The top few kilometers of the Earth’s surface are of extreme importance to our society. This near-surface region houses mineral and hydrocarbon wealth that are crucial for industrialized development, water needed for life, and it is an environment with which we must interact to build our infrastructure. To exploit these resources, and also to mitigate problems that arise from their exploitation, we need to image the earth’s interior without direct sampling. Substantial insight about the structure and composition about the near-surface can be gleaned by determining its physical properties. Density, magnetic susceptibility, electrical conductivity and elastic parameters can be critically diagnostic, and data from appropriate geophysical surveys can be inverted to generate 3D distributions of these properties. Since each structure or object in the ground is defined by its own suite of physical properties, it follows that the most reliable information can be acquired by carrying out inversions from different types of geophysical surveys and examining the cumulative set of recovered physical properties.
The goal of inverting all types of geophysical data is laudatory but the computational ease of inverting different surveys varies with the amount of data and the intrinsic resolution of the survey. Gravity and magnetic surveys generate simple data sets that arise from a single source and hence full 3D inversions have been available for nearly two decades. DC resistivity and IP (induced polarization) data are more complicated because they involve a number of transmitters, but these too are regularly inverted. It is only recently, however, that electromagnetic data in frequency and time, using multiple transmitters and multiple component receivers, can be inverted on computational grids that are fine enough to capture the relevant geology. For this reason, and because electrical conductivity is often a diagnostic physical property used for geologic interpretation, I concentrate upon methods to recover electrical conductivity in my talk.
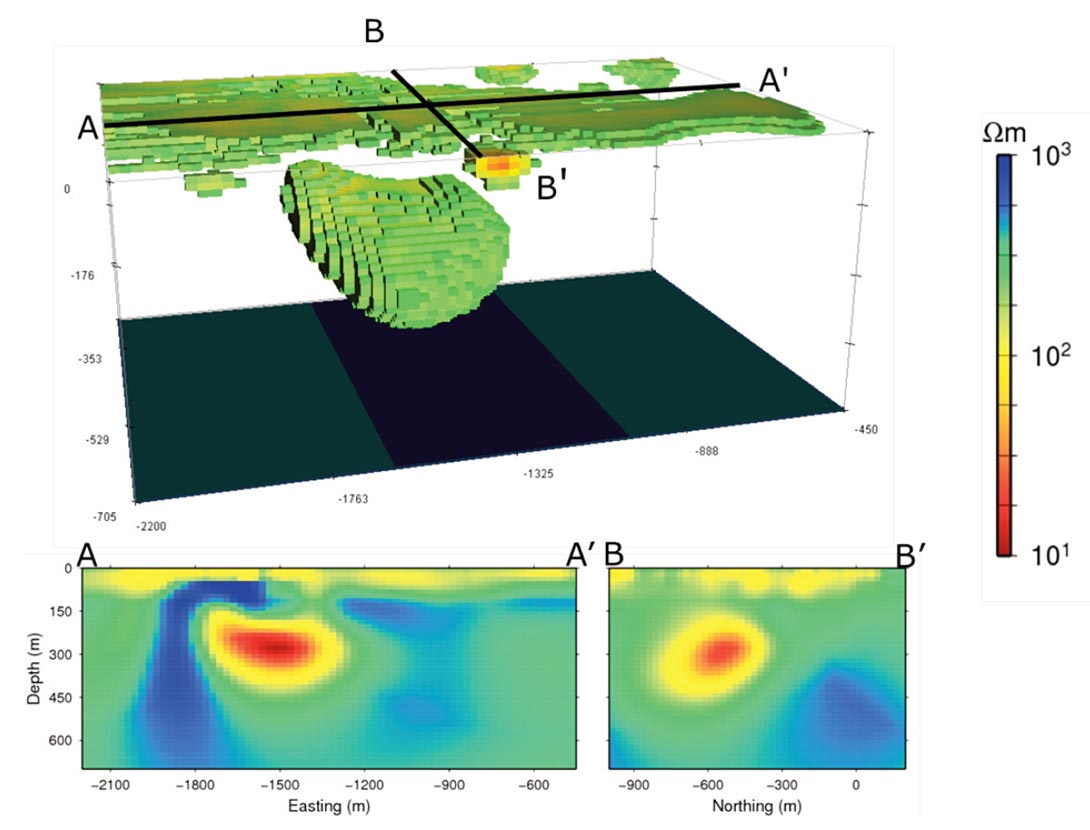
In a typical electromagnetic experiment a time-varying current in the transmitter induces currents in the earth which diffuse away from the transmitter. The data consist of electric and/or magnetic fields measured in time. Inverting these fields for an arbitrary waveform is analogous to carrying out full waveform inversion of seismic data. I illustrate some of the basic principles of the EM technique, and apply them to a mineral exploration problem where the location of a massive sulfide is sought. The result of inverting time domain data from three loop transmitters is shown in Figure 1. The field site is ideal because multiple geophysical survey methods have been performed over the site and there is substantial well information to provide ground truth with which to verify our inversion. The sulfide body is dense, magnetic, conductive and chargeable. Locating regions of high values of these physical properties indicates a likely location to spot a drill hole. A composite image that illustrates this is shown in Figure 2.
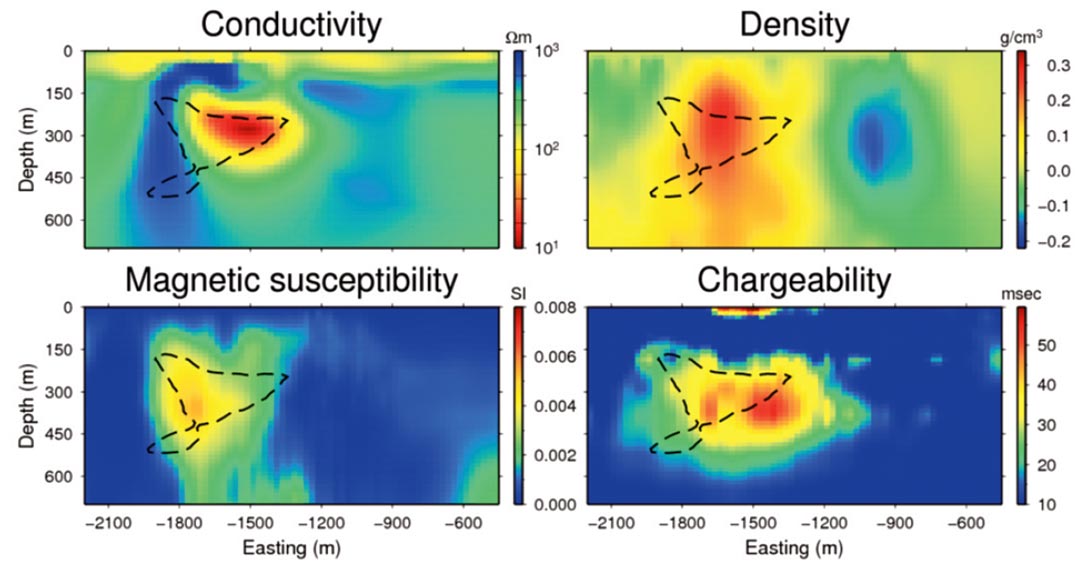
Electrical conductivity is diagnostic for a large range of problems that are characterized by different physical dimensions. For example, on the small scale we want to find a UXO that has dimensions of tens of centimeters. On the larger scale, we want to detect a deeply buried hydrocarbon deposit in a marine environment or detect a large mineral deposit. Fortunately, electromagnetic surveys are scalable. Small lowpowered systems are appropriate for small items while large highpowered systems are required to look deeper. For some investigations, however, the energy that can be generated through a man-made source becomes inadequate, and we must appeal to natural sources. Of particular interest is the ZTEM (Z-Axis Tipper EM) survey which is a modern version of the AFMAG survey used in 1950s. In the ZTEM technique, measurements of the vertical component of the magnetic field are acquired in an airborne survey and transfer functions with respect to the magnetic fields at a base station are computed. Data, which are obtained over a range of frequencies, can be inverted to yield a 3D conductivity structure. The sensitivity of the data can extend to depths of a kilometer or more and hence this survey has considerable potential for resource exploration and geologic mapping. Figure 3 shows the results of inverting synthetic data from a mineral exploration area.
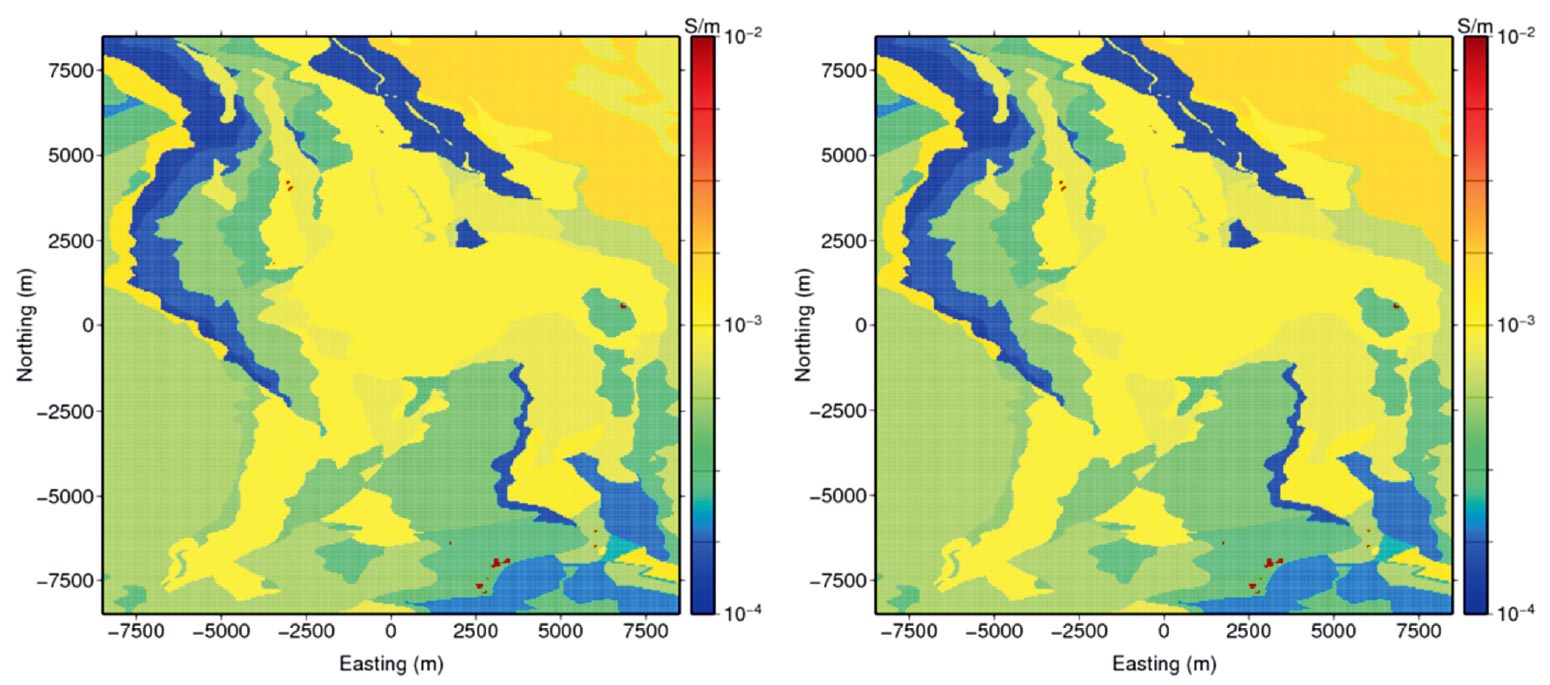
Because this talk will be presented to SEG and affiliated societies, as well as to student chapters, details of the talk will change according to the audience. The goal for the society meetings is to illustrate current capabilities for 3D EM inversions and to stimulate the desire to acquire enough data, of sufficiently high quality, so that good results from an inversion can be obtained. An additional goal for the student chapters is to illustrate that real-world problems are solved within multidisciplinary teams. In order to carrying out his or her job effectively, the geophysicist needs high level computational skills, as well as a strong background in math, physics, and geology.
Join the Conversation
Interested in starting, or contributing to a conversation about an article or issue of the RECORDER? Join our CSEG LinkedIn Group.
Share This Article