Abstract
The Canadian Beaufort Sea continental margin is probably the best known segment of the Arctic Ocean margin, based on the interpretation of multiple datasets defining the deep crustal architecture, regional structural trends and the temporal-spatial tectonic evolution. Of 53 oil and/or gas discoveries, most are trapped in structures formed during either Jurassic-Cretaceous continental rifting or Tertiary orogenesis.
Normal faults, active from at least Late Jurassic to mid- Cretaceous time, accommodated northwestward extension leading to continent margin formation in post-Albian time (ca. 95 Myr). The normal faults are best developed beneath Mackenzie Delta and Tuktoyaktuk Peninsula, and trap significant oil and gas accumulations, including the Parsons Lake gas field.
From latest Cretaceous to Late Miocene (Late Tertiary) time, five pulses of orogenic deformation are documented. The two most significant pulses are Paleocene to middle Eocene (Early Tertiary), and Late Miocene in age. The earlier event produced an arcuate array of structures onshore in northwestern Yukon and adjacent Alaska, and offshore within the continental margin basin. These structures did not develop exclusively by Cordilleran orogenesis because they include an important component of east-west shortening which probably was a response to North Atlantic seafloor spreading of latest Cretaceous and early Tertiary age. The Late Miocene pulse represents the final deformation event for much of the region. Holocene deformation occurs locally. The Miocene structures form long linear folds concentrated in the distal part of the Beaufort-Mackenzie basin. Folds and secondary faults related to these Tertiary deformation pulses trap oil and gas discoveries beneath the Mackenzie Delta, in the central Beaufort, and at Amauligak.
Introduction
Several major syntheses of the geology and tectonics of the Beaufort-Mackenzie region have been published over the last decade (Gabrielse and Yorath, 1992; Stott and Aitken, 1993; Norris, 1997). However, these syntheses are based on data largely acquired from the 1960’s to the mid-1980’s. Between 1985 and 1992, the Geological Survey of Canada undertook a major program of geological and geophysical data acquisition specifically designed to improve our understanding of the structural evolution, basin architecture, and deep crustal structure of the region. This summary is an overview of the major conclusions arising from that program.
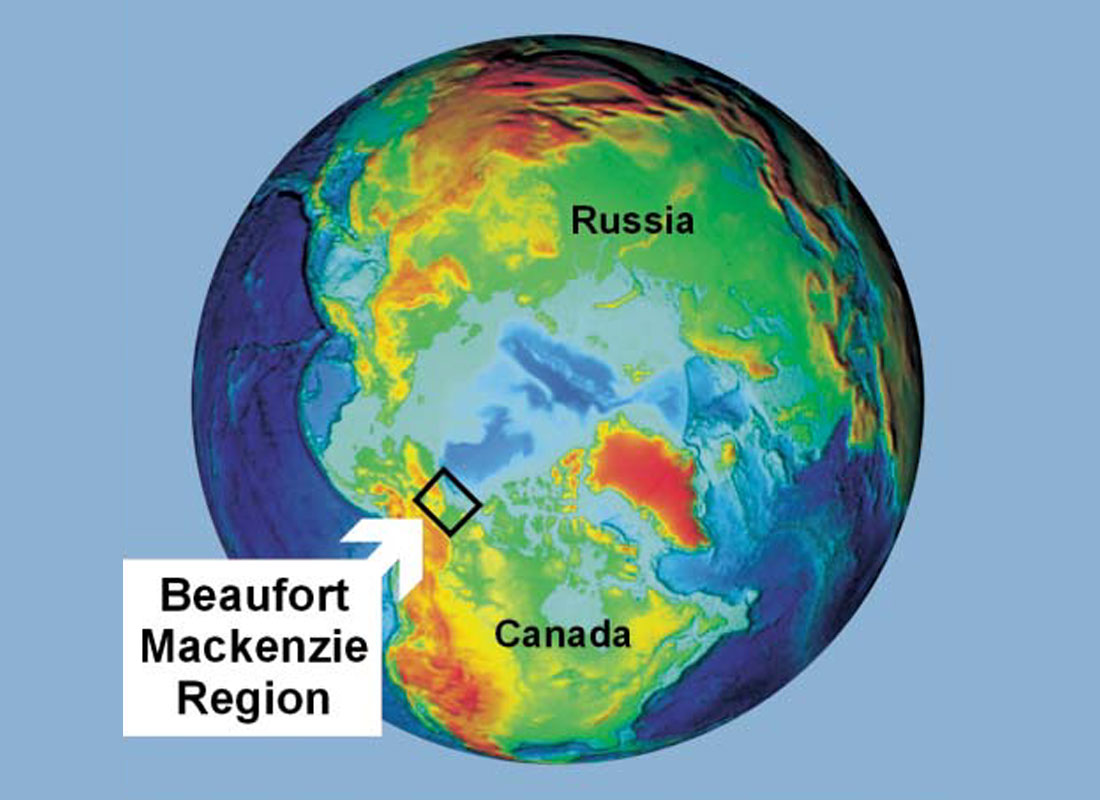
The Beaufort-Mackenzie region (Fig. 1) is an important frontier petroleum province that contains recoverable reserves estimated at 1.5 - 2 billion barrels of oil and 12 trillion cubic feet of natural gas in 53 significant discoveries (Dixon et al. 1994). The tectonic evolution of this region has played a vital role in establishing this oil and gas potential. Two principal stages of the regional tectonic history are particularly relevant. From at least the Late Jurassic to the Early Cretaceous (mid-Mesozoic), a period of rift faulting culminated in the formation of the Arctic continental margin (Embry and Dixon, 1990; Lane, 1997). Later, regional folding and faulting of latest Cretaceous and Tertiary age created additional traps for hydrocarbon accumulation (Lane and Dietrich, 1995; Lane, 1998).
Petroleum Potential
The Mackenzie Delta - Beaufort Sea region is widely considered to be one of the next major sources of conventional gas, required to satisfy projected increases in North American demand over the next decades. Important gas and oil accumulations are trapped in Mesozoic extensional structures, in particular the Eskimo Lakes Fault Zone, beneath Tuktoyaktuk Peninsula. For example, the Parsons Lake gas field (43, 44, 45; see Figure 2) contains 1.4 TCF of recoverable gas in two main pools, with subsidiary gas condensate and minor oil in a total of 13 separate prospects (Dixon et al. 1994).
The Atkinson Point oil discovery (12; Fig. 2) is also contained within the Mesozoic rifted zone beneath Tuktoyaktuk Peninsula.
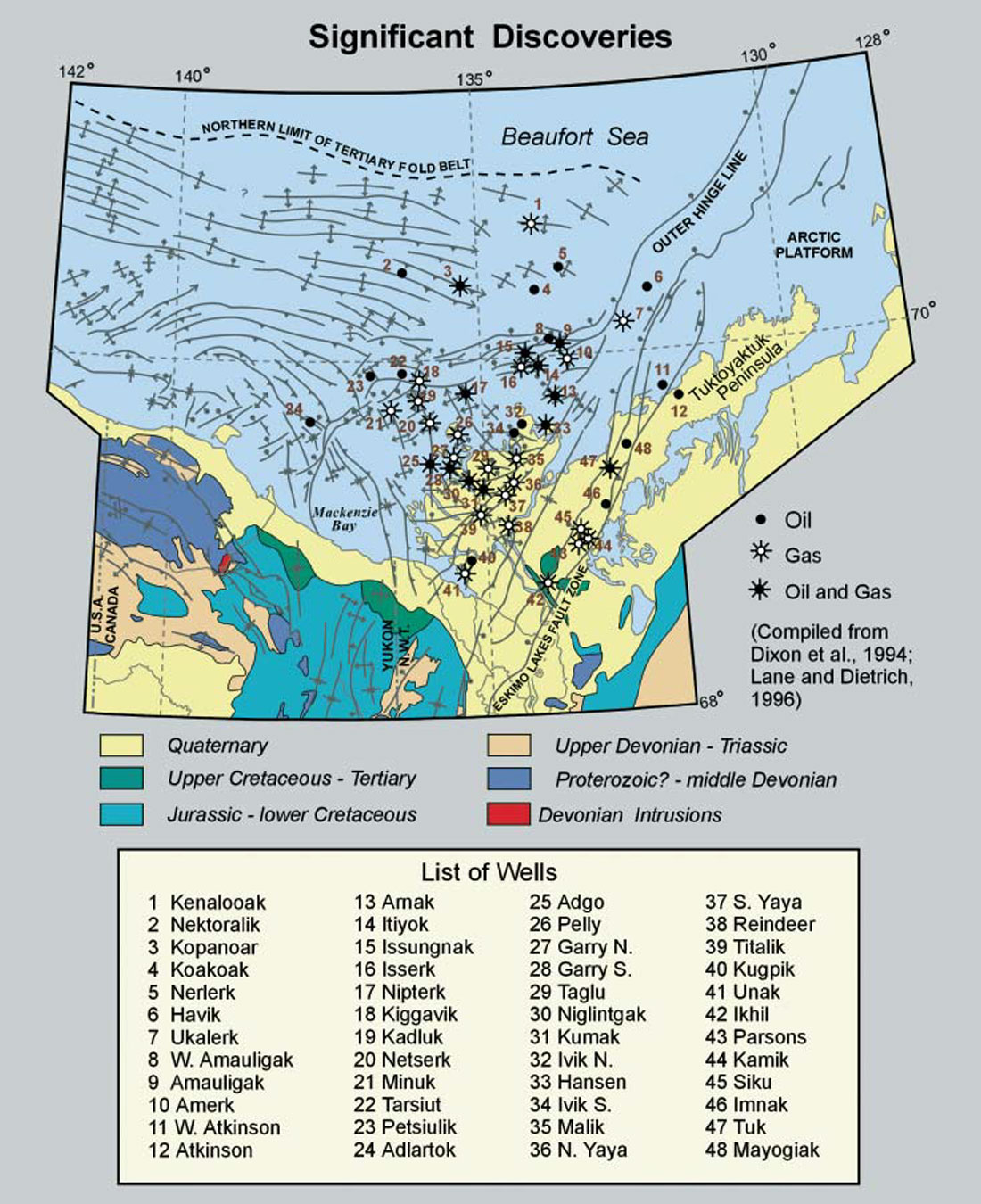
Oil and gas accumulations also occur in the hinge areas of Tertiary anticlines. This important structural setting beneath the Delta includes the Garry-Niglintgak (28, 30; Fig. 2) and Kadluk-Netserk-Adgo structures (19, 20, 25; Fig. 2). Examples of similar structures in the far offshore include Nektoralik (2), Kopanoar (3) and Adlartok (24). Many other significant deposits occur adjacent to zones of secondary Tertiary normal faulting in a broad structural transition zone near the basin margin. This setting includes major deposits such as Amauligak (89), Taglu (29) and Tarsiut (22). The Tertiary structural evolution is synthesized in Lane and Dietrich (1995).
Ikhil (42, Fig. 2) is the only currently producing field, supplying gas to Inuvik for local consumption. It lies near a subsidiary fault parallel to the Eskimo Lakes Fault, but penetrates a Tertiary reservoir.
Crustal Architecture
The regional crustal architecture of the Beaufort Sea continental margin is well understood, based on a variety of independent data sets. Regional surveys carried out by the Geological Survey of Canada include deep crustal reflection surveys (Cook et al., 1987; Dietrich et al., 1989, Lane and Dietrich, 1991; see Figs. 3 and 4), potential field studies, crustal seismic refraction surveys, and seismicity data (Stephenson et al., 1994; O’Leary et al., 1995). These studies were augmented by regional geological mapping, and public domain industry data comprising more than 200,000 line kilometres of seismic data and 247 exploration wells up to 5000 m deep (Lane and Dietrich, 1995). The transition from rifted continental crust to oceanic crust is outlined particularly well by the gravity anomalies and earthquake epicentres (Fig. 4). The generally northeast-southwest trend of extensional structures near the basin margin is largely inherited from Proterozoic and Paleozoic structural trends (Cook et al., 1987; Stephenson et al., 1994). The locations of tectonic fracture zones (Fig. 4) are defined by the truncation of gravity anomalies, the seismicity distribution, and the aeromagnetic signature (Stephenson et al., 1994; Lane, 1994, 1997).
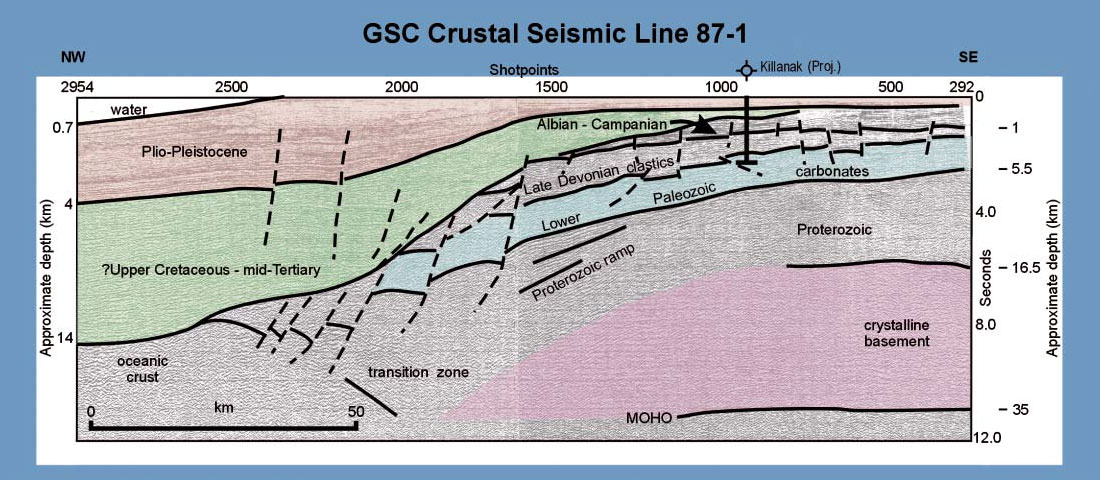
Mesozoic Extensional Phase
From at least Late Jurassic to Albian time, continental rifting produced large half-grabens and troughs, now preserved in the subsurface (e.g. Kugmallit Trough, Blow Trough; Fig. 4). Major bounding faults are exposed locally onshore and are well-defined seismically (Figs. 3 to 5). In the eastern Beaufort Sea continental margin, where Mesozoic structures are approximately parallel to pre-existing (basement) structures, the basin margin transition is narrow. In the west, where the Mesozoic trends diverge from basement trends, the transition zone is much broader (Fig. 4). The narrow transition zone is imaged in Figure 3.
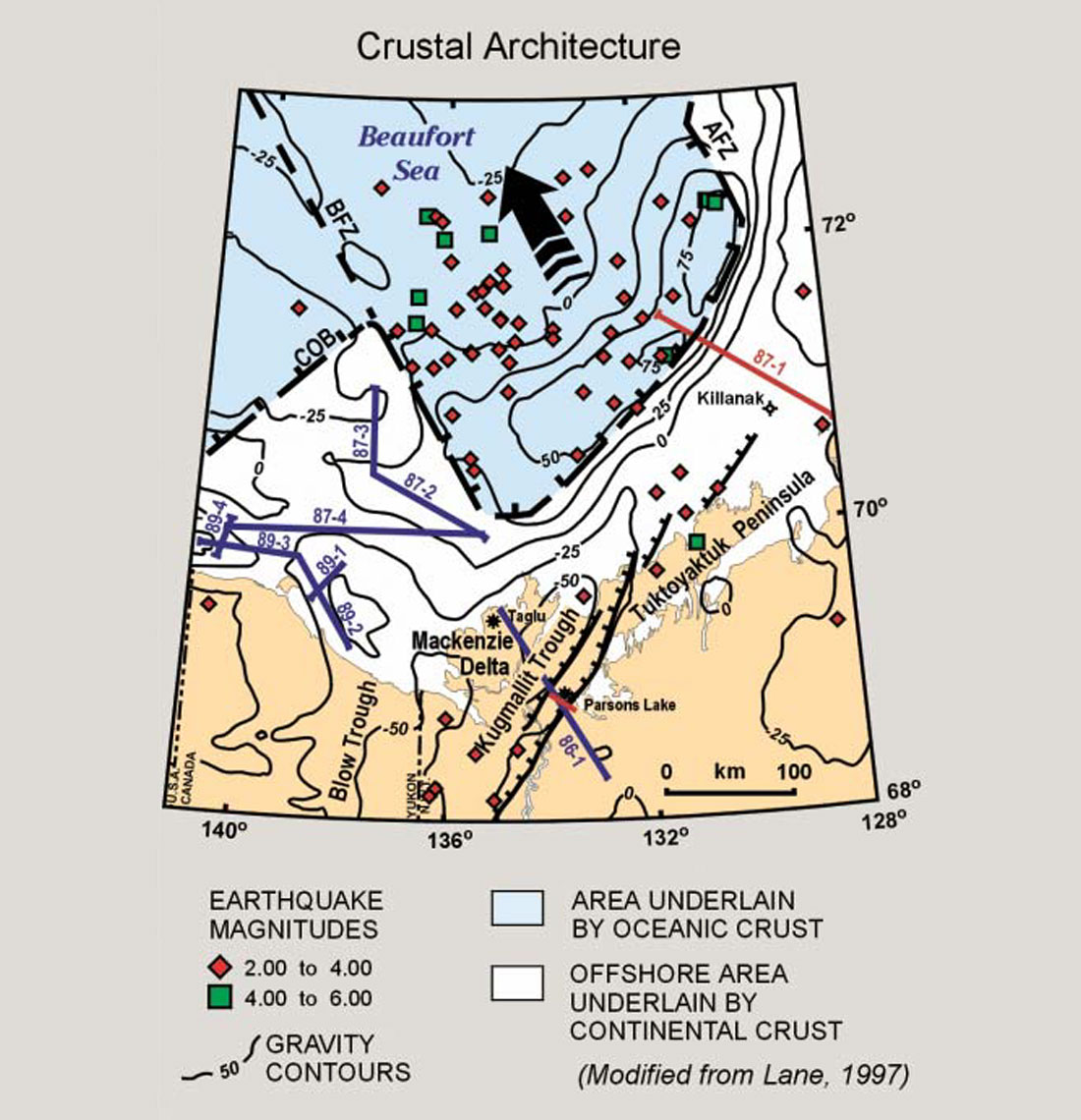
The extensional phase culminated in a major regional pulse of extension-related faulting and sedimentation in Albian time, part of a major continent-scale event affecting the full length of the Canadian polar margin from the Yukon to Ellesmere Island (Lane, 1997). In the Canadian Beaufort Sea Region, this event culminated in the formation of the western Arctic Ocean basin (Embry and Dixon, 1990).
The unconformity at the base of the Upper Cretaceous succession is identified as the breakup unconformity in this region because it marks the end of major rift-faulting along this part of the Arctic margin (Fig. 5). Public domain seismic reflection data through the Parsons Lake gas field (Fig. 5) images the Eskimo Lakes Fault Zone (ELFZ), a major listric normal fault underlying the Mackenzie Delta and Tuktoyaktuk Peninsula (Fig. 4). This zone marks the southeastern limit of the thick Jurassic to Tertiary succession and thus defines the basin margin in this area. The preserved thickness of the Jurassic to Lower Cretaceous succession is much greater on the northwest side of the fault than on the southeast, providing an excellent image of the sub-Upper Cretaceous breakup unconformity. Above the breakup unconformity, minor Tertiary reactivation has only slightly affected the younger succession. The Late Cretaceous is marked by relative tectonic quiescence, thermally controlled subsidence and sedimentation, coincident with the major phases of ocean basin formation in the adjacent western Arctic Ocean.
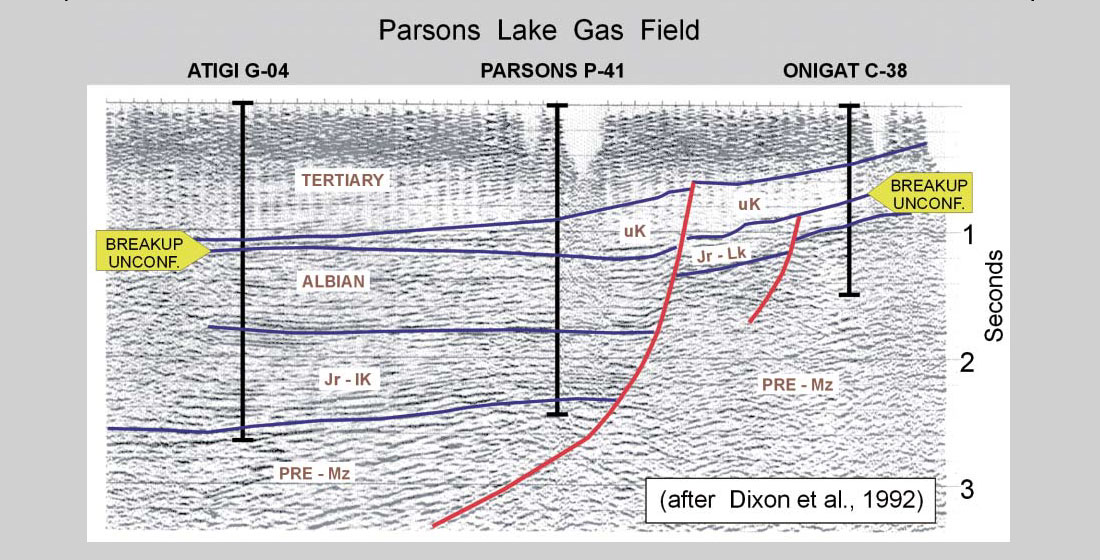
Tertiary Orogenic Phase
Beginning in Maastrichtian (latest Cretaceous) time, detached folding and thrust faulting climaxed in Paleocene to early Eocene time. Later pulses of minor deformation continued regionally until the late Miocene, and locally to the Holocene (Lane and Dietrich, 1995).
In general, the age of the dominant event within each part of the Beaufort-Mackenzie region shows a younging trend from latest Cretaceous and Paleocene in the hinterland to Miocene in the foreland (Fig. 6). The offshore data indicate that the deformation was episodic (Lane and Dietrich, 1995). Deformation ages for the offshore Beaufort Foldbelt are based on seismic stratigraphy controlled by data from exploration wells. Ages for the Brooks Range and Yukon north of 67º N are based on published apatite fission track cooling ages (e.g., O’Sullivan et al., 1993; O’Sullivan and Lane, 1997). Those for the Yukon farther south are based on published and unpublished biostratigraphic control. One small isolated area on the southwest margin of Eagle Plain shows a Paleocene age, reflecting a new, unpublished fission track age.
The “Post mid-Cretaceous” age constraint in the south (Fig. 6) reflects the lower resolution of the available fossil data, not an older deformation age. Facies and thickness trends of Late Cretaceous strata from Eagle Plain indicate that deformation postdates deposition of the Eagle Plain Group, which is as young as Campanian in age (Dixon, 1992; Lane, 1996). The pre-mid Cretaceous deformation identified in the extreme south reflects an earlier phase of Cordilleran evolution and is not discussed here.
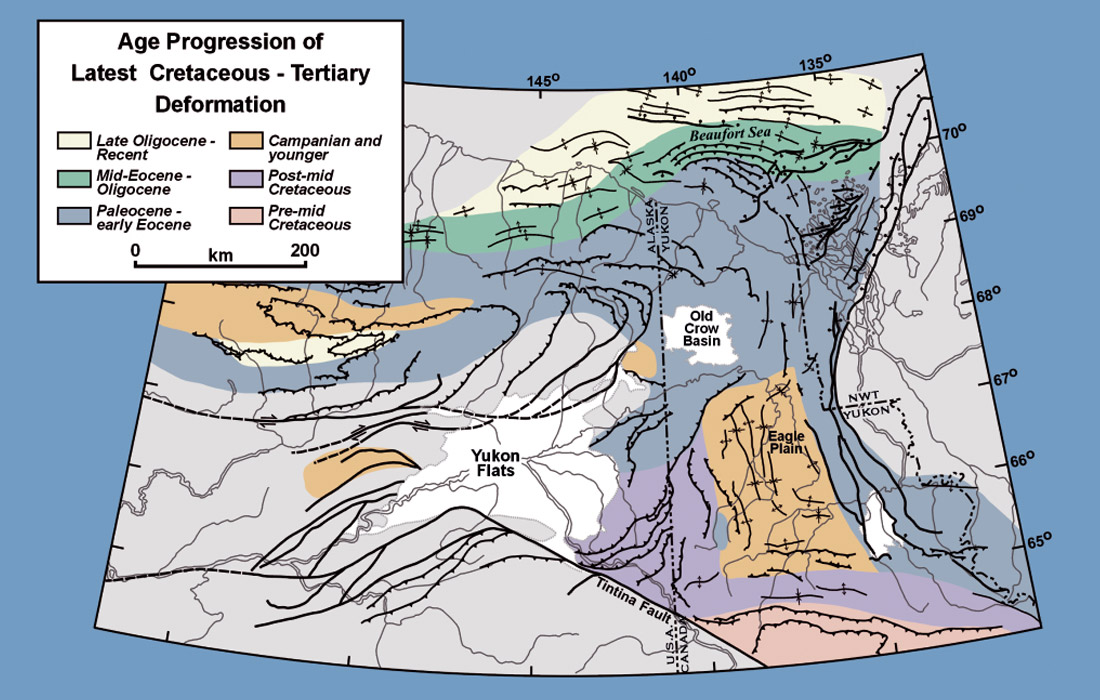
Paleocene to early Eocene (early Tertiary) deformation resulted from complex interactions of two complementary tectonic drivers. Cordilleran deformation relates to the collision of far-travelled tectonic terranes from the southwest, against the continental margin of western North America. Within the Beaufort-Mackenzie region, this translates into structures with generally northward tectonic transport directions. Simultaneous eastward motion of Eurasia against North America, related to the opening of the north Atlantic Ocean, produced important east-west shortening throughout northern Yukon. These tectonic drivers interacted to produce large scale arcuate fold systems both onshore and offshore. The shortening associated with north-directed folding and faulting is estimated to be on the order of 50-100 km; whereas the total east-west shortening across the Yukon and adjacent northeastern Alaska is on the order of 200 km (Lane, 1998).
Late Tertiary deformation relates only to Cordilleran tectonics. The Miocene structures form long linear folds concentrated in the distal part of the Beaufort-Mackenzie basin (Fig. 2). Also, reactivation of Jurassic-Cretaceous rift faults and early Tertiary structures is a widespread characteristic of the Miocene deformation, but it is particularly significant beneath the Mackenzie Delta (Lane and Dietrich, 1995). Secondary faulting is also important, especially near the eastern basin-margin (Taglu and Tarsiut-Amauligak fault zones).
Previous tectonic models have suggested that the region is characterized by strike-slip faults with hundreds or thousands of kilometres of displacement. Continuity of stratigraphic facies belts, and potential field data have been used to demonstrate that such models are not correct (Lane, 1992). However, local-scale strike-slip structures are well documented and may be important at the scale of individual hydrocarbon pools (Lane and Dietrich, 1995). This is particularly true of the southeastern part of the basin. Most of the existing hydrocarbon discoveries lie within approximately 150 km of the southeastern basin margin, defined by the Eskimo Lakes Fault Zone and the Outer Hingeline (Fig. 2). This broad zone is geologically complex because the crustal structure changes dramatically across it. Southeast of the basin, thick rigid basement is overlain by a thin cover of sediment. To the northwest, in the basin, the basement is very thin and is covered by young, very weak sediments as much as 14-16 km thick (Fig. 3). This transition zone defines a profound mechanical discontinuity. Accordingly, deformation is concentrated and complex in this area. This broad transition zone has been interpreted as having deformed, at least in part, as a zone of right-lateral oblique extension that accommodated Tertiary orogenesis within the deformed belt to the west, whereas the more rigid crust to the east was essentially undeformed (Lane and Dietrich, 1995).
Concluding Discussion
Although the basin architecture and structural geometry are well understood at the regional scale, more detailed mapping and structural analyses are required to refine this knowledge at scales that are useful to explorationists, regulators and local land administrations. Many structures are known but not adequately tested for petroleum potential. Still others, mainly offshore, will not be economically accessible in the near future. Despite important unresolved political, pipeline, engineering and environmental issues, expectations are high among local communities, governments and developers that Mackenzie Delta gas will soon bring prosperity to the region.
Join the Conversation
Interested in starting, or contributing to a conversation about an article or issue of the RECORDER? Join our CSEG LinkedIn Group.
Share This Article