that led to the formation of planets. One only has to look at the moon in a small telescope to see the results of impacts. Although the flux of terrestrial impactors is greatly reduced from the time of the Late Heavy Bombardment 4.1 – 3.8 billion years ago, we still see the evidence in such events as the 1908 Tunguska air burst, and the more recent 2013 Chelybinsk meteor. There are also many remnants of past terrestrial impacts scattered over the globe. The Earth sweeps up about 100 tons of mostly small micrometeroids each day. Our atmosphere protects us from most of the small NEOs that become meteors or Bolides, if bright (See Figure 1).
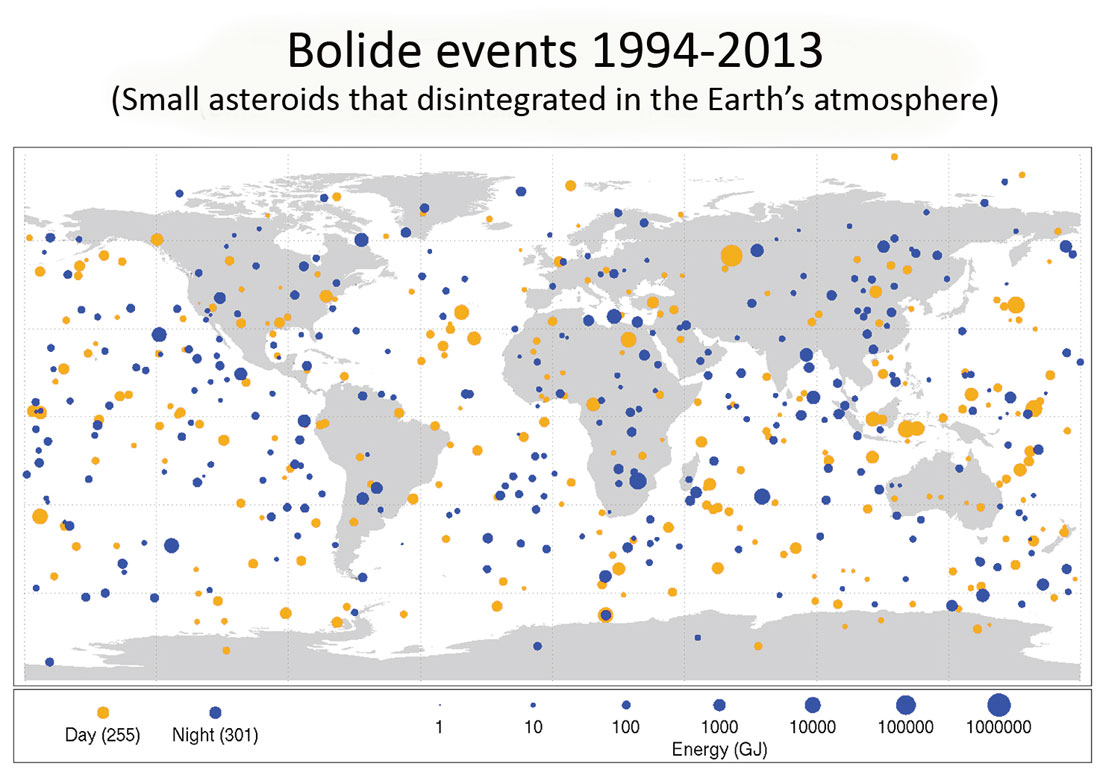
The KT extinction event
The iridium anomaly in the global Cretaceous-Tertiary (KT) boundary and identification of the associated Chicxulub impact crater (Hildebrand et al., 1991) was the “smoking gun” of this major extinction event. This served as an example of the importance of asteroid impact on the evolution of life on the Earth. Although the collision frequency is much lower than in the past, the question is not whether there will be future impacts, but when. Clearly, efforts to mitigate a future impact are contingent on a complete understanding of orbital geometry, and this begins with discovery.
Quantifying the present danger of terrestrial impacts
NASA’s NEO Observations Program (NEOO) is a result of a 1998 congressional directive to create a program (initially called Spaceguard) that would identify at least 90 percent of bodies 1 km or larger. Impacts from bodies of this size would be capable of global consequences, such as filling the atmosphere with ejecta debris for years and destroying the food chain.
By the late 1990s, computer power and sensitive solid state detectors had matured, and allowed for much more efficient discovery of NEOs. Given what little we knew about the numbers and size distribution of NEOs, it would have been irresponsible to not at least quantify the impact threat by exploring the NEO domain.
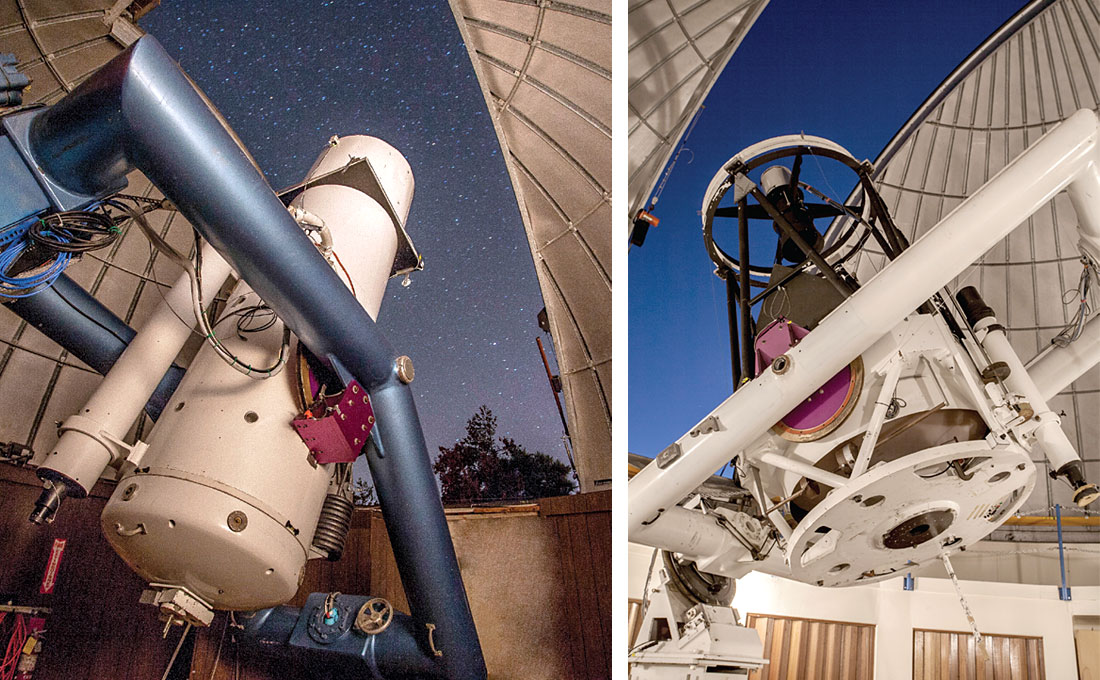
In 2005, a law was passed directing NASA to extend the search for NEOs to a subgroup called Potentially Hazardous Asteroids (PHAs), defined as NEOs that come as close to the Earth as ~9.7 million km and are 140 m or more in diameter. Such objects are particularly susceptible to future perturbations into Earth-intersecting orbits and could cause significant regional damage.
Repurposing little-used telescopes
The original 1998 NASA NEOO program did not have the resources to build a network of telescopes optimized to detect moving NEOs, so existing, but little-used telescopes were modified for this task. Since 1998, 15 different ground-based telescopes have been surveying the sky for various durations. Most were older telescopes requiring refurbishing and upgrades to be effective for this purpose.
For CSS, that meant computerizing and interfacing large-coupled device sensors (CCDs) to four old hand-slewed telescopes. In the Catalina Mountains north of Tucson are the 0.7-m wide field survey Catalina Schmidt (2002–present), the 1.5-m Mt. Lemmon narrow field survey telescope (2003-present), and the 1.0-m narrow field follow-up remotely controlled telescope (2014-present). Additionally, at the Siding Spring Observatory in Australia, we upgraded the 0.5-m wide field survey Schmidt (2003–2012) to cover the southern sky.
The CSS was the leader in discovering NEOs from 2005–2013, and will soon get an upgrade using the largest monolithic CCDs with 110 megapixels. In spite of all the comings and goings of NEO surveys, the discovery rate continues to rise.
The Wide-field Infrared Survey Explorer (WISE), launched in 2009, included a NEOWISE component to identify NEOs in its data, and because it was sensitive to the thermal infrared, provided essential information on the reflectivity of NEOs. Having used up its cryogen, it still surveys from orbit at shorter wavelengths.
How to find a NEO
NEOs are identified by their motion relative to background stars. CSS does this by taking four images of a standard star field separated by 10–15 minutes. Very sensitive and large charge coupled devices (CCDs) are capable, with 30-second exposures, of reaching stars a billion times fainter than is visible in a very dark sky by the naked eye. The images are corrected for instrumental effects, and by using reference field stars, the brightness and astrometric location of each “star” is determined. Software then generates catalogs of all the “stars” in the images and the four images are compared. All objects with the same coordinates are ignored, and the remaining objects are tested for linear motion. Those flagged by the software as having linear, consistent motion are flagged and presented to the observer to validate their reality. If the motion is similar to typical NEOs, the positional information is sent to the Minor Planet Center (MPC) where a preliminary orbit is calculated, and ephemeris generated for the benefit of follow-up observers (most are amateur astronomers) who try to extend the observed orbital arc. The original observer may schedule additional follow-up observations if the prospective NEO might be making a close pass. At CSS, the observer makes essential decisions in real time, resulting in some of the results described below.
NEO population
When the Spaceguard Survey started in 1998, there were about 500 known NEOs – now we know the orbits of over 14,000 NEOs. Nearly half have been discovered by CSS.
Because the optically fast CSS telescopes allow detection of small, nearby, rapidly moving NEOs that may escape detection because of trailing losses, NEOs as small as 1-m can be detected (Figure 3). Close approaches to the Earth happen several times a year, but there are no known PHAs with a high probability of impact over the next 100 years.
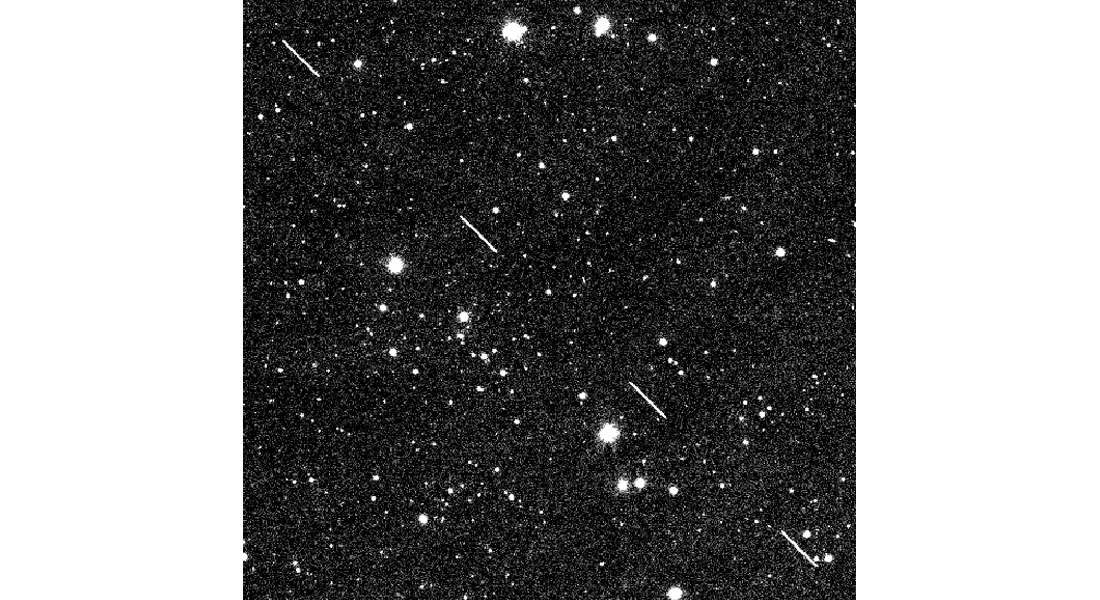
Recent results of modeling the size distribution indicate that NEOs orbiting interior to the Earth stand a good chance of becoming heated by the Sun to the extent that they break apart, leaving meteor streams.
Found along the way: temporarily “captured ”NEO
The NEO 2006 RH120 was discovered by the CSS in September 2006. It is only 2-4 meters across, but was in a temporary, loosely- Earthbound orbit. At first, it was thought to be a piece of space junk, but the measured effect of solar radiation over the four three-month orbits was more consistent with a rocky natural asteroid. It was ejected back into a heliocentric orbit after passing its fourth perigee in June 2007.
This sort of encounter is expected every few years.
Incoming!
CSS has discovered three small NEOs before they impacted the Earth. The first one was 2008 TC3, which was discovered on October 6, 2008 by CSS when just beyond the orbit of the moon, and 20 hours before entering the Earth’s atmosphere.
Alerted by the software indicating NEO-like motion, CSS observer Richard Kowalski, working in real-time, followed observing protocol by making a second set of observations and sending the coordinates to the Minor Planet Center, which (among others) determined that it was on an impacting trajectory. The word got out to the amateur follow-up community, and over 570 telescopic observations were made over the 19 hours before it entered the Earth’s shadow and was no longer observable. Precise orbit determination at JPL predicted an entry velocity of 12.4 km/sec at a slant angle of 20 degrees over northern Sudan not long before local sunrise. The real-time reporting of our CSS observations was critical in being able to obtain data that determined that it was a 4-m-sized object of irregular shape rotating in an energized non-principal axis mode (wobbling like a poorly thrown football) with principal periods of 49 and 97 seconds. Spectra (550–800nm) from the 4.2-m William Hershel telescope in the Canary Islands were consistent with C- or M-type asteroids. This was the first time an incoming impactor was observed in space prior to impact. There was a visible dust trail cloud shortly after entry (See Figures 4 and 5).
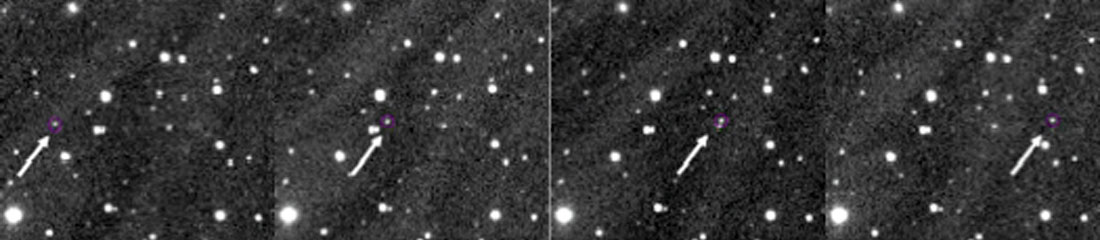
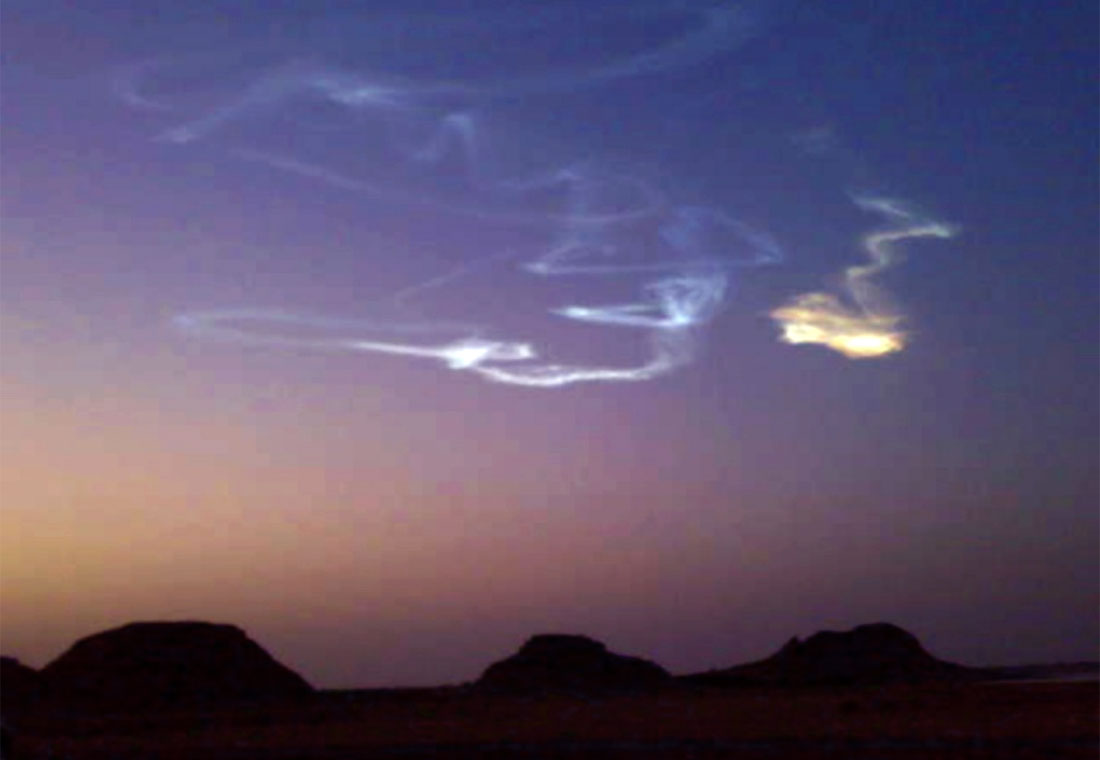
The small size of 2008 TC3 led most to assume that it would burn up upon atmospheric entry. However, two months later a party led by P. Jenniskins (SETI/NAS Ames) and M. Shaddad (University of Khartoum) systematically searched the ground track of 2008 TC3 and recovered over five kilograms of the meteorites named Almahata Sitta (station six – on the Wadi Halfa to Khartoum railroad). So this event was, in effect, a very inexpensive NEO sample mission (See Figure 6 to Figure 8).
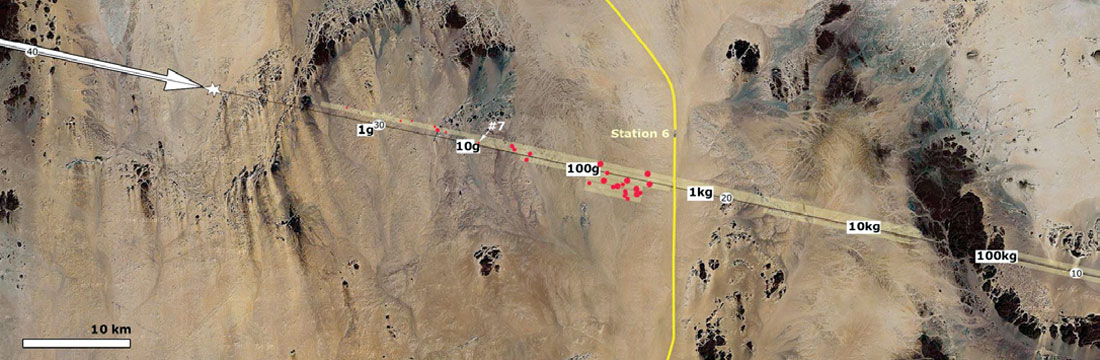
Almahata Sitta meteorites are classified as rather rare, anomalous, polymict ureilite (achondrite) with large carbonaceous grains. Specimens have multiple distinct lithologies ranging from ordinary chondrite to bencubinnite, suggesting a complex collision history among different compositions. Amino acids have also been found.
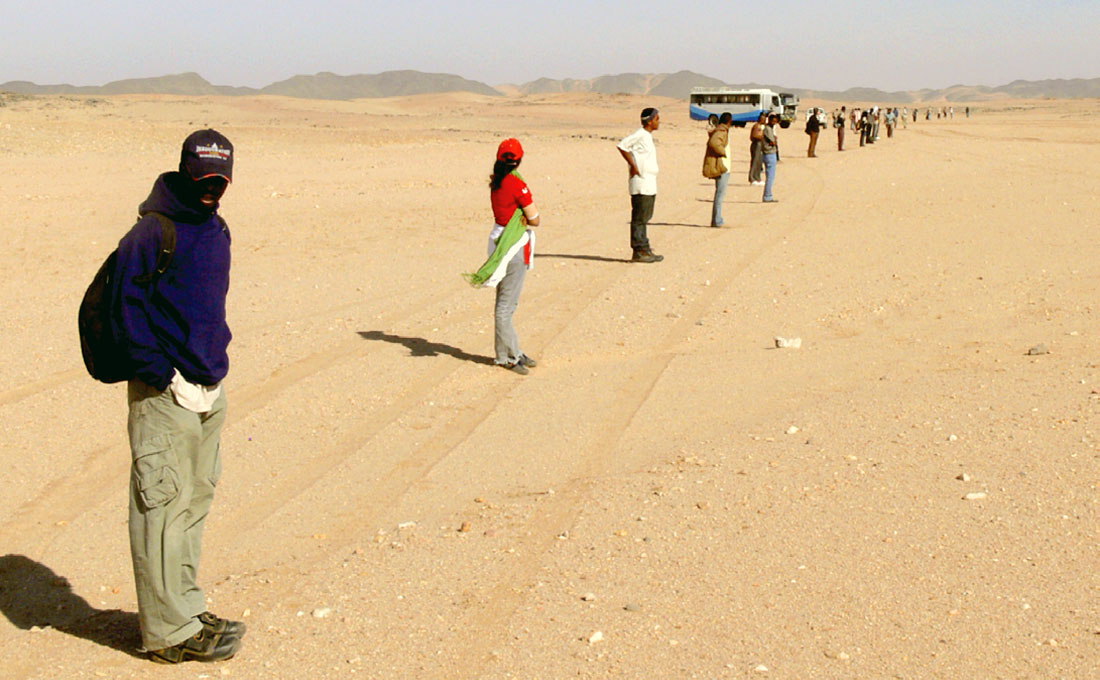
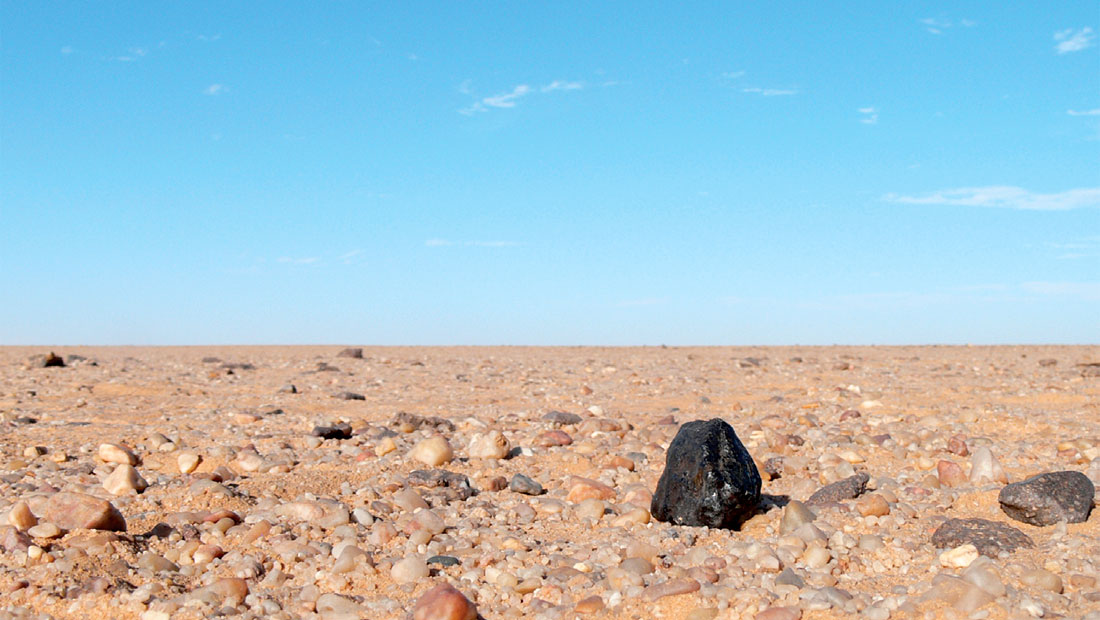
2014AA, the first NEO of 2014, was discovered by CSS 21 hours before entering the atmosphere, but conditions were such that the orbit was poorly determined. It entered the atmosphere over the mid-Atlantic ocean about 3,000 km east of Caracas, Venezuela. It was about the same size as 2008 TC3, and infrasound shock waves from the air burst were picked up by three stations of the Comprehensive Nuclear Test-Ban Treaty. Since the only data were the discovery images covering about 70 minutes, we know little about its physical characteristics.
A CSS object discovered in 2013 with the survey temporary designation WT1190F had a highly elliptical geocentric orbit, and was linked to three other objects with a 22.66-day orbit. It is thought to be the trans-lunar injection stage of the Lunar Prospector launched in 1998. When the final “discovery” was linked to the previous ones, it became clear that it was not a natural object, so was never given an MPC designation. It was predicted to reenter the atmosphere on 2015 Nov. 13 just south of Sri Lanka. Because of the timely determination of the impact time and location, it was possible to deploy an airborne observation team from the United Arab Emirates Space Agency, which recorded the entry fireball. It entered the atmosphere at 11 km/sec in the daytime (Figure 9).
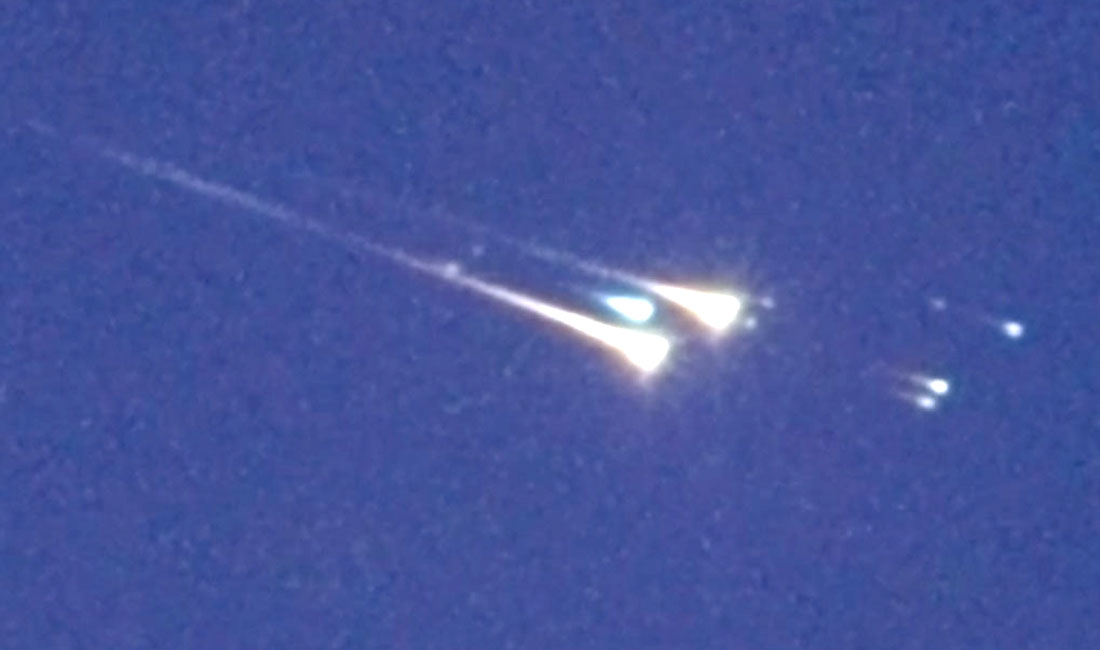
Main Belt Comets, or active asteroids; a new class of Solar System objects
Active asteroids in the main belt belong to a new class of asteroids exhibiting mass loss much like comets. About 18 MB asteroids have shown evidence of activity due to a variety of mechanisms. Exogenic activity might include collisions with other asteroids, like 596 Scheila, or effects of radiation forces causing rotation spin-up and ejection of regolith material, or even whole scale splitting up of the asteroid's “rubble-pile” structure. Endogenic activity would be periodic outgassing of volatiles, like water, that entrain regolith dust from deep deposits of ice. The largest MB asteroid 1 Ceres shows a weak atmosphere of water vapor. A smaller CSS discovery MB asteroid P/2014 (Gibbs) shows a curved tail consistent with long-term outgassing from an internal volatile reservoir (See Figure 10).
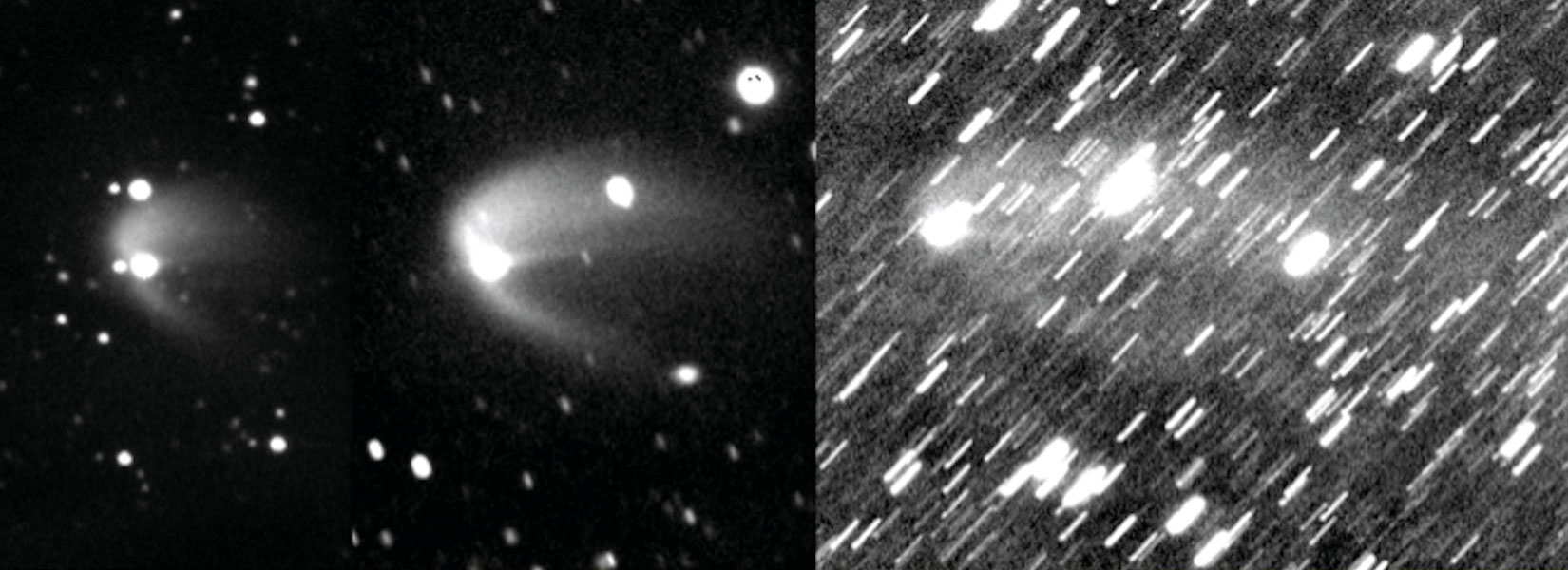
Several of these active asteroids have been discovered over the course of the CSS. To better investigate the asteroids activity, the Hubble Space Telescope is used to provide the resolution unattainable from the ground and can provide constraints in interpreting the mechanism for the activity.
Water-driven activity in the main belt may be significant in signifying a water reservoir responsible for terrestrial water. We continue to watch for more active asteroids.
Beyond the Solar System
While the CSS is optimized for detecting moving NEOs, the data can also be used to detect stationary transients. In 2007, we partnered with Caltech as the Catalina Real-time Transient Survey, which takes the images as they come off the pipeline, and looks for all objects that change brightness, rather than movement. Such transients are announced nearly real-time so that rapid follow-up spectroscopic observations can be made to identify the process causing the brightness change before they become too faint to observe. The temporal coverage over the years makes it easy to identify supernovae since they just pop up and fade away a few weeks later. Such objects will reside in the background star field far from our solar system. Since then, these include 2770 Supernovae, 1318 Cataclysmic Variables, 389 Blazars, and 3384 Active Galactic Nuclei. These are all of interest to astrophysicists (See Figure 11).
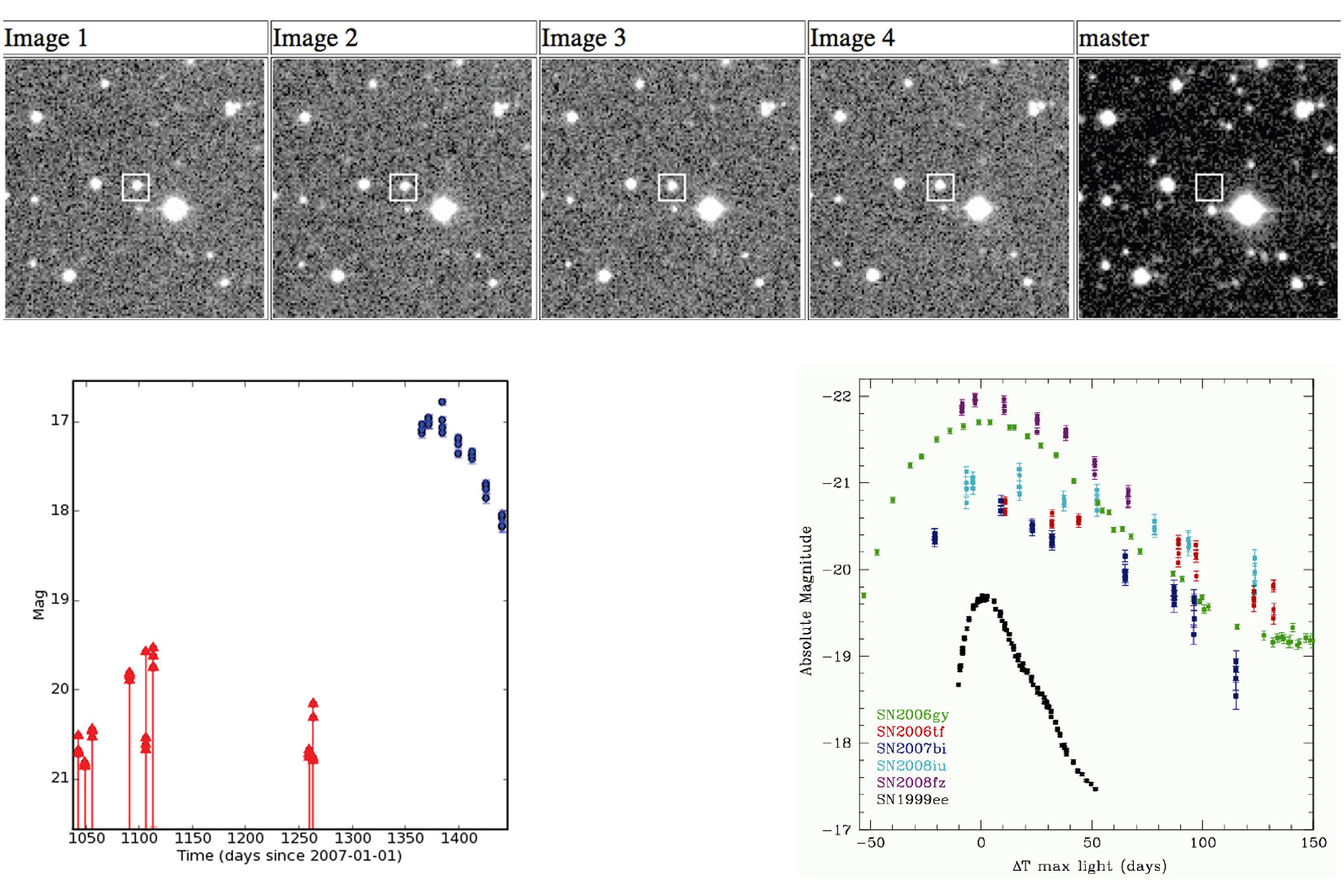
Combining data on 71 billion images of 500,000 sources from many visits over the years has led to the discovery of more than 61,000 new variable star light curves.
All of these results are possible due to the systematic coverage of the sky and the near real-time identification and publishing so that spectroscopic characterizing is possible while the object is observable.
Join the Conversation
Interested in starting, or contributing to a conversation about an article or issue of the RECORDER? Join our CSEG LinkedIn Group.
Share This Article