Summary
Extracted post-stack seismic attributes from time-lapse 3-D seismic data have been used extensively to map steam areal conformance by using statistical analysis in the development and monitoring of follow-up processes at Imperial Oil’s Cold Lake production project.
A quantitative cross-calibration scheme between time-lapse seismic surveys has been developed using data normalization. It was found that a significant difference results from using a different calibration set; and that time-lapse data cross-calibration provides better, more stable and consistent results.
Attributes from principal component decomposition of seismic data compensate for the redundancy of conventional seismic attributes in statistical analysis and provide better well-to-seismic ties when a full set of principal component attributes are used.
Vertical steam conformance can be established through poststack inversion to provide 3-D visualization of the impact of lithologic control on steam distribution and steam migration pathway. The vertical conformance reveals that sequence architecture has a significant influence on steam migration. A sequence with high mud content tends to limit or slow both vertical and lateral migration of the steam chambers.
In pre-stack efforts, the functional relation of P- to S-wave velocities, called the mud-rock line, plays an important rule in extracting S-wave information from pre-stack data. An optimized mud-rock line for Cold Lake clastic rocks is an exponential relationship between P- and S-wave velocities. With an optimized mud-rock line and high frequency seismic data, the inverted pseudo S-wave from AVO analysis has much higher resolution than the P-S wave data. The reservoir heterogeneity can be well-defined by fluid factor alone. The application of this technique to reservoir monitoring defines steam channels and identifies lithologic barriers within the reservoir.
Introduction
The Clearwater Formation was deposited during maximum transgression of the Mannville Group. The Clearwater Formation at Cold Lake is about 420 m below the surface. The gross thickness of oil sands is from 40 m to 75 m. The average net pay is about 45 m. The bitumen weight percentage saturation varies from 0 to 12% with an average of approximately 10%. The reservoir’s average porosity is 32%. The permeability varies from 0.5 to 4 darcies. The bitumen viscosity at reservoir pressure and temperature is rather high ranging from 104 to 106 cp. Since heat can be used to reduce bitumen viscosity, cyclic steam stimulation (CSS) is one of the techniques that can be used to realize bitumen production. Conceptually, the CSS process has three phases: steam injection phase (steam injected into the reservoir), soak phase (steam and condensed water heat the viscous oil), and the production phase (heated oil and water flow are pumped to the surface). The steam chamber around the wellbore is subdivided into three zones: the heated zone, transition (impedance) zone, and cold zone. Late cycle CSS reaches its economic recovery limit due to a decline in OSR (Oil-Steam-Ratio). The Follow-up Processes (FUPs) program is targeted at increasing economic recovery at Cold Lake beyond the 25% average recovery expected with CSS. This increase will be achieved through infill drilling. The first follow-up process implemented at Cold Lake was the BB/W injector-only-infill (IOI) pilot, started in late 1988. Findings from this first FUP have led to further IOI piloting at B2/B5 pads and a horizontal injector, M-H1, also at BB/W. FUP pilots involving horizontal well producers have been piloted at AA, B03, and C03 pads. Horizontal-injector-producers (HIP) build on the findings of all previous FUP pilots that have been implemented. Seismic waves can recognize the steam zone for three major reasons. The most critical reason is that natural gas (CH4 or methane) evolves from bitumen when the reservoir pressure drops below its bubble point at a particular temperature (2 to 3 MPa). The percentage of gas does not have to be high. In particular, the P-wave velocity drops from 2300 m/s in oil sands with 0% gas saturation to 1850 m/s with 2-5% gas saturation (e.g. foamy oil). Increasing the rock temperature also decreases the rock velocity. In the situation of high temperature and low pressure water vapor exists in the heated zone, which causes a seismic response similar to gas.
Three key seismic attributes were used for the monitoring of reservoir bitumen recovery in the early stages of this technology development (Eastwood, 1994). The delay time (sag) is generated by mapping the relative time difference of reflections from horizons above and below the reservoir between repeated (timelapse) surveys. The amplitudes of horizons above, below and within the reservoir between repeated (time-lapse) surveys were also used for the monitoring. Frequency attenuation within the reservoir is the third attribute used for monitoring. Statistics such as discriminant analysis for a large number of attributes analysis were introduced (Eastwood, 1996). Isaac (1996) further combined 2-D compressional-wave and converted shear-wave with 3-D seismic data to illustrate that Vp/Vs can be used to distinguish heated from cold reservoir. Her amplitude-versus-offset (AVO) analysis confirmed the amplitude anomalies were caused by low velocity intervals (heated reservoir).
To achieve this project’s objectives, we need to: (1) carry out time-lapse data normalization and develop the concept of cross-calibration in statistical analysis of time-lapse data, based on interval attributes analysis; (2) develop non-redundant attributes - principal component attributes and compare with conventional attributes in statistical analysis; (3) discuss 3-D steam conformance and influence of sequence strata via post-stack inversion; (4) develop localized mud-rock line and conduct time-lapse AVO inversion and AVO inversion for reservoir heterogeneity study.
Cross-calibration and normalization of time-lapse data
Discriminant analysis of seismic attributes is a valid algorithm for steam conformance mapping (Eastwood, 1996). The infill drilling results correspond well with the seismic interpretation. Figure 1 shows the core analysis results and formation temperature log from two infill wells (B5-33 as a hot location and B5-28 as a cold location) at B5 pad; and both wells are compared with original CSS well B5-08 that was drilled in 1985. A significant drop of oil saturation is shown at the hot location (Figure 1a). The temperature can reach as high as 140 (C in the production phase. However, the difference of oil saturation at cold location is small (Figure 1b). The temperature was about 25 (C as the highest in the formation. The repeated survey was conducted in late 1997 to understand steam conformance in this new phase and further evaluate IOI (Injector- Only-Infill) steam injection performance. The ultimate goal is to tie the production data with seismic results by comparing pads with infill drilling and without infill drilling. In the past time-lapse seismic surveys were analyzed independently. By comparing two independent results a conclusion about reservoir dynamic change can be drawn. This approach is challenged when the questions rise: How consistent are the results between the two surveys? When the later reservoir status is unknown or partially unknown can cross-calibration build up the bridge to obtain new parameters?
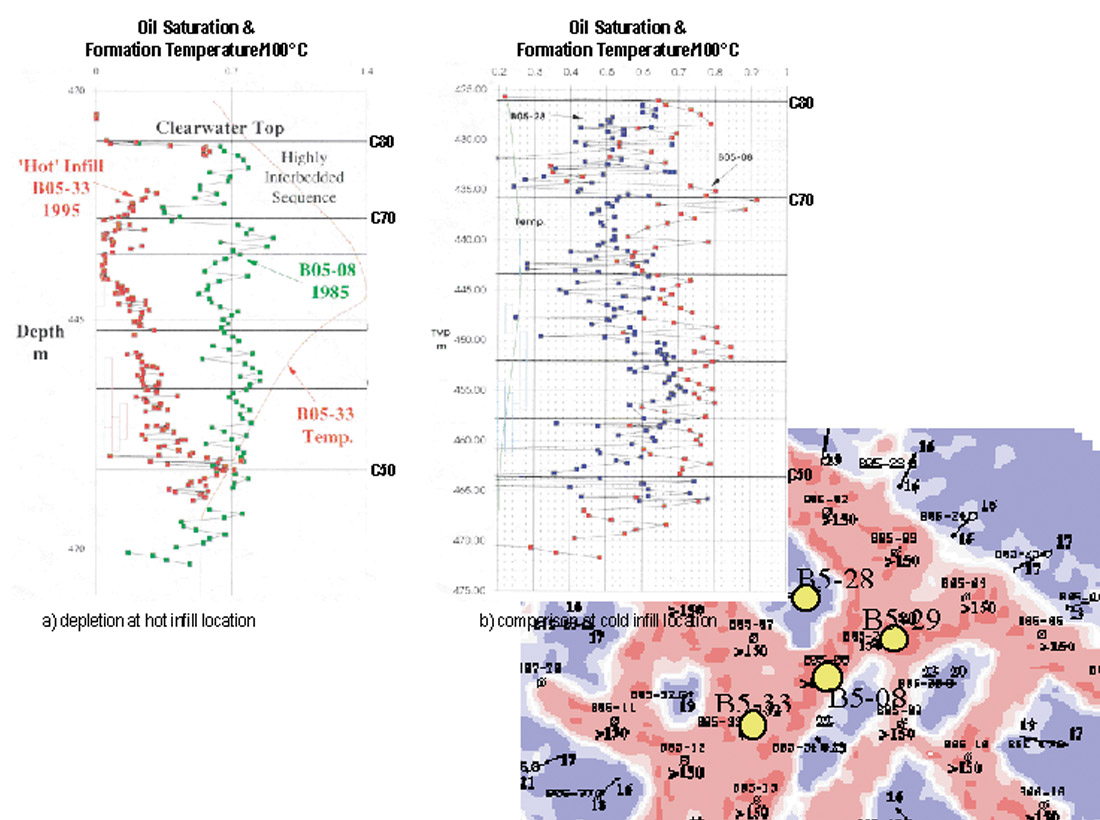
Cross-calibration can also be called cross-training as the process is part of statistical analysis. This novel approach uses the ‘95 (pre-IOI) well calibration set to train ‘95 seismic data (attributes) to obtain a training set. This training set is then applied to the discriminant analysis of the ‘97 seismic data. The seismic attributes training set of survey A is applied to the discriminant analysis of survey B. This process is called cross-calibration or cross-training. This process brings consistency to time-lapse seismic analysis. Therefore, it may provide consistent results in terms of reservoir status, and parameters. It may provide a means for reservoir prediction. In this case the reservoir status of ‘95 is known, and seismic data is available for ‘97. The unknown is the ‘97 reservoir status. To make this process work, time-lapse seismic data normalization is required. The time-lapse seismic data normalization can be implemented in a number of different ways:
- Whole survey match operator – average filter of all filters that are derived from each post-stack CDP trace pair between surveys, both phase and amplitude are adjusted between surveys.
- CDP-to-CDP match operator – a single filter is extracted from each CDP pair. Data normalization is implemented on the post-stack trace base. Both phase and amplitude are normalized for each trace, which may be different.
- Shot phase match operator – filters are derived to match the phase of each trace of a shot gather of survey A to its correspondent stack CDP of survey B. Only phase is normalized.
- Shot phase match plus CDP-to-CDP match operator – combination of above operations 3 and 2.
- CDP-to-CDP gain match operator – a filter is extracted for each CDP traces pair but only amplitude adjustment is applied.
- Multi-trace operator – one filter is derived from a block of CDP traces and applied to the whole survey data normalization.
A number of rules were followed when a filter was designed. These rules include:
- The section used for the filter design should have minimal or no acquisition footprint. With current acquisition geometry, seismic artifacts (acquisition footprint) appears at about 200 ms (200 meters in depth) and above on the section in Cold Lake 3-D seismic data.
- Focus on the data portion where the best repeatability is found.
- Exclude the reservoir portion where changes occur from survey to survey.
- Use the information above the reservoir rather than below the reservoir as changes which occurred in the reservoir between surveys may affect the seismic section below it, except special situations such as Cold Lake, Alberta where the reservoir is very shallow (around 420 meters to 470 meters). In this case a part of the seismic section a certain distance below the reservoir is used.
- The operator should have reasonable length and cover at least a few wavelengths so that it does not pick up the local geology.
The concept of cross-calibration is first applied to the analysis of B2456 time-lapse survey data sets. Figure 2 shows cross-calibration comparisons of pre- and post-IOI steam conformance without (Figure 2a) and with (Figure 2b, 2c, 2d) different timelapse data normalization. In the analysis, pre-IOI (‘95 survey) attributes were trained with pre-IOI (‘95 well condition) calibration set, then applied to the discriminant analysis of post-IOI (‘97) survey. Comparing these results with independent analysis it was concluded that cross-calibration provides reasonable and consistent results and post-stack data normalization is as good as pre-stack data normalization.
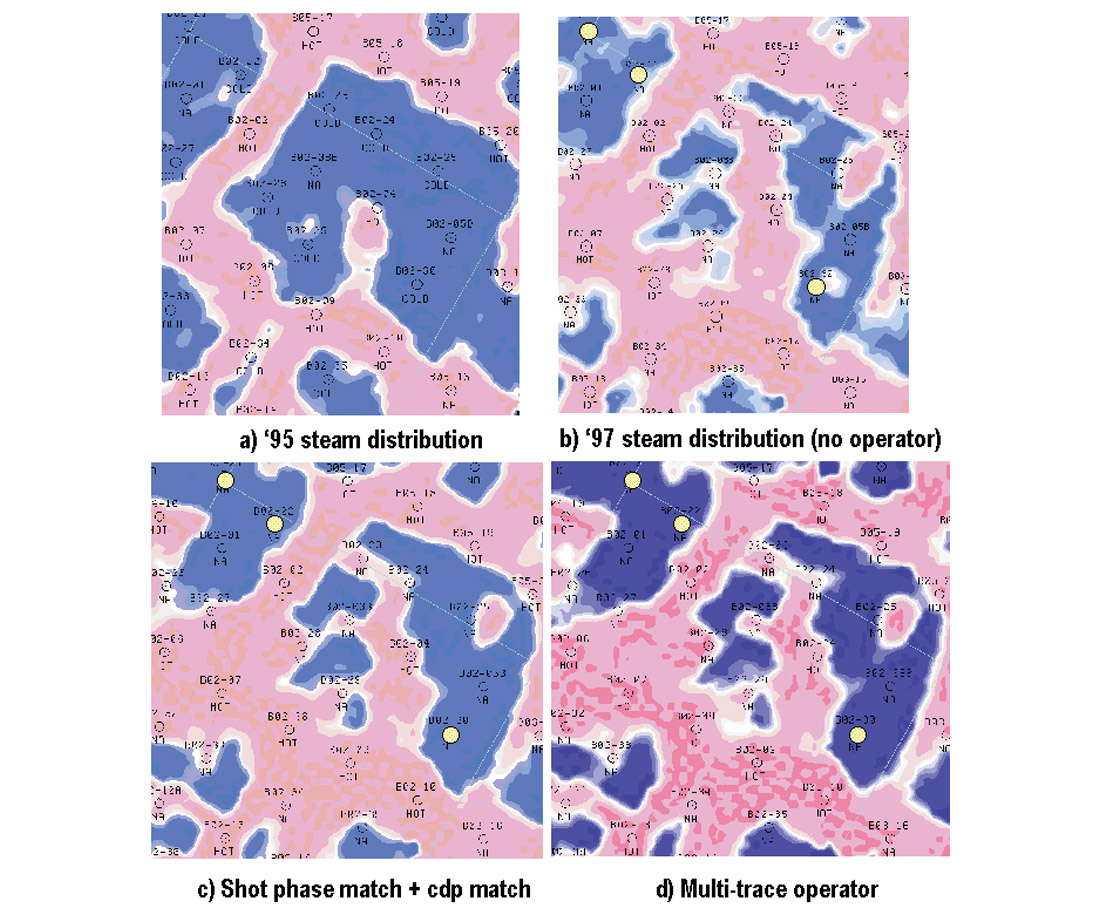
Non-redundant attributes: principal component attributes
Conventional seismic attributes, such as average amplitude, isochron, peak amplitude, central frequency, etc., are readily extracted from a data volume and can be related to reservoir properties in many instances. However, these attributes do not necessarily capture the entire variance of the reservoir property of interest. As well, these attributes have some degree of correlation, and thus, analysis techniques must be able to compensate for the redundancy.
In contrast, seismic attributes calculated from principal component decomposition of the seismic data are not correlated (Figure 3) and the full suite of principal components contain all the variance in the seismic data (Hemon and Mace, 1978; Fournier and Derain, 1995). Perceived disadvantages of principal component attributes are that they are data set dependent and are not directly related to physical properties.
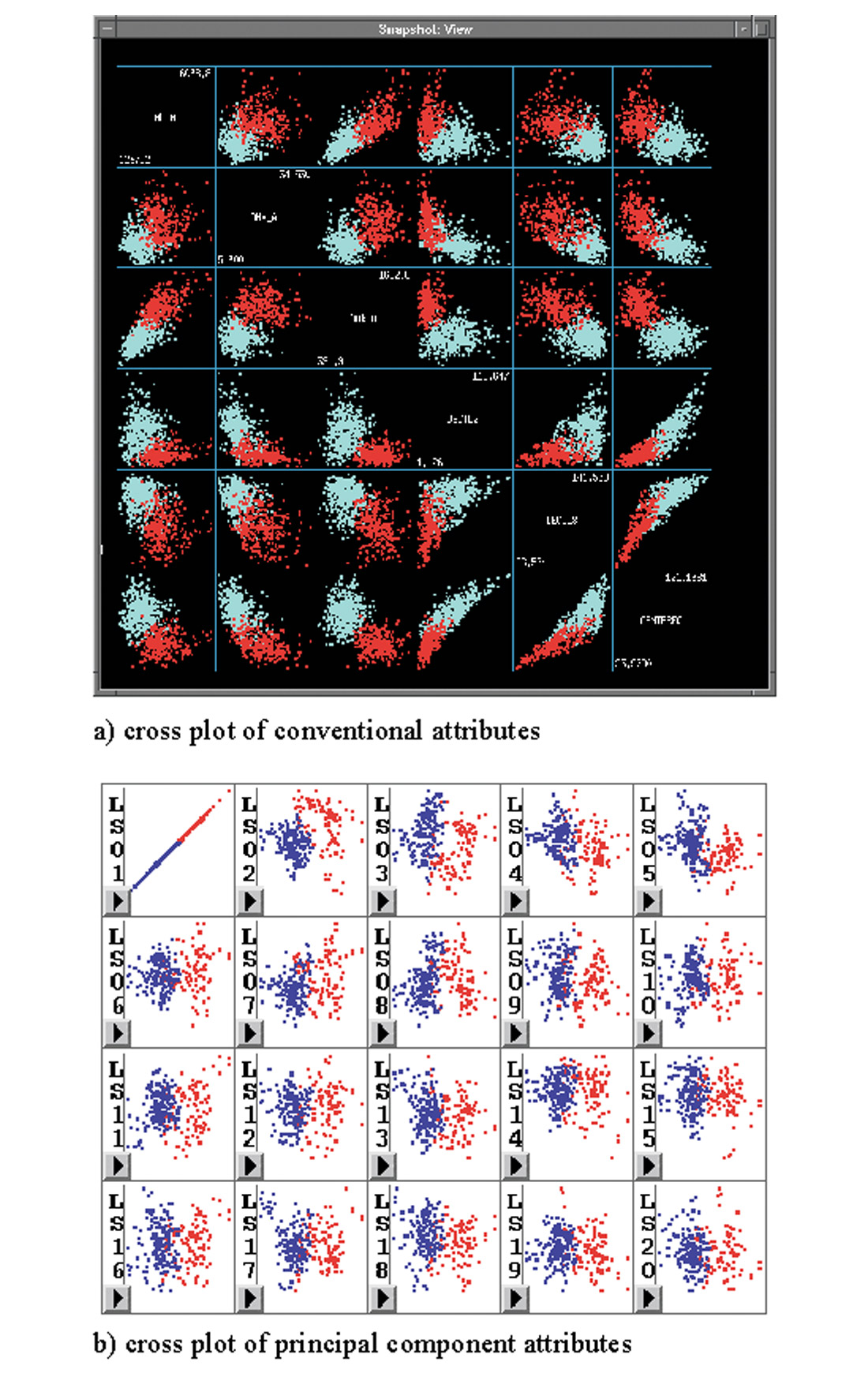
The principal component attribute analysis at the A02A04 seismic monitoring project produced better results than the conventional attribute analysis. In particular, the discriminant function provided an 89% correlation with well control, and the areal conformance was better correlated with the engineering pressure communication data. Finally, the spatial coherence of the mapped features is higher with the principal component attribute analysis than with the conventional attributes.
Three horizontal wells were drilled recently as infill wells to the survey area to enhance the recovery (Figure 4, well path denoted in green lines). Seismic probabilities and resistivity logs of well D03-H3 are plotted in Figure 4. The high probability (>0.5) locations are the steam chambers intersected by the horizontal well, where the resistivity is low (<10 ohm) as the reservoir is depleted and water saturation is high. It is indicated that principal component attribute results in a better fit to resistivity than conventional attributes.
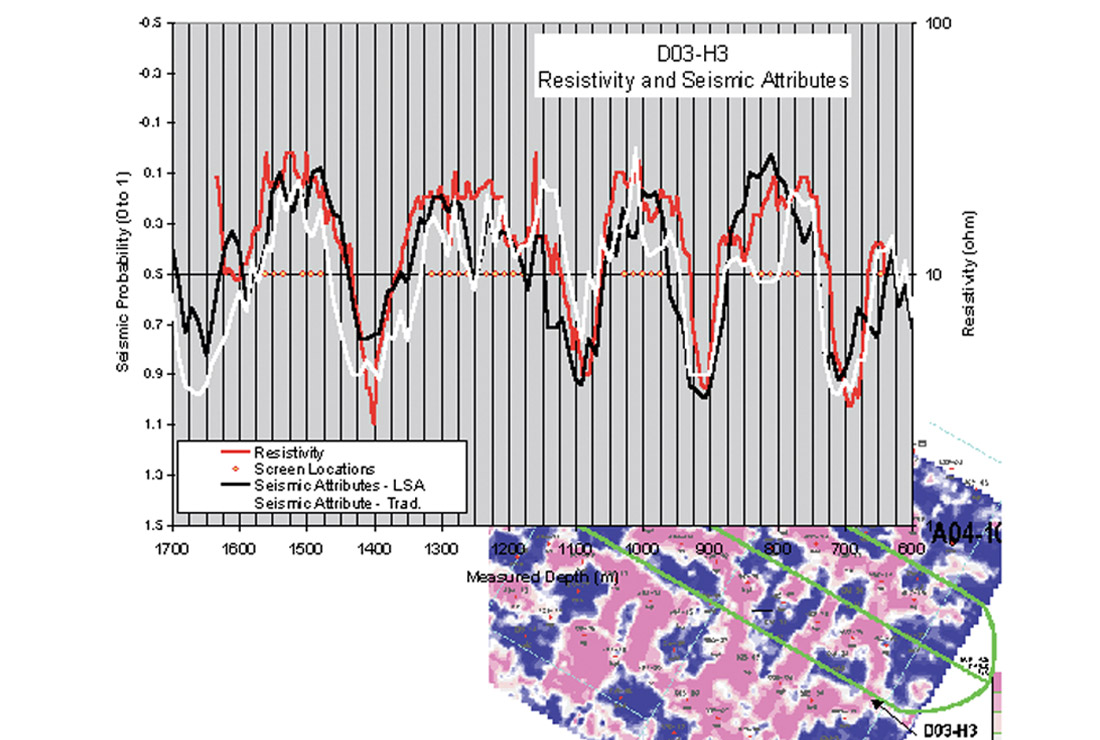
The principal component attributes are not directly related to the physical properties (no physical meaning for each attribute), yet, they fit well to the Cold Lake steam conformance mapping, as it is a multi-variant analysis. Perceived disadvantages of the principal component attributes are that they are data set dependent and they are not applicable for single attribute analysis. If time-lapse data sets are decomposed independently, cross-calibration of time-lapse attributes may be invalid.
3-D steam conformance and influence of sequence strata
A steam conformance map provides important input in terms of infill location and steam performance evaluation. Steam conformance in 3-D space would be the next potential advancement. In particular, mapping of steam conformance in the vertical dimension will provide insights into the rate at which steam moves vertically in the reservoir and the impact of lithologic influence on the heated zone or steam chamber. From a process perspective, this data will facilitate the determination of the relative impact of gravity drainage and fracturing on the growth of the heated zone. From a practical perspective the results will directly impact the selection, design and operation of follow-up processes.
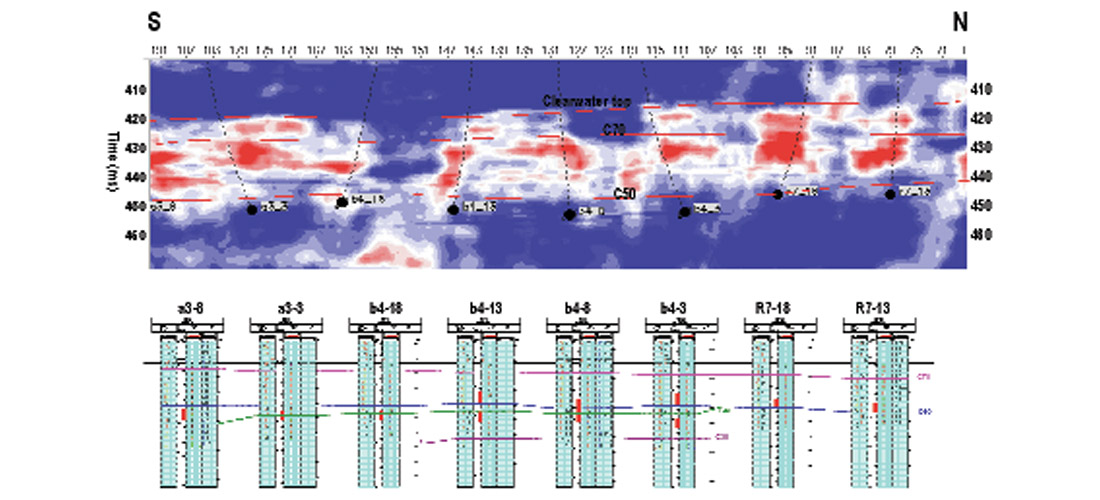
In fact, the steam barrier discussed above is the C70 sequence boundary within the Clearwater formation. The upper part of the C70 is good quality reservoir while the lower part gets very shaley. This shaley boundary may not have enough impedance contrast to act as a strong reflector on P-wave data. However, due to the steam disturbance above and below this boundary it can become a good reflector. The major reservoir unit at Cold Lake is sequence C50. Of sequences C30, C40, C50, and C70, the C50 is composed of the cleanest sand. The sharp lower boundary of the steam chambers corresponds closely to the C50 sequence boundary. Apparently the mud content of the C30 and C40 prevented significant steam penetration. In the area where C50 is the dominant reservoir, steam usually breaks through some of the barriers such as thin shaley boundaries, in the later cycles of CSS. Steam chambers exhibit a characteristic blocky appearance on vertical conformance plots, as seen in Figure 5. Sequence boundaries may be damaged and not show reflections. However, in the area where C50 is reduced, steam chambers are restricted locally and areal communication channel networks are in the early stage. Sequence boundaries are preserved. The C70 overlies the C50 in the study area. This sequence is muddy at the base and sandy at the top. The muddy interval was a weak impediment to steam migration, but steam still penetrated the upper portions of the sequence in some areas. Cross-line 138 is a section crossing the B4 and R7 pads in a north-south direction. Apparently, the C70 sequence boundary acts as a steam communication barrier between the major sequence C50 and upper C70. A detailed sequence stratigraphy cross-section is built using well logs along cross-line 138. Shown in Figure 5 is the comparison of a well log cross-section with sequence boundaries mapped and the vertical steam distribution shown. The vertical steam texture confirms sequence boundaries defined by well logs. The general trend of C70 sequence thickening towards the north can be observed on the vertical conformance texture.
Pre-stack (AVO) inversion and reservoir heterogeneity
It is usually difficult for a compressional-wave (P-wave) to differentiate shale from a non gas-bearing sandstone as the impedance contrast between them is not high enough. Figure 6 shows the cross plot of P-wave (Ip) and S-wave impedance (Is) from well OV11-17. It is seen that both shale and permeable sands have similar range of P-wave impedance (Ip). However, their shear-wave impedance is different enough to enable us to separate shale from permeable sands. This is one of the key motivations for a shear-wave study. Also, a better understanding about reservoir fluid can be obtained by combining S-wave with P-wave data, as S-waves do not respond to fluid compressibility changes, hence they respond only to the changes related to rock matrix and density.
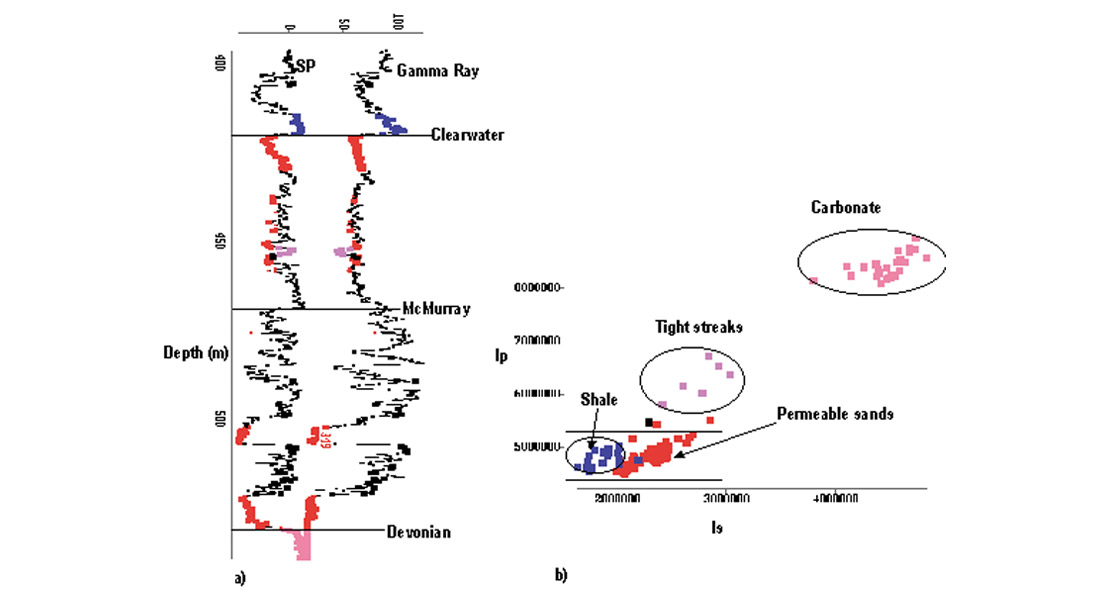
A critical factor that affects AVO inversion results is the mudrock line. A localized mud-rock line usually provides more accurate estimation for shear-wave information than a general one. We conduct an iterative process in AVO inversion. After the first iteration a pair of P-wave (Ip) and S-wave (Is) impedances is obtained. The Vp/Vs ratio for the next iteration can simply use the relationship Vp/Vs=Ip/Is. This iterative procedure keeps updating the Vp/Vs ratio and thereafter improves the ray tracing result () and gives a better estimate of Vp/Vs ratio. The input data for AVO inversion can be a CDP gather or a gather of limited angle stacks or a gather of limited offset stacks. The advantage of using a CDP gather is that no effect of averaging has been introduced into the inversion. But the data could be noisy, and it does not honor the fact that the wavelet may change with the ray angle. It may be computationally intensive, as inversion of each trace in the CDP gather requires ray-tracing. The advantage of using limited angle stacks is that the stacked traces are cleaner, and wavelets are allowed to change from angle to angle. But it does involve more work to estimate the wavelets.
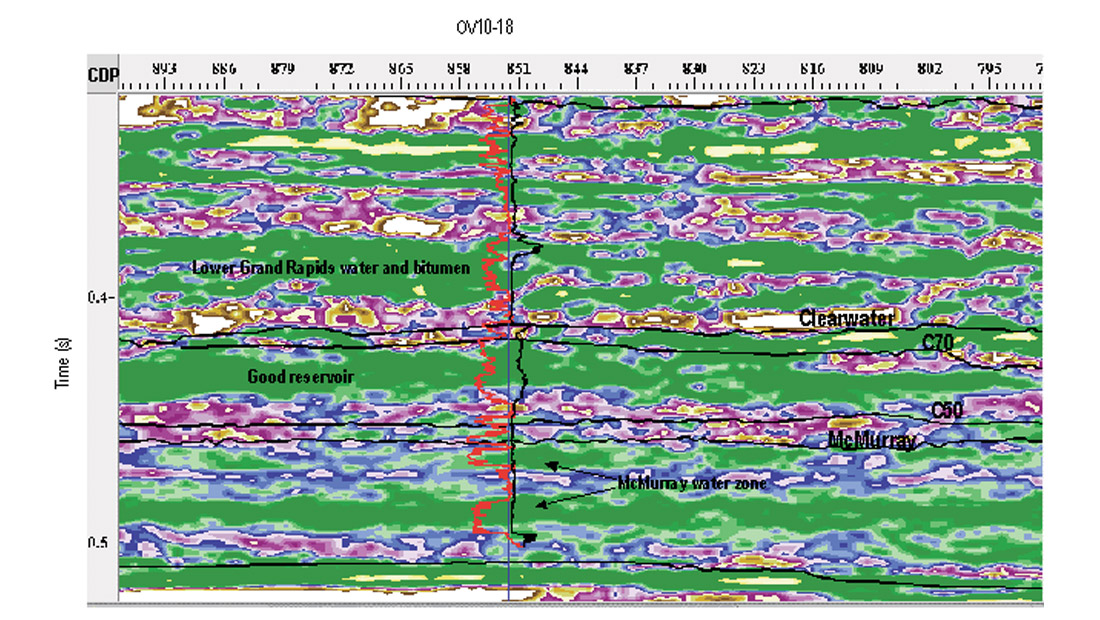
Shown in Figure 7 is the ‘fluid factor’ from AVO inversion, assuming the density is unchanged, which is true in the reservoir interval. Green and yellow colors represent good permeable sands which are either reservoir (high resistivity) or water zone (low resistivity). Blue and brown (including white) colors represent shale or muddy zones. The SP and resistivity logs of well OV10-18-65-3w4 are tied to the ‘fluid factor’ section. It is clear that the ‘fluid factor’ identifies good reservoir (some of C70 and upper part of C50).
Economics and summary
The operation of CSS may become uneconomical as it approaches late cycles. The oil-steam-ratio (OSR) gets very low. Time-lapse seismic assisted follow-up programs (FUPs) can usually lift up OSR, and turn around production within one cycle period. Figure 8 shows a comparison of production curves for pads with FUPs (green curve) and the adjacent pads without FUPs (gray curve). By April-May, 1998 the production with FUPs is 40% or more higher than production without FUPs.
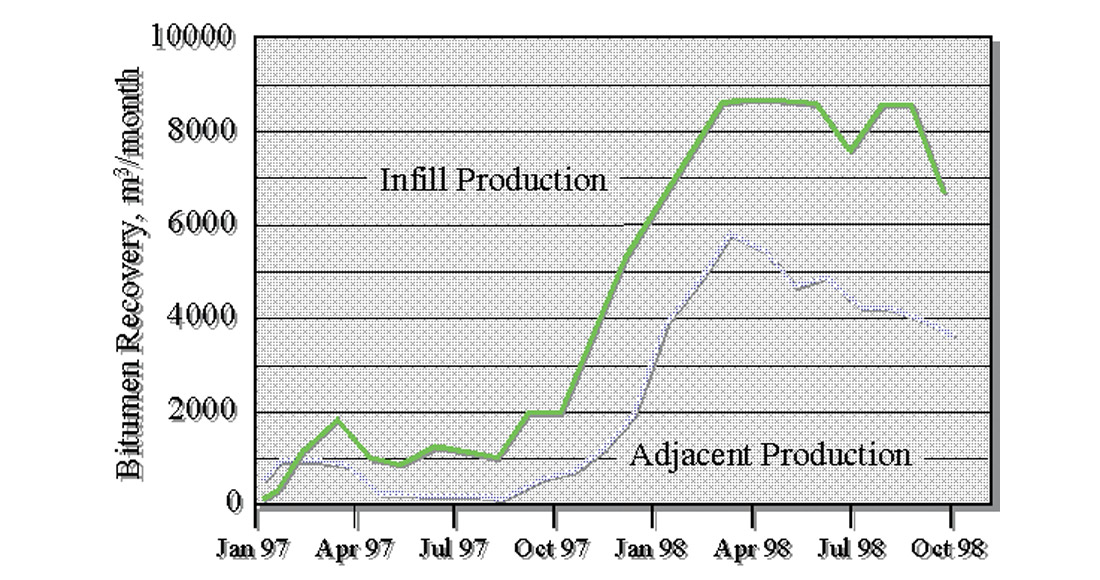
The work can be summarized as followings.
Based on statistical (discriminant) analysis of conventional seismic attributes, a quantitative cross-calibration and cross-training stream has been developed for time-lapse seismic data analysis using data normalization as a bridge. This cross-calibration or cross-training provides better, more stable and consistent results than independent training for reservoir monitoring. It was found that multi-trace operators derived from unchanged reservoir provide better data normalization.
To compensate for the redundancy of conventional seismic attributes, seismic attributes calculated from principal component decomposition of the seismic data are developed and used in the discriminant analysis for reservoir monitoring. These attributes produced better results than conventional attributes at some monitoring projects. To achieve this superior result, it was necessary to include a full set of principal component attributes. To minimize the dependence on the seismic data sets, time-lapse data sets were analyzed by merging two data sets together. The principal components were extracted from merged data sets, and attributes were calculated separately from individual surveys. A significant improvement has been made on time-lapse data analysis by applying this technique.
Since time-lapse data normalization is no longer required as wavelets are extracted independently from each data set, poststack inversion is applied to time-lapse seismic data sets of B2456. The vertical conformance reveals that sequence architecture has significant influence on steam migration. A sequence with high mud content tends to limit or slow both the vertical and horizontal migration of the steam chamber.
The functional relation of P- to S-wave velocities, called the mud-rock line, plays an important role in extracting S-wave information from pre-stack data. The mud-rock line established from in-situ P- and S-wave velocity measurements is the optimized for Cold Lake clastic rocks. It is an exponential relationship between P- and S-wave velocity. This study provided insights on the feasibility of using AVO inversion to differentiate shale from sandstone and reservoir fluid monitoring. The inverted P-wave is similar to the regular processed P-wave stack; it shows slightly higher resolution than, but is not as continuous as, the regularly processed P-wave stack. However, the inverted pseudo S-wave has much higher resolution than the P-S wave data. With an optimized mud-rock line and high frequency seismic data, reservoir heterogeneity can be well defined by fluid factor alone. The application of this technique to reservoir monitoring defines steam channels and identifies lithological barriers within the reservoir.
Acknowledgements
The author wishes to thank Dr. Rob Stewart for much advice in the course of this work. I appreciated many discussions with Dr. John Eastwood, Dr. Dave Johnston, and Mr. Glen Bishop. Imperial Oil Resources is greatly appreciated for allowing the publication.
Join the Conversation
Interested in starting, or contributing to a conversation about an article or issue of the RECORDER? Join our CSEG LinkedIn Group.
Share This Article