Introduction
Seismic interpretation has been based traditionally on seismic reflectivity strengths measured by amplitude. Modern workstations and interpretation software calculate a wide variety of attributes based on seismic amplitudes. Historically, analyses based solely on amplitude have posed a number of significant problems for the interpreter. Reflection amplitudes in seismic data are blurred representations of the actual stratigraphy. The convolution of a non-stationary pulse shape with a wide variety of impedance contrasts in the subsurface adds a significant element of uncertainty to the final mapping of reservoir, seal and trap. Information is carried in the seismic signal at frequencies above and below the dominant frequency contained in the data. Recently developed spectral imaging techniques based on the continuous wavelet transform (CWT) allow geophysicists to investigate and characterize this information buried in the seismic signal.
SPICE (SPectral Imaging of Correlative Events) is an attribute that uses the continuous wavelet transform to calculate a bedform boundary framework from seismic data. SPICE extends the unique properties of the continuous wavelet transform to locate and describe spectral anomalies in the seismic signal. The result is a display that reduces the uncertainty in picking subtle bed-form boundaries and brings out the full extent of the resolution of the seismic amplitude data. SPICE offers a straightforward way to interpret a seismic section similar to the way a geologist in the field maps beds and faults directly from the outcrop.
Wavelet Transform and SPICE
There are many techniques for analyzing time-frequency relationships in seismic data. The Fourier Transform (FT) and the Short Time Fourier Transform (STFT) are the most popular transforms for decomposing a signal into its spectrum. The Fourier transform is best suited for stationary data (frequency content does not change with time). Signals that have different spectral characteristics at different locations in time are non-stationary. Our common experience tells us that the frequency content of seismic data changes with time. To adequately describe a non-stationary signal, a time-frequency method must simultaneously isolate time and frequency information. Figure 1 shows the amplitude spectrum of a non-stationary signal calculated using the Fourier transform. The Fourier method successfully maps the time domain signal to the frequency domain but time localization is lost. The Fourier transform reveals how much of each frequency exists but not where in time it exists. The STFT represents an effort to deal with non-stationarity by subdividing the signal into smaller portions that are assumed to be stationary. Although the STFT does remedy some of the localization problems associated with the FT, it is not optimal since it uses a single window length for all frequencies. Short time duration windows do not properly sample low frequency components and long time duration windows do not localize the high frequency components.
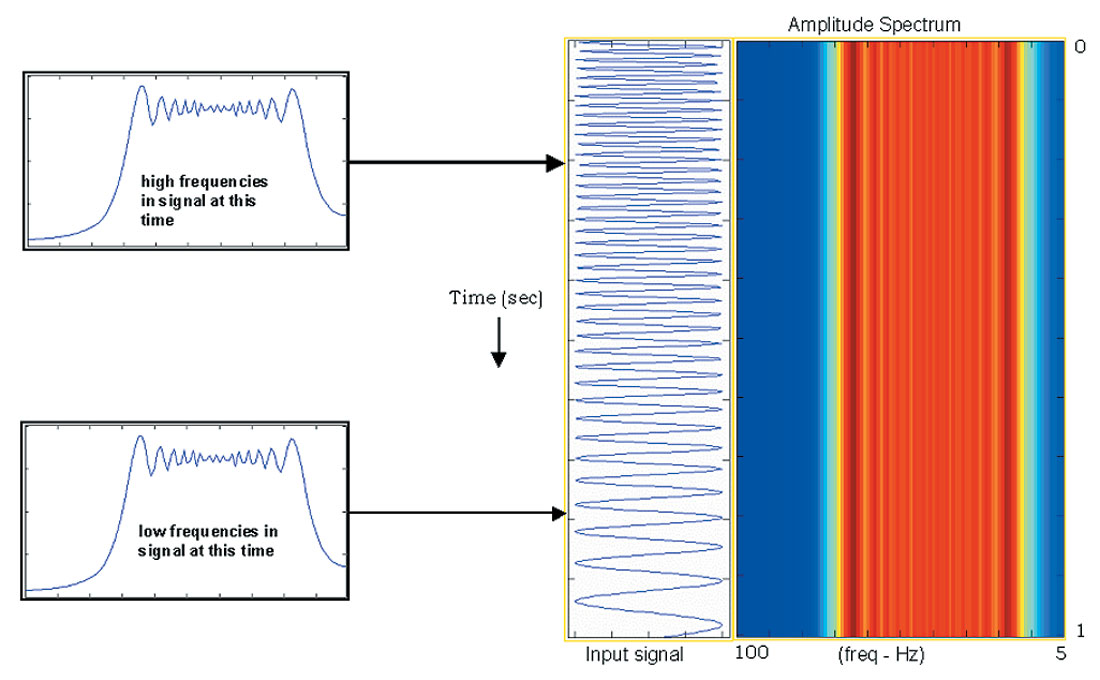
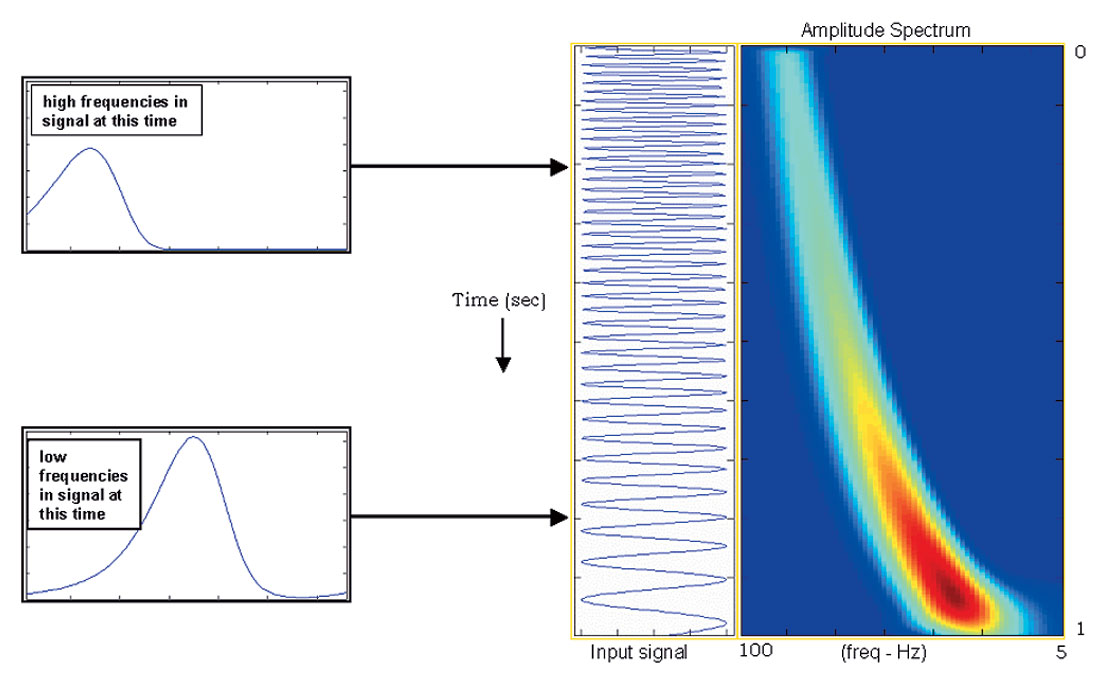
The continuous wavelet transform (CWT), which is a more recent development, circumvents the fixed window length restriction by using multiple window lengths, with each window length dependent on the frequency or scale being analyzed. Shifting different scale wavelets along the trace decomposes the seismic signal. Figure 2 shows the amplitude spectrum of a non-stationary signal calculated using the CWT. The CWT locates temporally the position of events as well as providing a localized spectral distribution of the constituent frequencies. The properties of the CWT result in better time-frequency localization of the signal.
The wavelet transform may be defined as:

where ƒ( t ) is the time-domain data (a seismic trace), ψ∗ (t) is the complex conjugate of the analyzing wavelet, τ is the translation parameter and σminσ σmax is the scale parameter. The wavelet transform is computed across a range of scales and shifted over the entire trace according to the translation parameter τ . F (σ ,τ ) is therefore the transformed trace at a particular scale and translation. SPICE (Liner et al., 2004), which is based on the CWT, uses a complex Morlet wavelet (the product of a Gaussian and a complex exponential). Although Morlet wavelets lack some of the rigorous mathematical properties of other wavelets (they do not form an orthonormal basis), they are nevertheless well suited for seismic data due to their similar oscillatory behaviour.
Using complex Morlet wavelets, SPICE maps a single trace to the time-frequency plane and then analyzes the spectrum at each location along the trace to detect anomalies. These anomalies determine the location of bed-form framework boundaries. Since SPICE condenses the spectrum at each sample, there is no data proliferation as there is with spectral decomposition. Thus the input seismic volume and the output SPICE volume are of the same dimensions.
Stratigraphic and Fault Interpretation of SPICE Attribute Displays
The SPICE bed-form boundary framework advances mapping of complex stratigraphy and structure because new criteria are added to guide the interpretation. These new criteria and how to use them are demonstrated in a series of examples taken from seismic data in West Cameron, a shelf area in the northern Gulf of Mexico. A base map of general locations is shown in Figure 3. Figures 4-5 validate the straightforward use of the SPICE bed-form framework directly as beds or groups of beds, similar to outcrop geology. This validation is presented within the context of a sequence stratigraphic interpretation of the SPICE sections correlated with lithology fraction (LithFrac) curves. LithFrac curves are calculated well log curves that are regionally calibrated and normalized to indicate sand percentage.
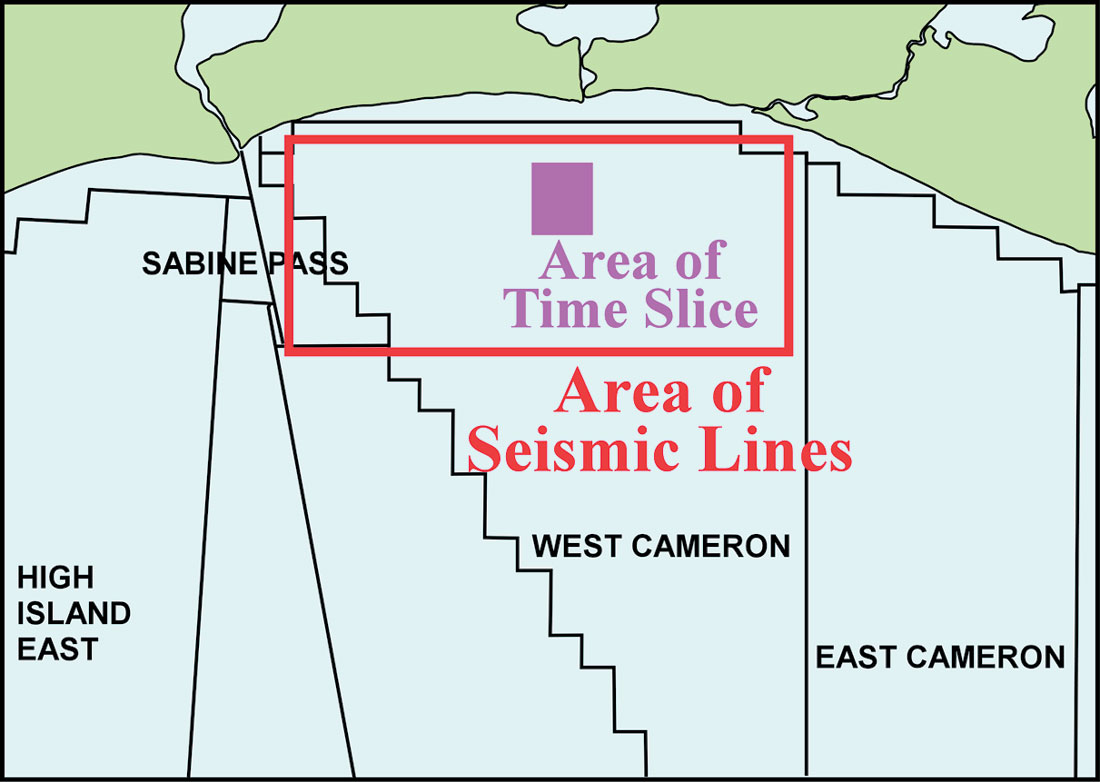
The key criteria offered by SPICE are discrete boundaries formed by its unique calculation of spectral anomalies in the seismic time trace. The colour bar used in the figures for this SPICE bedform framework is a black-grey-white scale, with colours at the top end of the figures showing stratigraphic interpretations. The black end of the colour bar shows the position in time of the sharpest anomaly boundary.
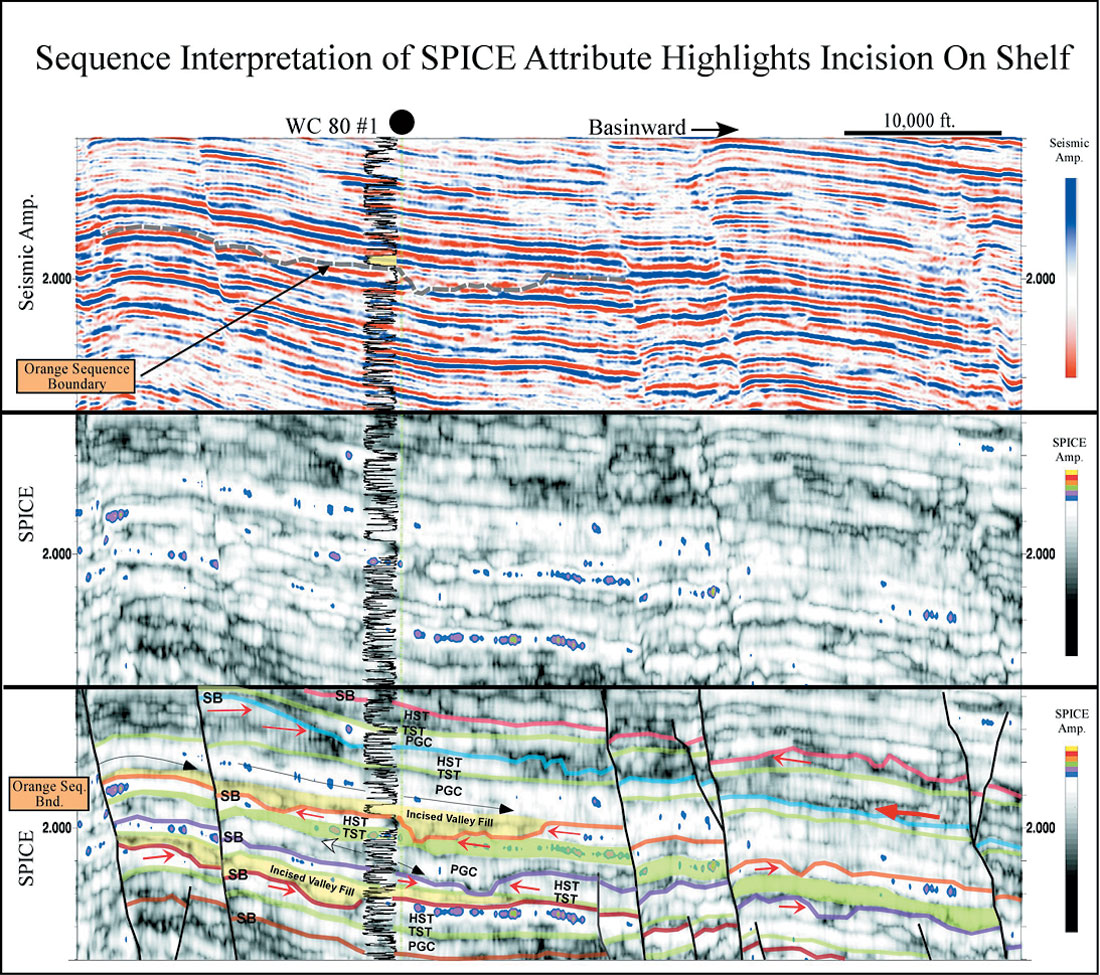
Figures 4-5 show a more detailed examination of the bed boundaries on the SPICE sections. Each figure shows a LithFrac curve overlain on a SPICE section. A sequence stratigraphic interpretation is performed based on the integration of the well log shape and the patterns of the SPICE bed-forms. A legend for the sequence stratigraphic symbols used in the figures is shown in Figure 6. The colours of the sequence boundaries on the figures are only for illustration and do not represent the same time-correlative sequence.
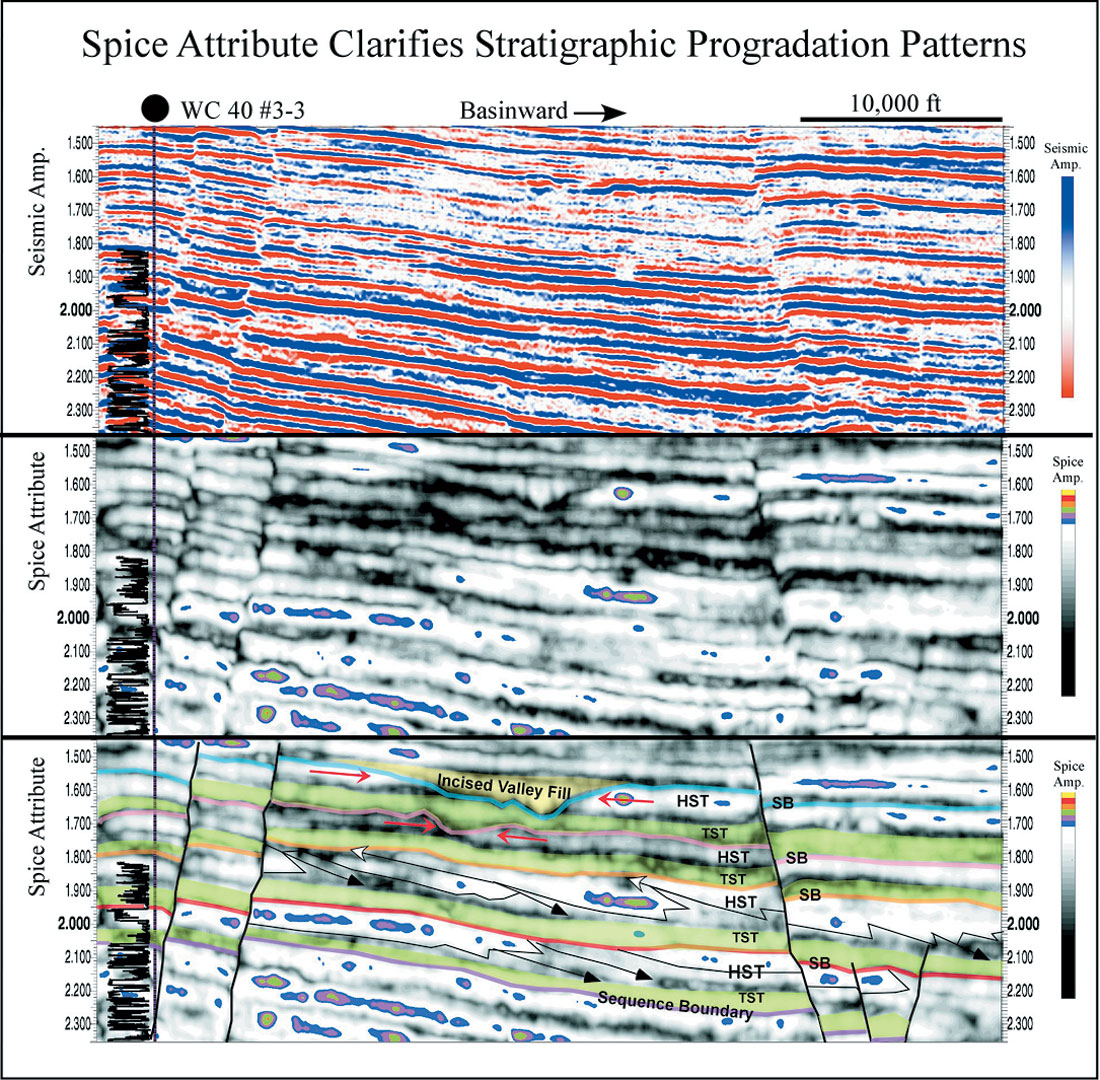
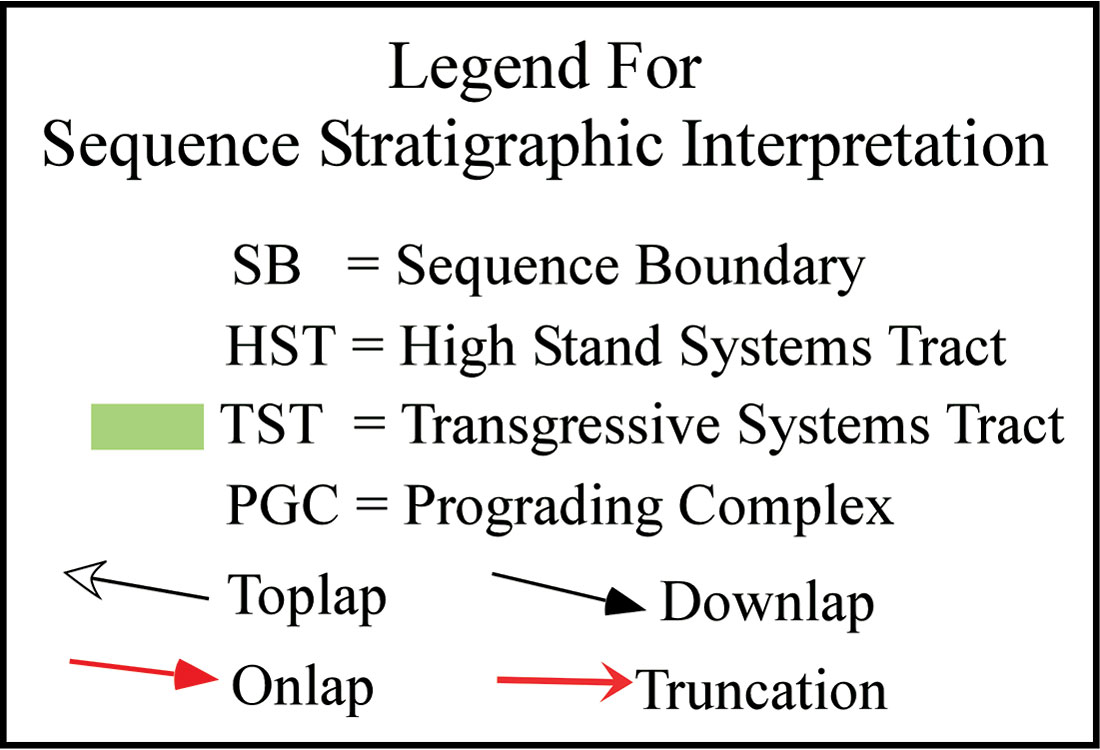
These interpretations show many of the characteristic features of shelf sequence architectures. Figure 4 highlights the distinct incised sequence boundaries at almost every sequence. The two most prominent Incised Valley Fill units are coloured in yellow and the SPICE bed-form boundary at the orange sequence boundary ties to the base of a prominent blocky sand in the well log. There is also a SPICE boundary at the top of this sand. A dashed line denotes the location of the incised orange sequence boundary on the seismic amplitude section. Geometries in the bed-form boundaries include downlap and toplap, onlap and truncation. The Prograding Complex (PGC) in this area shows subtle downlap geometry, and the Transgressive Systems Tract (TST), coloured in green, is the most laterally continuous unit, with the base Transgressive surface producing a distinct boundary. Figure 5 illustrates two highstand units that have downlap and toplap geometry with no incision on the SPICE section. These geometries are barely visible on the seismic amplitude section in these thin sequences. The shallowest blue sequence boundary shows a pronounced incision on the SPICE section that correlates to an ambiguous seismic amplitude geometry.
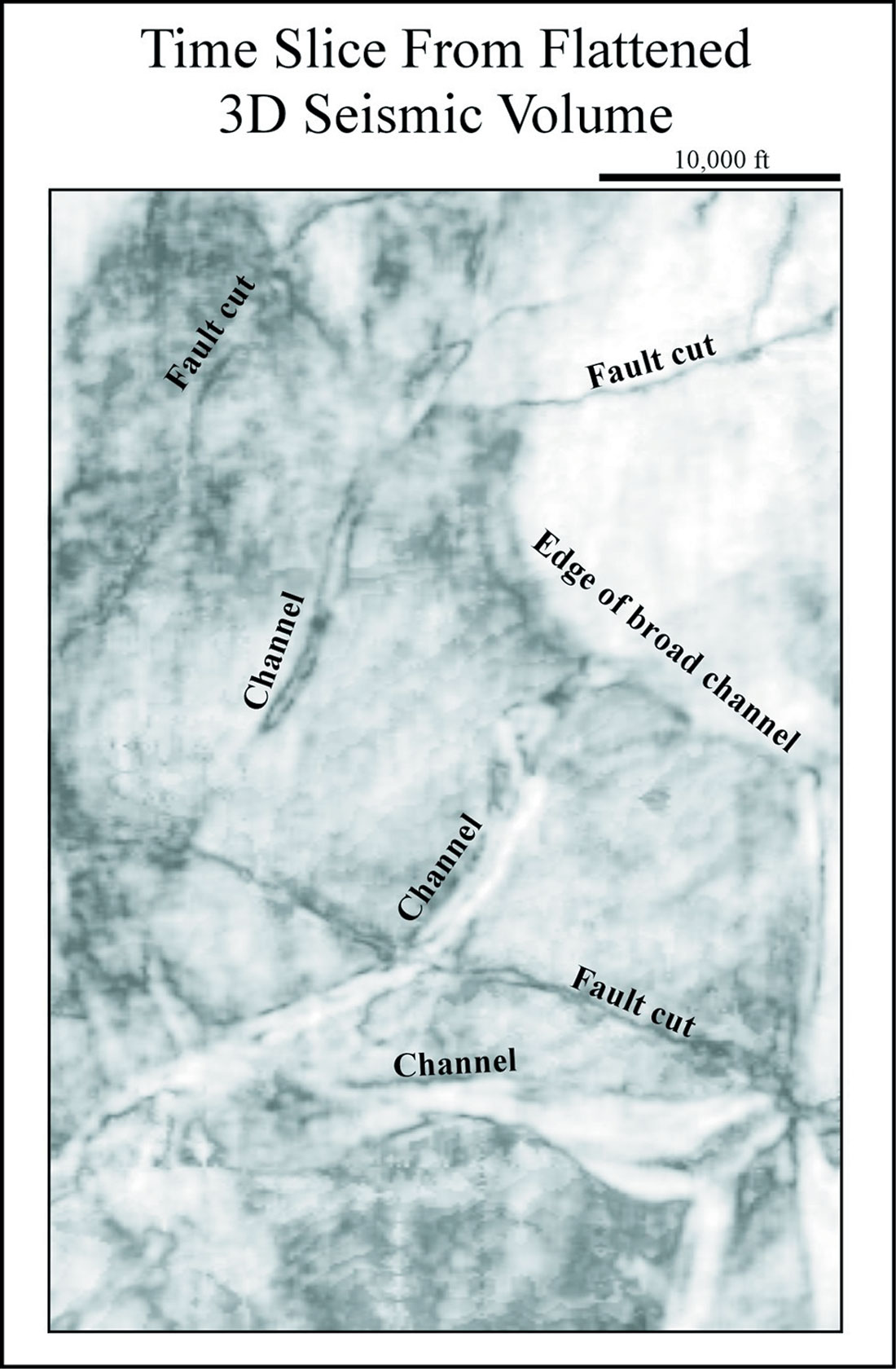
Further validation of the vertical SPICE interpretation can be demonstrated by viewing the data in a time slice. Figure 7 is a flattened time slice of several blocks in the West Cameron area of the Gulf of Mexico. The stratigraphic detail in the slice clearly demonstrates that SPICE produces laterally coherent features (channels and fault cuts) that are consistent with shelf architectures in the area.
Conclusion
The method of mapping a seismic signal to the frequency domain determines the amount of information that can be extracted from the signal. The spectral richness and nonstationary character of the seismic trace require a process that localizes rapidly changing features in the spectrum. For geophysicists investigating the spectral behaviour of seismic reflections, traditional methods of time-frequency decomposition impose a trade-off between frequency resolution and time localization. Wavelet-based spectral imaging is an alternate time-frequency method that not only gives global frequency content but also localizes, in time, where individual frequency components occur. SPICE extends the wavelet transform by analyzing and characterizing the frequency spectrum at each time sample. Single traces are extracted from a seismic volume, pass through the wavelet transform and a process that detects spectral anomalies, then reconstructed into a new volume. The resulting attribute creates a bed-form boundary display that represents an advancement in the level of structural and stratigraphic detail that can be extracted from seismic data.
The characteristics of the SPICE bed-form boundaries match closely to detailed sequence stratigraphic boundaries from well logs. Incised shelf sequence boundaries resolve as well-defined discrete boundaries on vertical SPICE sections and examples show much higher detection of these boundaries compared to seismic amplitude sections. Additionally, complex structural interpretations are assisted by precise location of bed offsets and SPICE amplitudes. Horizontal slices from SPICE produce geologically consistent results in complex stratigraphic and structural settings.
Acknowledgements
The authors thank Fairfield Industries for permission to use and publish data for this paper. This implementation of SPICESM is an extension of research done by C.-F. Li and C. Liner, University of Tulsa.
Join the Conversation
Interested in starting, or contributing to a conversation about an article or issue of the RECORDER? Join our CSEG LinkedIn Group.
Share This Article