Abstract
Lithology and principal stress are the main driving mechanisms behind the development of the subsurface geopressure profile. Lithology distribution creates the conditions that lead to compartmentalization. Sequence/seismic stratigraphy are good guide lines to establish the paleoenvironment and sediment’s spatial distribution in a basin.
The overburden (principle stress) is a result of the sum of sediment and water weights. Current water depth in offshore has a direct impact on the size of the regional hydrostatic-lithostatic pressure envelope. The combination of changes in lithology and the current water depth affects the pore pressure shift from normal hydrostatic to an abnormal geopressured system. The petrophysical properties of the impermeable beds (shale, mudstone), which are represented by maximum flooding surfaces, sequence boundaries, and parasequences, are responsible for geopressure compartmentalization. Therefore, sequence / seismic stratigraphy can lead to the establishment of the expected pressure profile in both seals and reservoirs at any specific location in the basin.
Introduction
Recognition of the architecture of the seismic reflectors is essential to defining the depositional cycles in clastic basins. In a progradational system (low stand), where deltaic coarse clastic beds (parasequences) move basin-ward, sandy facies dominate the inner shelf environments. Pore pressure (PP) profile shows a dominant hydrostatic gradient with a medium sealing capacity across the seals. On the other hand, in a retrogradational (high stand), where mud rich sediments move toward the land mass, PP profile shows higher gradients and shale beds show higher sealing and retention capacities.
On the slopes, course sediments bypass the mud rich highly inclined surface. Consequently, the PP profile shows a fast increase with occasional compartmentalization.
In the bathyal zone, where most of the basin floor fans (BFF) rest, a more relaxing PP profile develops. A moderate to low sealing capacity exists due to the presence of the relatively high permeable reservoir sediments. Moreover, the current large hydraulic head retards the development of the geopressure profile. Therefore, drilling for hydrocarbon in deep water needs to reach greater sediment depth relative to the play concept on the shelf.
Concepts and Methods
For the purpose of this article, two maximum flooding surfaces (MFS) and three sequence boundaries (SB) were used to display the impact of sequence stratigraphy on PP development (Fig.1). A carbonate platform was assumed as a foundation for these cyclic events. The maximum flooding surfaces demarcate the final stage of retrogradational parasequences. The upper two SB’s represent low stand events associated with multiple progradational parasequences (PS). The lower SB represents a hiatus between the carbonate base and the younger sediments.
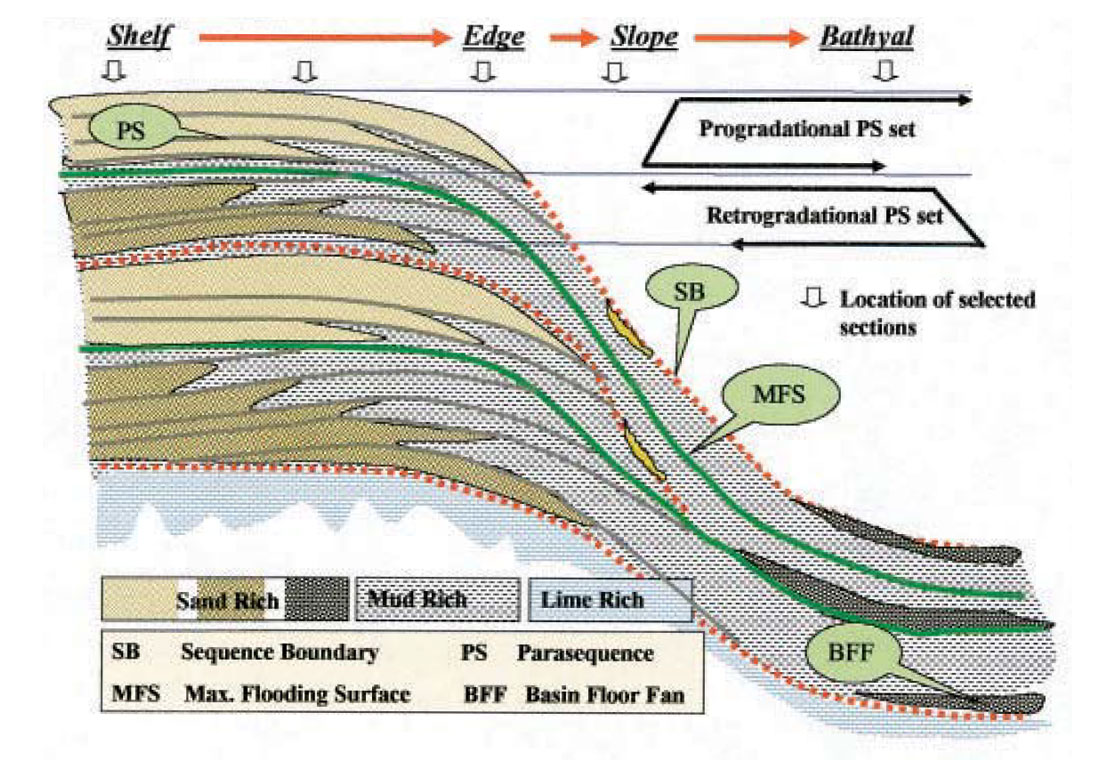
To illustrate the impact of sequences distribution and the current water depth (mud line below sea level) on the pore pressure development, five bore-hole locations were selected to represent each environment:
1. Inner Shelf PP Profile: The lithology of the section in the inner shelf (inner neritic) is dominated by sandy rich sediments. As long as diagenesis and meteoric alterations did not impact the primary porosity, compartments usually are represented by thick permeable facies. Maximum flooding surfaces are the strongest seals rather than the low permeable bedding planes within the parasequences.
The pore pressure profile is characterized by a cascade fashion type track (Fig.2). The PP in the normal pressure coincides with the regional hydrostatic gradient in the basin. The MFS usually represents the top seal of the geopressured section below. Compartments in the geopressured system bear gradients similar to the regional hydrostatic gradient, but pressure progresses in different envelopes. The lithostatic pressure commences at shallow depth and leads to a large principal stress and higher retention capacity in the geopressured section. The sealing capacity of the interbedded shale beds are moderate in nature, especially if they represent a sequence boundary between progradational and retrogradational parasequence sets.
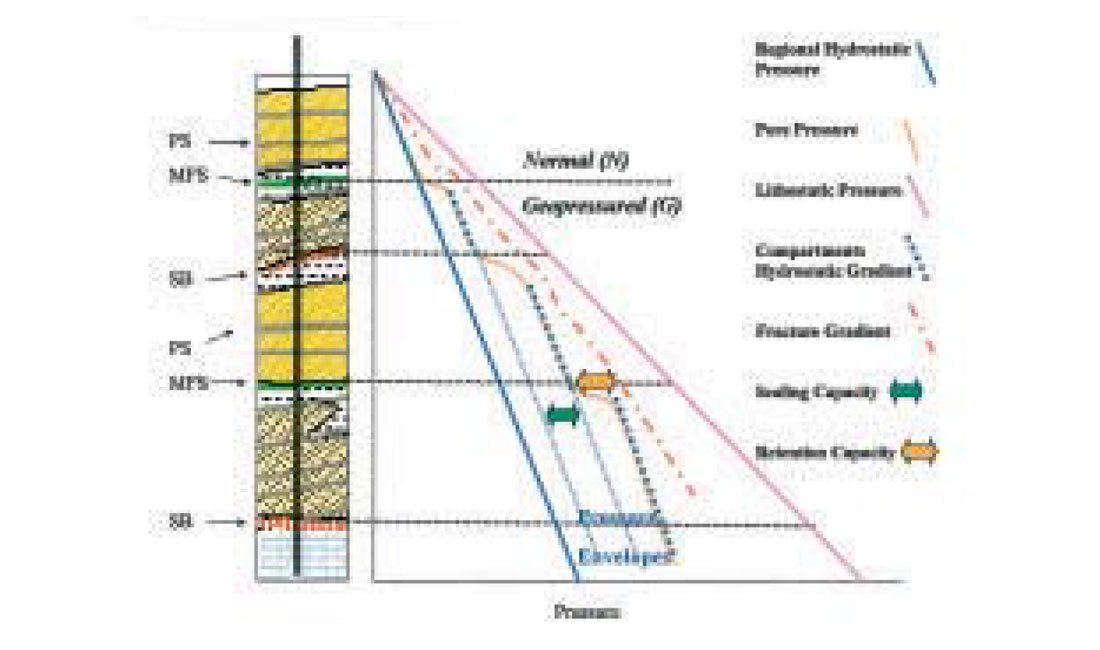
The predominant sandy facies in the inner shelf section increases the risk of trapping mechanisms, especially within faulted structural segments. Therefore, large potential hydrocarbon accumulation in this zone is unlikely unless four way prospective closures are targeted.
2. Outer Shelf (Outer Neritic) PP Profile: The outer shelf (outer neritic) is the most optimum environment for hydrocarbon entrapment. The reservoir quality in each compartment is built of medium to fine clastics. Moreover, the relative large thickness of the shale beds enhances hydrocarbon entrapment in faulted segments.
A considerable shift of pore pressure envelopes takes place where the maximum flood surfaces thick shale are present. Progradational / retrogradational parasequences usually show a moderate to weak pressure shift. The PP profile is characterized by relatively high sealing and retention capacities (Fig.3).
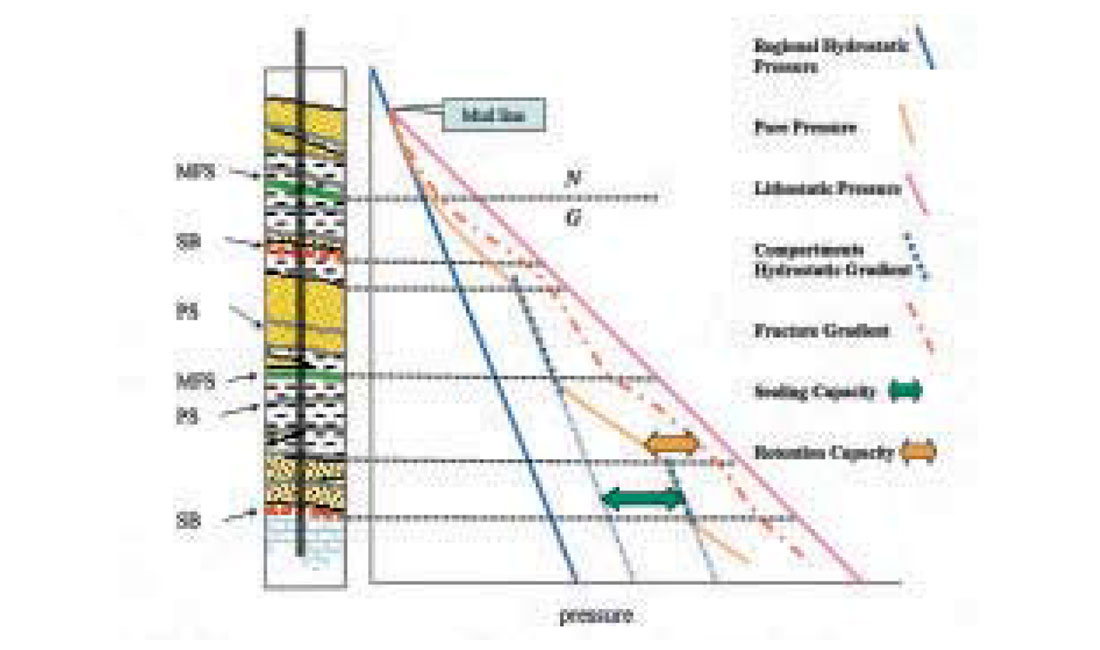
The outer shelf environment of the middle–lower Miocene in offshore Texas and Louisiana represents ideal case histories of highly successful exploration programs. The adequate sand percentage (25-35%) and strong sealing/retention capacities are the main reason for finding substantial reserves. Explorationists describe this paleoenvironment belt as a fairway for optimum play concept choices.
3. Shelf Edge PP Profile: The geological setting on the shelf edge is dominated by prodelta facies with occasional sand rich progradational parasequence sets. The frequent presence of very low permeability sediments leads to the acceleration of pressure gradient below the cap of the geopressured zone. The less frequent presence of permeable compartments and the down shift of the mud line (Fig.4) leads to a closeness between the PP gradient and the fracture gradient (FG). Therefore, retention capacity of seals for hydrocarbon entrapment is relatively low. Most of the shelf/slope break discoveries in the GOM are limited in their size and productivity.
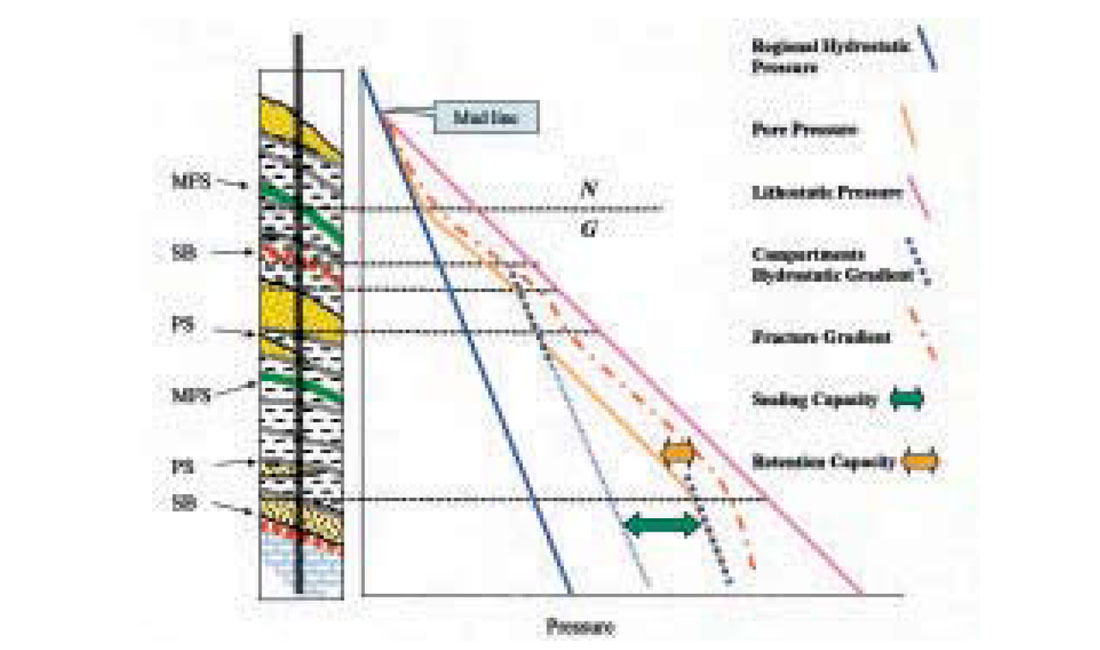
4. PP Profile on the Slope: The slope environment is dominated by mud rich sediments. Slumped and rafted blocks are occasionally found embedded in the low permeability host sediments. On the other hand, incise valleys and channel cuts can be found and these are usually filled with permeable sediments. The combination of the low permeability section and the large hydraulic head (due to the deep bathometry) leads to a fast acceleration of the PP gradient commencing at the mud line. The closeness of the PP and fracture gradients leads to a low retention capacity (Fig.5). Moreover, due to the sediment’s high tilt, centroid effect can inflate the PP to the fracture pressure in reservoir beds.
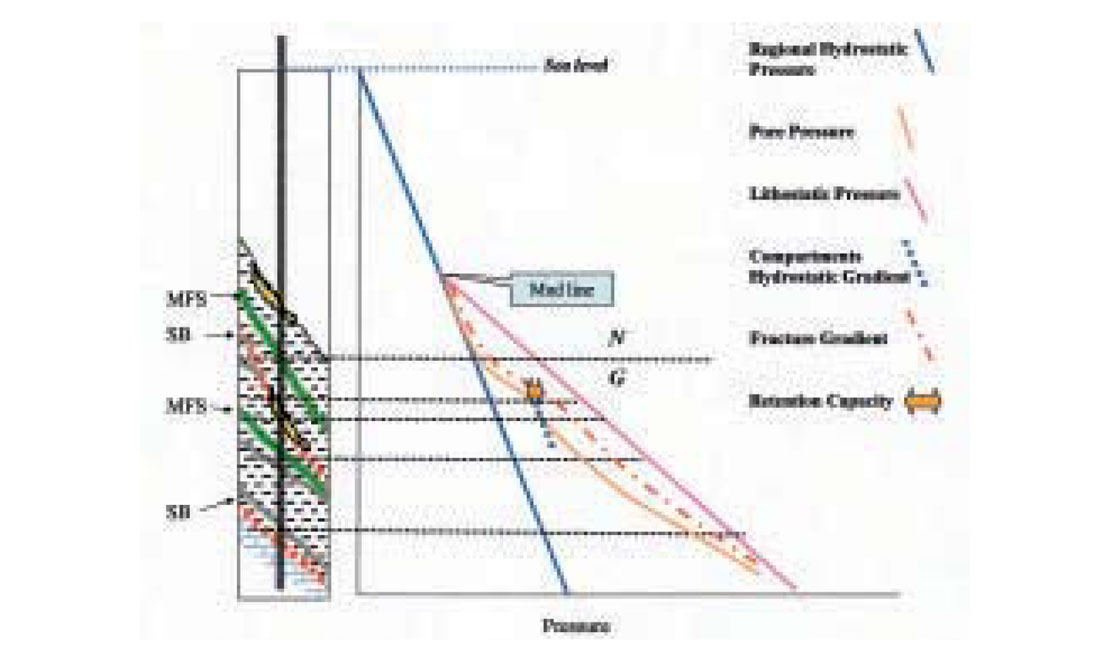
5. PP Profile in the Bathyal Environment: The deep water bathyal basins usually receive enormous sediment influx during the low stand progradational system. Most of the high energy sediments bypass the edge/slope zones and pond in turbidite masses. Basin floor fans form in cases where these sediments find a passage (sub-aqueous channels) to bathymetric low areas. These occasional relatively thick coarse clastics are usually embedded in the deep seated bathyal mud rich facies.
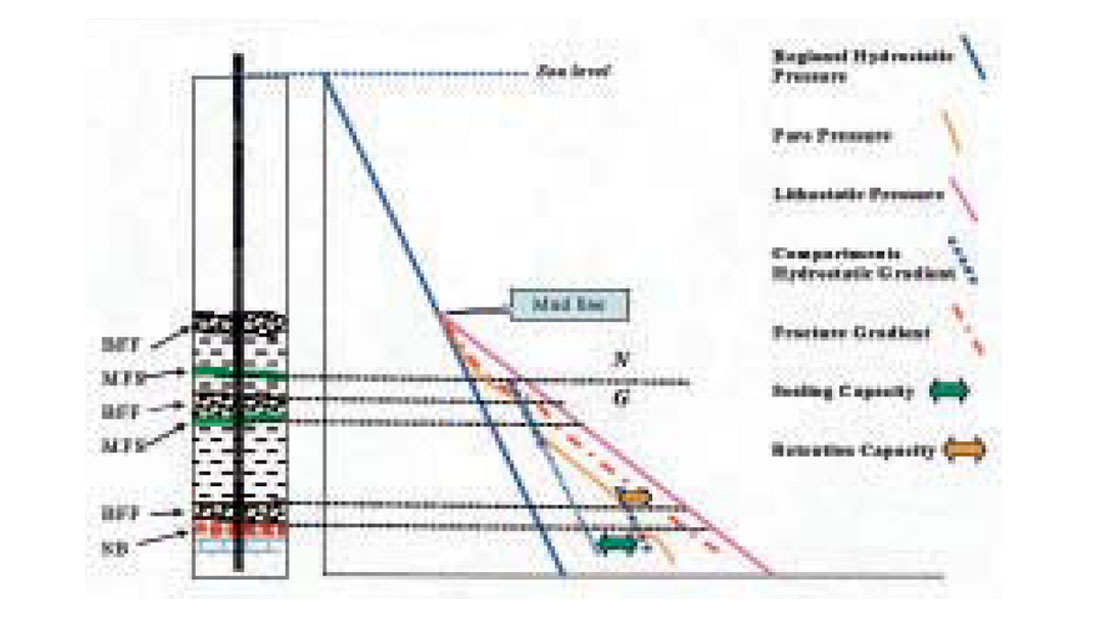
The large gap between the current mud line and sea level leads to a substantial reduction in the principal stress. Therefore, the pressure envelope between the regional hydrostatic and lithostatic gradients commencing at the mud line is relatively small. This leads to the closeness of the PP, FG and lithostatic gradients (Fig.6). Therefore, in spite of the presence of relatively moderate to high sealing capacity, retention capacity is very limited in the shallow part of the sedimentary section. For a successful exploration play in the deep water, deeper sediment objectives need to be targeted. Drilling deep targets compensates for the loss of the principal stress due to the current water depth. In the GOM deep water exploration, most of the economically feasible projects were found at greater depth than the discoveries found on the shelf.
Conclusions
Seismic facies analysis leads to the build up of sequence stratigraphy frame work. Predicting lithology in different paleoenvironment provinces in the geologic setting of a basin in relation to the current water depth are the back bone of pore pressure profile assessment.
On the shelf (inner and outer neritic), pore pressure develops in a cascade fashion. Compartments usually bear hydrostatic gradients. The shift in these pressure envelopes dictates the sealing capacity (SC). On the other hand, the difference between the PP and FG corresponds to the retention capacity (RC) for hydrocarbon entrapment. Outer shelf environment usually represents an optimum exploration trend due to the relatively high SC, RC and the optimum lithology distribution.
On the edge/slope, where mud rich sediments dominate, pore pressure profile increases at a higher rate. The closeness between the PP and fracture gradients is responsible for the limited retention capacity. In deep water environment where basin floor fans, turbidites, and sub-aqueous channel fills exist, retention capacity is relatively low at the shallow part of the section. Conversely, sealing and retention capacities increase in the deep part of the section.
Join the Conversation
Interested in starting, or contributing to a conversation about an article or issue of the RECORDER? Join our CSEG LinkedIn Group.
Share This Article