A course organized by the Geoscience Department of NTNU (Norway) took place in mid-June in Aínsa, Spain. Graduate students from NTNU, TUDelft (Netherlands) and the University of Calgary participated. Geological outcrops were used to provide an introduction to deep marine, shallow marine and fluvial sedimentary systems. Implications of these sedimentary environments for carbon capture and sequestration were investigated, as well as the consequences for geophysicists and reservoir engineers considering such systems. Such environments may provide attractive targets for potential storage of fluids thus it is important to understand their development, structure, and relationships to other geologic features. This course was extremely beneficial to the development of well-rounded geoscientists as it reinforced the importance of stepping out from behind the computer screen and going into the field to study outcrops, leading to a strengthened understanding of the subsurface.
Course Overview
From June 15-19, 2017, students from the Departments of Geoscience and Chemical and Petroleum Engineering at the University of Calgary traveled to Spain to participate in a geologic field course in the Aínsa Basin, as part of Project CARBEOR, a collaborative training program between the Norwegian University of Science and Technology (NTNU) and the University of Calgary, for advancing CO2 storage and CO2 enhanced oil recovery. The Containment and Monitoring Institute (CaMI) of CMC Research Institutes Inc, is also involved in CARBEOR. Two students from the Delft University of Technology (TUDelft) also attended the Ainsa course. The primary objective of the course was to give engineering and geophysics graduate students the opportunity to observe and study various sedimentary depositional structures and environments, and to explain how these features would impact the injection and flow of carbon dioxide during subsurface sequestration. As the participating students were not experts in field geology, the field trip was designed to provide an introduction to a wide variety of sedimentary environments. The varied geology and spectacular outcrops of the Aínsa basin made this location an ideal choice for the course. An additional goal of the course was to bring together graduate students, postdoctoral researchers, and professors from the U of C, NTNU, and TUDelft in order to build relationships, facilitate the exchange of ideas, and encourage collaboration between participants in the field of carbon capture and storage (CCS). Participants also have the opportunity to be involved in the future cross-pollination of ideas as part of an ongoing effort which has already seen student exchanges between Canada and Norway.
While the course focused on simple geology concepts, it provided numerous valuable insights for geophysicists interested in CCS. Particular emphasis was given to the implications of sedimentary environments on the development of porosity and permeability of the rock strata, especially as they relate to the reservoir and seal of potential carbon sequestration projects.
Basin Overview
The Aínsa Basin is located in the Pyrenees of northeastern Spain (Figure 1), and is part of the deep-marine Graus/Tremp-Aínsa-Jaca Basin complex in northern Spain (Figure 2), which formed as a result of thrust activity related to the evolution of the Pyrenees (Pohl and McCann, 2013). The basin is widely studied and geologically significant due to the abundance of easily accessible outcrops representing several different depositional environments and structural features. The namesake town of Aínsa, approximately 300 kilometers northwest of Barcelona, served as a central hub for daily excursions to outcrops and viewpoints in various parts of the basin.
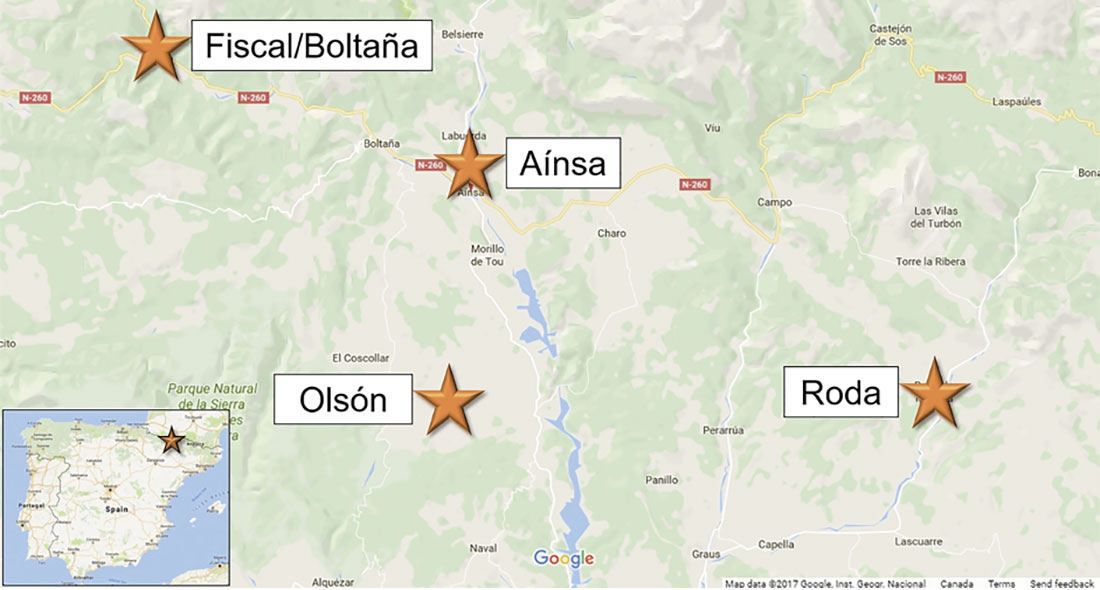
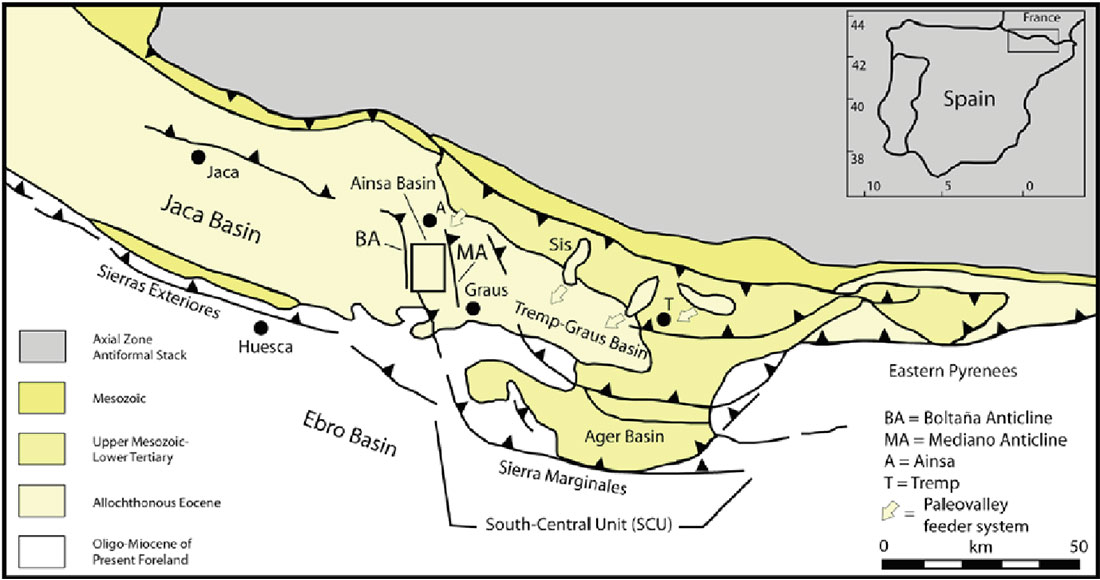
Formation of the basin was associated with the Pyrenean orogeny, caused by the convergence of the Iberian and European Plates during the Late Cretaceous (Sibuet et al., 2004). Sediments filling the basin are largely Eocene clastic deposits, with Cretaceous carbonates mappable at certain locations. The orogenic influence on the geologic structures in the Aínsa basin are observed easily, with the town located in a syncline bounded by the prominent Mediano (east) and Boltaña (west) anticlines (Kjemperud et al., 2004), and the thrust-related Peña Montañesa north of the town site (Figure 3). The basin fill is comprised of a succession from deep marine to fluvial sediments, which are observed in many outcrops. Each day of the course was dedicated to one of the three major depositional environments observed: deep marine, with a focus on turbidites; shallow marine deltas; and a blend of braided and meandering fluvial systems. These varied depositional environments allow for the study of several sedimentary structures and assessment of their suitability as potential subsurface storage reservoirs for carbon dioxide sequestration. It is of the utmost importance to understand these geologic features in outcrop so that predictions and models of injected CO2 plume propagation, potential fracture development, fluid leakage, and caprock integrity will be as accurate as possible.
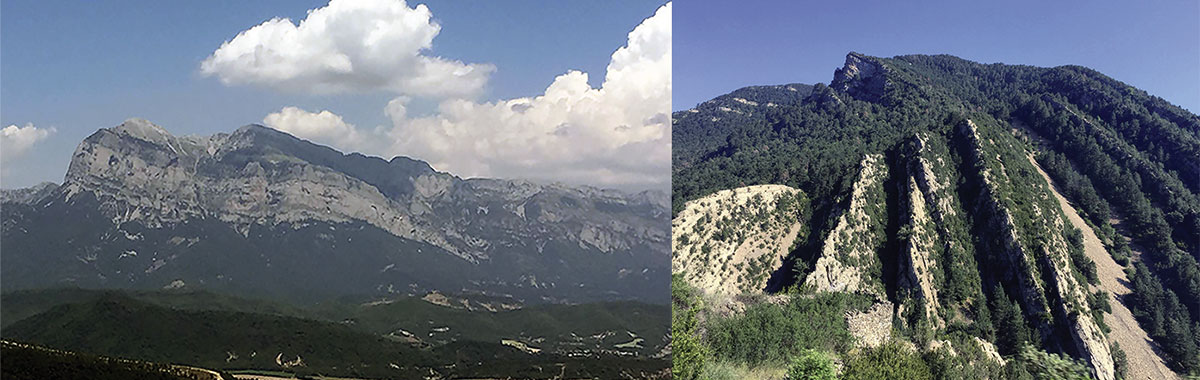
Deep Marine Deposits
The structure of the course focused on studying deposits from major depositional systems within the Aínsa basin, with the first day of the course examining deep marine deposits, particularly density-driven turbidite flows. Several outcrops and viewpoints were observed in order to study both the composition and the scale of these turbidite deposits, as well as to appreciate the significant impact of the Pyrenean orogeny on the structure of the basin. The outcrops were located close to Aínsa and near the town of Fiscal, approximately 25 kilometers northwest of Aínsa. Near Aínsa, sandy turbidite deposits were observed in large outcrops, showing the characteristic shape of a channel-like feature. The turbidite deposits were several hundred metres across, and could be correlated for short distances (up to 5 km). The sediments can be traced to their source: typically canyons approximately 10-12 km from Aínsa. Additionally, the turbidite channels were observed to be stacked, with some potential for connectivity if a younger channel had eroded into an older one. Interbeds of fine sandstone or shale separate these turbidite channels. At a smaller scale, the turbidite flow channels were observed to fine upwards from well-rounded pebbles at the base to mud or silt (Figure 4).
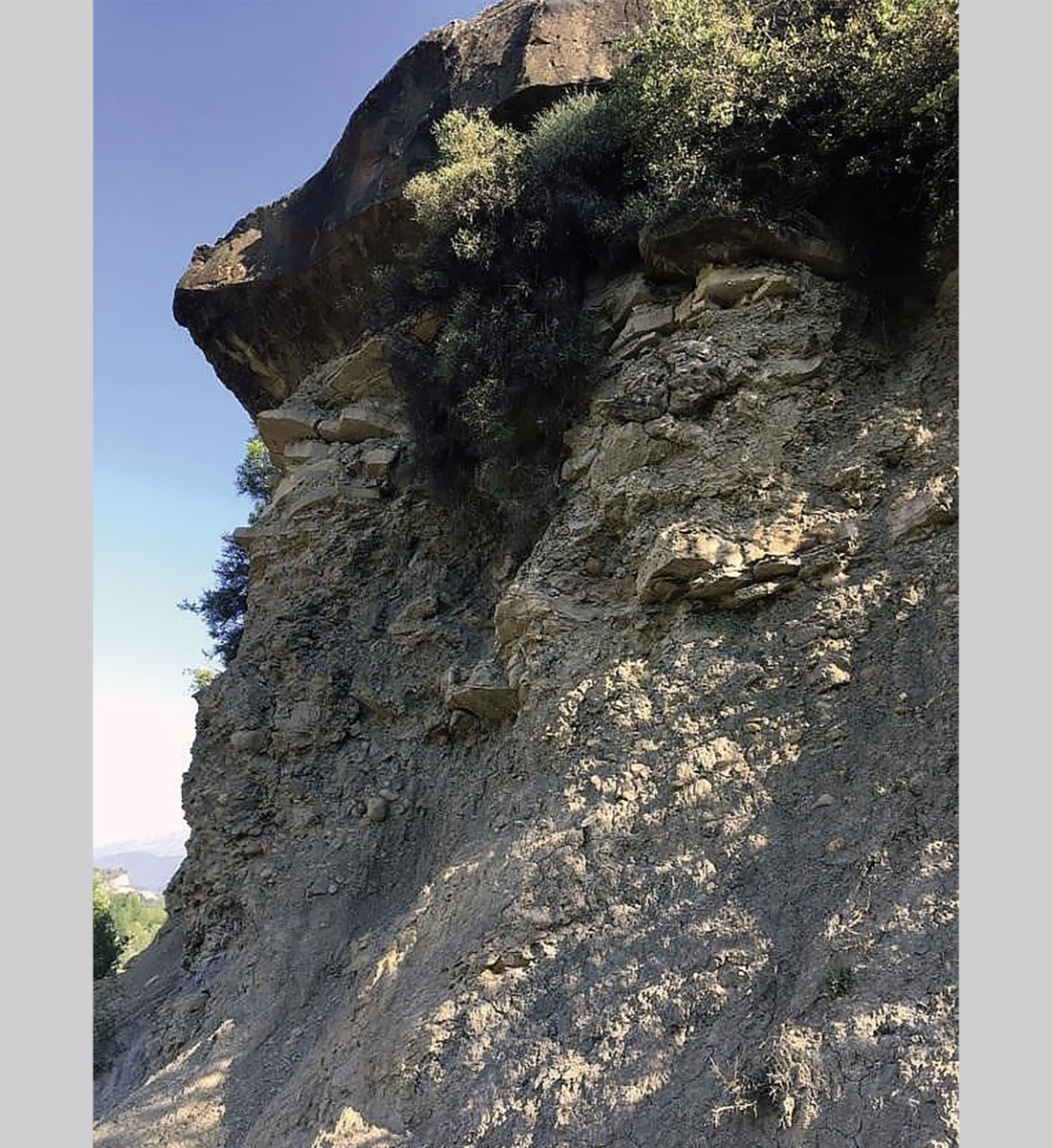
These observations have significant implications for CCS as isolated sand bodies, separated by impermeable shale deposits, will be less attractive storage targets than turbidite sands that have eroded into each other and stacked, increasing the potential storage volume. Understanding the composition of these deposits is also important, as the amount of coarse sand and conglomerate present in the turbidite channels will affect the porosity, and thus the potential storage volume, of the sand body.
The final location of the first day was near Fiscal, and provided an extensive and detailed example of a partial Bouma sequence in turbidite deposits. Layer A of the sequence was easily interpreted, with massive sandstones up to 1 m thick observed over a distance of several hundred metres. These massive sandstone bodies, with no discernible internal structures, were also observed to have eroded into underlying beds of previous sequences. Bouma sequence Layers B and C were also observed, with laminations and ripples evident. In this outcrop, Bouma layers D and E were not observed, likely having been eroded away by subsequent turbidite flows. Understanding Bouma sequences is important for CCS as the presence or absence of layers D and E, which are typically lower porosity and permeability, plays a significant role in modelling the connectivity of more porous sand bodies.
The marked differences in CCS reservoir quality between turbidites with layers of fine sediment, such as D and E Bouma layers, and those without highlight some of the limitations of seismic measurements in characterizing possible CCS reservoirs. The gradation in turbidite sequences from ideal coarse sediment to problematic fine sediment was frequently observed at Aínsa to take place at scales of less than 1 m. Major implications for reservoir quality may then lurk below achievable seismic resolution. This emphasizes the importance of reliable geologic understanding to complement seismic measurements. It also suggests the promise of using non-conventional seismic analysis. Characterization by stratigraphic filtering, for instance, may contribute to understanding of layering at sub-resolution scales, and thus provide important information about turbidite deposits.
Shallow Marine Deposits
The second day of the course focused on shallow marine deposits, with a particular emphasis on deltaic sands. Three outcrop sites were visited, each allowing the participants to observe depositional structures of deltaic sediments at various scales, and understanding of how these deposits interact within the larger depositional system. The outcrops were located near Roda de Isábena, approximately 60 kilometers southeast of Aínsa. The first viewpoint was an excellent example of prograding deltaic lobes, and how various features arise from changes in the depositional environment. As shown in Figure 5, we easily observed that beds near the bottom of the section dip towards the right of the photo, representing the progradation of deltaic sands from the shoreline towards the shelf edge. Additionally, the dip direction of the sandstone beds in the outcrop changes from the bottom to the top of the section, interpreted to be a result of a change in the progradation direction of these deltaic lobes.
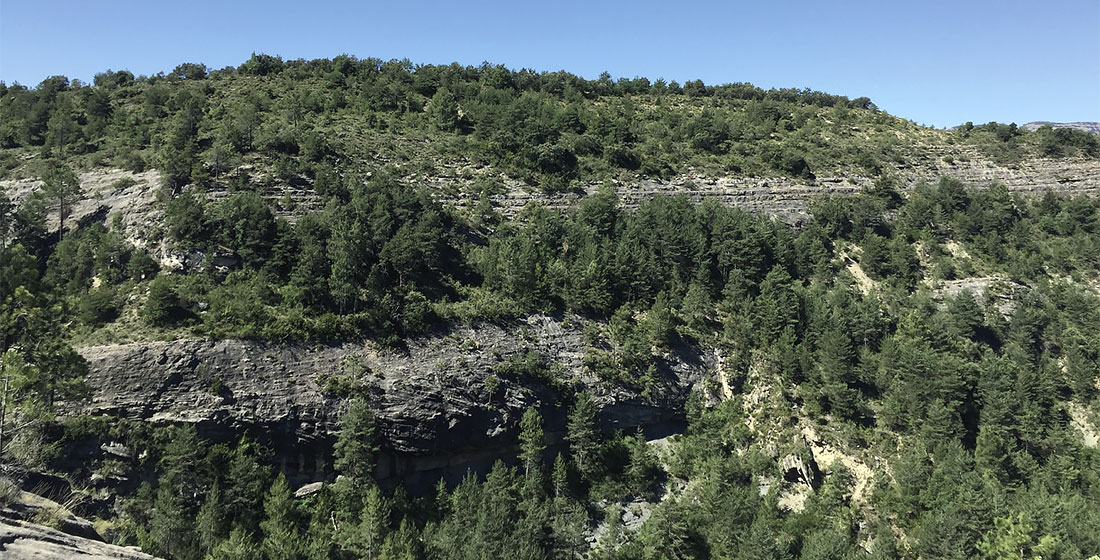
A crucial implication for CCS in deltaic deposits is the potential connectivity between lobes and also between the larger packages of these sand deposits. An important control on permeability between packages is the formation of calcite-cemented nodules between lobes. Thinner calcite nodules between lobe deposits may act as baffles to fluid flow, or more significant barriers if widespread cementation between nodules develops. Calcite cemented nodules also have considerably higher bulk modulus and density than the surrounding sandstone. The consequent change in impedance can provide a significant reflection coefficient for propagating seismic waves, thus making reflection seismic a useful tool in characterizing deltaic sands for CCS. The nodules, observed in the outcrop photo and at the photographer’s location, are typically 1 to 2 m in diameter, with poor connectivity.
The second and third locations of the day were located to the south of the first outcrop, and allowed for the study of deltaic deposits at both very small and very large scales, respectively. The second outcrop, located in a modern river valley, displayed dune-like deposits which reflect the daily cycles of sedimentation. Larger sand deposits, up to 20 cm thick, were observed cutting into underlying strata, and were separated by thin mud layers up to 2 cm thick. The alternating sand and mud deposits are indicative of daily fluctuations in the energy level of the depositional system, and allow for conclusions to be drawn regarding the connectivity between sand bodies. The presence of thicker mud layers between sands would have had a significant effect on the potential of these deltaic lobes to act as storage reservoirs for CCS. As in the case of deep marine turbidites, these sub-resolution effects can have major consequences for the suitability of the deposit for CCS.
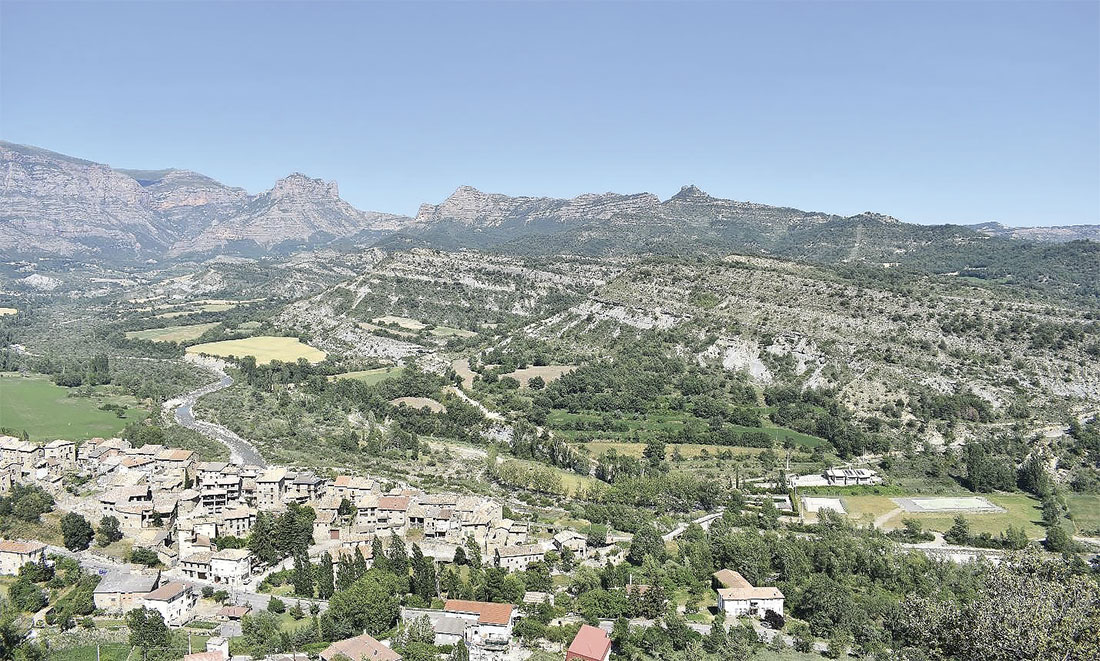
The final location provided context for the role and extent that deltaic deposits have within the larger depositional system. Figure 6 shows a picture of an outcrop of deltaic sands directly across the river valley, dipping towards the right; these are directly correlated to the lobes observed at the first outcrop. The regional depositional system can also be inferred, as the base of the mountain in the centre-left of the background represents the paleo-shoreline, and is comprised largely of conglomerates.
Fluvial Deposits
The final day of the course, compressed into a half-day session due to extreme heat, examined the depositional features associated with a combination of meandering and braided fluvial systems. The two outcrops were located near Olsón, approximately 25 kilometers south of Aínsa, with one outcrop displaying stronger meandering influence and the other more of a braided system.
In one of the outcrops, conglomerate bodies, with large clasts up to 10 cm in diameter, were readily observed. The higher porosity conglomerates were separated by medium to fine-grained sandy lenses, as shown in Figure 7. This outcrop is interpreted to show gravel bars, up to 2 m thick, separated by sand-filled channels in a braided fluvial system. The two most important implications for CCS in a braided system are the connectivity within the conglomerate bars, and the ability of the finer-grained material to act as a seal. As observed in the photo (Figure 7), the bars stack and migrate laterally, due to migration and reworking of the channel. It is possible that high-energy events that deposited these bars may erode into underlying bars, potentially increasing the connectivity between these bodies and thus increasing the available storage volume. An additional concern for CCS in braided systems is the presence of the seal in the form of the finer material. While the sandy deposits may be impermeable, with silt and fine sand present, the deposits may not be extensive enough to adequately perform as a sealing unit.
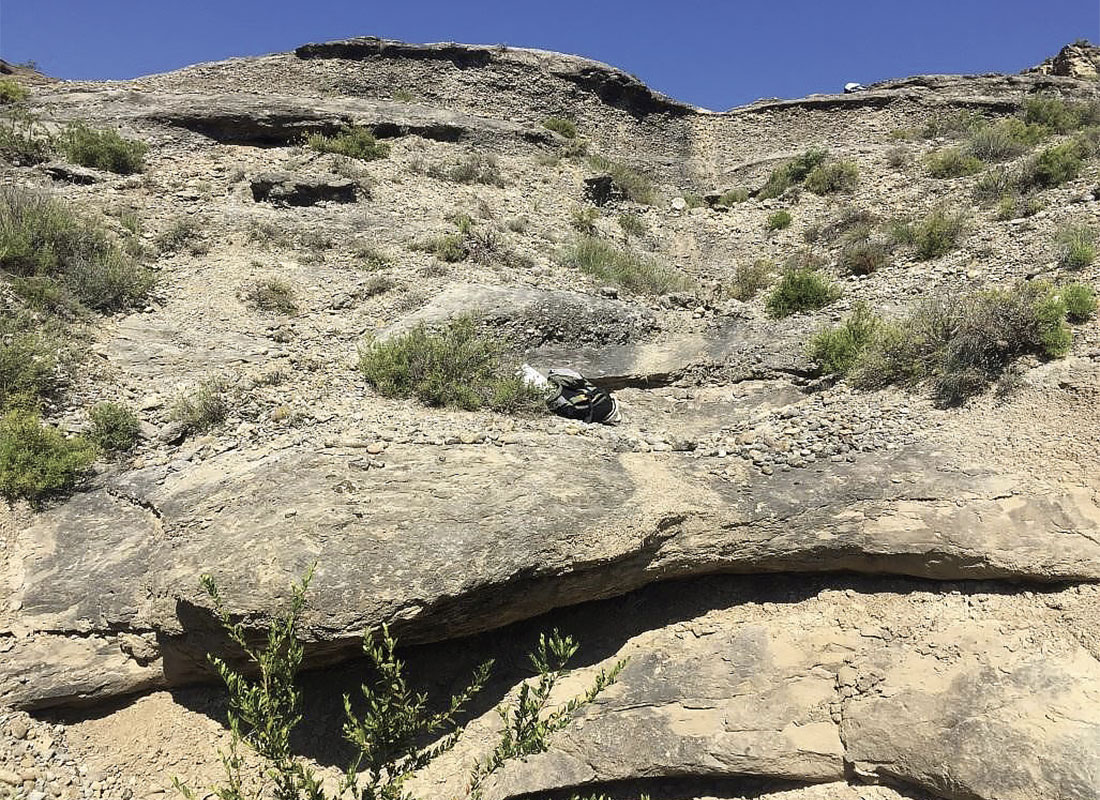
In addition to studying outcrops from a braided-dominant fluvial system, point bar deposits from a meandering-dominant system were also observed. Between the host shale and sandy point bars, an erosional surface overlain by a layer of pebbles, up to 10 cm in diameter, was observed. This is interpreted to represent a transition from a high energy environment to a lower energy environment. Within the large sand body, distinct layers of sand were observed to be dipping in the same direction, interpreted to be a point bar, building outwards into the channel. The structure of this body can be seen in Figure 8, with the internal beds of the point bar dipping towards the left in the photo.
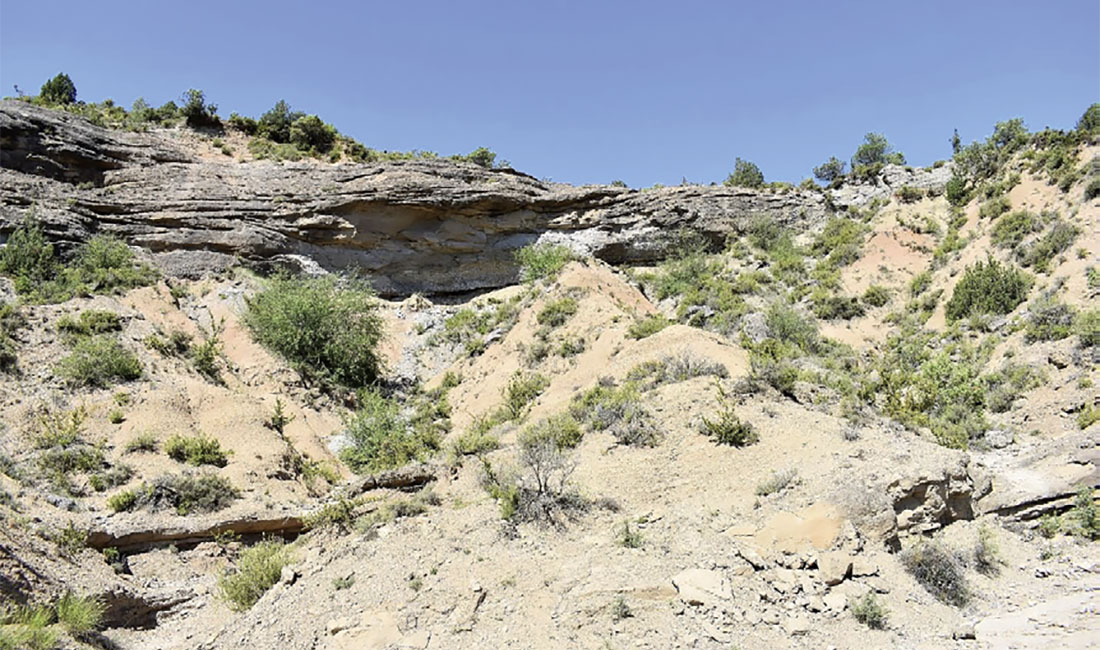
Percolation theory was introduced in an evening classroom session to help address the issue of connectivity between bar deposits in the absence of large scale 3D seismic volumes. This theory provides a statistical relationship between the fraction of “conductive” material in a medium and the probability of one conducting region being connected to infinitely many others by a conducting path (Shante and Kirkpatrick, 1971). This probability usually assumes a value very near to zero or unity, with an abrupt transition region. By understanding the high permeability sands to be the “conductive” elements of the CO2 sequestration system, this allows for a simple characterization of the probability that a reservoir is truly sealed in a 3D sense.
Summary
This field course offered an introduction to sedimentary depositional environments for non-geologist graduate students working in related fields. It also provided contacts and a basis for future collaboration between students from the three participating universities. The relationships between the sedimentary structures observed and applications to CCS were emphasized on this course. From a geophysicist’s perspective, many important challenges for CCS were highlighted, especially the importance of sub-seismic resolution layering in determining the quality of potential reservoirs.
As part of Project CARBEOR, Calgary-based CMC Research Institutes will host a summer field school in 2018 at the UCalgary-CMC Research Institutes Containment Monitoring Institute (CaMI) Field Research Station, in Newell County in southern Alberta.
Acknowledgements
We would like to thank the University of Calgary, NTNU, CMC Research Institutes Inc and Don Lawton for providing us with this opportunity and organizing our participation in the field course. The instructors for the course: Martin Landrø, Philip Ringrose, Cai Piug de Fabregas, Allard Martinius, Ole Torsater, and Sverre Ola Johnsen have our sincere gratitude for making the course such an instructive, informative, and fun experience.
Join the Conversation
Interested in starting, or contributing to a conversation about an article or issue of the RECORDER? Join our CSEG LinkedIn Group.
Share This Article