Summary
We review the developments in marine seismic technology over the past five years, and then use recent innovations to predict the next five years. Overall, most oil companies are beginning to embrace better acquisition planning as being a necessary foundation to overcome long-standing challenges to better seismic data. In particular, we review the innovations affecting better target illumination, improved removal of multiples and noise, higher 4D (time-lapse) repeatability, and better resolution. Each appropriate technology is then ranked as first order vs. second order significance.
Introduction
Franklin Institute Online cites Technology as being “The application of scientific advances to benefit humanity“. Application of seismic technology is about how all this is applied to provide a better end product to reduce drilling risk and improve reservoir recovery.
During the 1990’s 3D seismic exploration as a tool for large regional seismic exploration became de facto standard. In the last part of that decade, purpose built streamer vessels were deployed, more than doubling the number of streamers that can be towed behind a single vessel. The main drivers for technology development were operational efficiency; the cost per square mile of 3D seismic was reduced approximately by a factor of five from 1990 to 2000. The continuous improvements in computer hardware technology were quickly followed by the usage of better seismic processing algorithms. The improvements are evident on vintage data that is reprocessed with newer processing algorithms. Pre-stack depth migration became standard for GOM. Towards the end of the decade, 3D visualization tools became available for stereographic volume rendering. Applications such as interpretation and well positioning while drilling in an immersive visualization environment has proven to be successful in increasing drilling success. Industry confidence and awareness with so-called quantitative interpretation (QI) has blossomed, moving beyond mere AVO (amplitude versus offset) analysis to now routinely include stochastic elastic inversion and multi-attribute interpretation – quite often within visualization environments. Correspondingly, geoscientists are now asking for better data and more data types. Perhaps surprisingly, seafloor seismic has struggled to establish itself as a key technology, and continuous improvements in streamer seismic technology attract greater attention. Seafloor seismic became commercially available for the oil companies. This technology offered measurements of converted waves as a supplement to compressional waves, in addition to the ability to separate up- and down-going wavefields. Despite significant investments by service companies, and internal and external marketing from technology enthusiasts in the oil companies, 4C OBS is currently at best a niche acquisition technology.
Operational efficiency has been a key driver for technology development in seismic exploration during the 1990’s. This is still an important driver, but over the last few years we clearly see a trend where the oil companies more strongly emphasize the value of quality in the seismic product to enhance recovery and reduce risk where the cost is highest: drilling and production. In the North Sea, repeat (also called 4D) streamer seismic is more or less applied as a default to enhance production. Multi-Azimuth streamer surveys are being applied to improve image quality and reduce drilling risk, as the cost of seismic is demonstrably minor compared to drilling. Do we observe a paradigm change on how seismic is valued amongst oil and gas companies? With a continuous emphasis on seismic as a cost-effective way to improve ROI on oil and gas fields, we envision that significant overall improvements will arise in the next decade.
We present advances in new technology during the past five years connected with key challenges in seismic exploration. We describe how engineering and geophysical technology contribute to solve these challenges. It is not just improvements in specific technology that provide the optimal solutions, but also improvements along the complete seismic value chain: from survey design, through acquisition and seismic processing technology to the determination of reservoir properties and fluid flow models. New technologies have contributed to cost efficient solutions for difficult illumination problems, removal of multiples and noise, higher resolution, and better accuracy in repeat seismic signals.
Advances in seismic in the last five years addressing key challenges in reservoir exploration and exploitation
Any seismic project is best addressed by satisfying the strategy of “define the challenges, select the technology”. A large regional seismic survey to be acquired for exploration purposes has different objectives from a 4D seismic survey where a key objective is to minimize non-production related differences between the baseline and monitor surveys. Likewise, the key challenges to sub-salt illumination and imaging in the GOM are different to the masking of reservoir zone images by strong multiples in the Gulf of Suez.
We have addressed a set of key challenges below for many oil and gas field scenarios, and discuss how various advances in seismic technology are applicable solutions, including relevant examples.
Technology advances to improve target illumination in geology with complex overburden.
Seismic wave propagation through the Earth becomes complicated when rapid lateral variations in the geological (velocity) model exist above or near the target. In the worst scenario “multi-pathing” occurs and several seismic arrivals from the same interface are recorded at coincident surface locations. This will translate to degraded image quality, resolution and interpretability. Furthermore, many areas on the target interface may correspond to holes in seismic illumination, and only weak or scattered seismic energy may be reflected back to the surface. This will also correspond to poor or useless seismic images. Poor illumination is most common in the single vessel streamer scenario because a narrow range of source and receiver distributions is a logistical outcome for any given shot.
Fortunately, modern modeling technology can demonstrate the quantitative relationships between acquisition geometry, the geological model, and the effects upon image quality. A new method presented in Laurain et al (2004) simulates the potential image quality at selected horizons in a 3D geological model for a given survey configuration after migration. The method is based upon 3D ray tracing and incorporates anelastic attenuation and the realistic frequency bandwidth of the seismic signal. In contrast, previous illumination analysis tools failed to incorporate the Fresnel zone, and did not incorporate scattering effects when estimating image quality. Such tools will complement the growth of Multi-Azimuth (a survey acquired in two or more directions by a single vessel) and Wide-Azimuth (a survey acquired with two or more source vessels, in order to significantly increase the crossline aperture for each shot location) surveys (refer to Figure 1 for a schematic comparison).
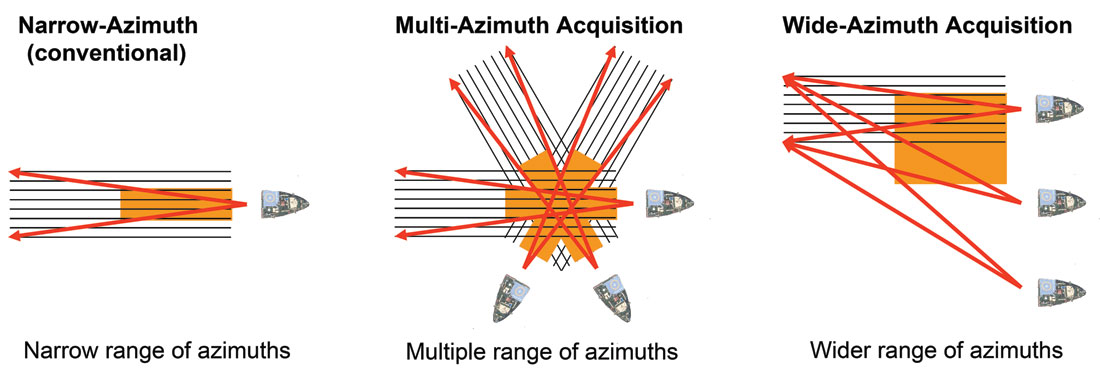
The Varg Field in Block 15/12 of offshore Norway is a field with significant imaging challenges. The 1998-1999 vintage data was acquired with a two-streamer vessel in the East- West (“dip”) direction, and was reprocessed with Kirchhoff PSDM migration two times. Image quality was persistently poor in the reservoir zone. Comprehensive survey analysis demonstrated that different shooting directions would yield complementary but different target illuminations. Hence, a decision was made to acquire a Multi-Azimuth survey with two new directions on top of the existing survey, and then combine all three in one final image (Gaus and Hegna, 2003). Figure 2 demonstrates that the Multi-Azimuth result provided a step-improvement in image clarity and resolution by comparison to the original data. Both datasets were processed through a pre-stack depth migration (PSDM) flow. Two new wells were subsequently drilled, more than doubling the recoverable reserves. Several subsequent Multi-Azimuth surveys in other global locations have proven the technology as a successful tool to overcome imaging and resolution challenges in areas affected by poor target illumination. In the most difficult scenarios, such as subsalt imaging in the GOM, Wide-Azimuth acquisition is also becoming accepted as a successful tool.
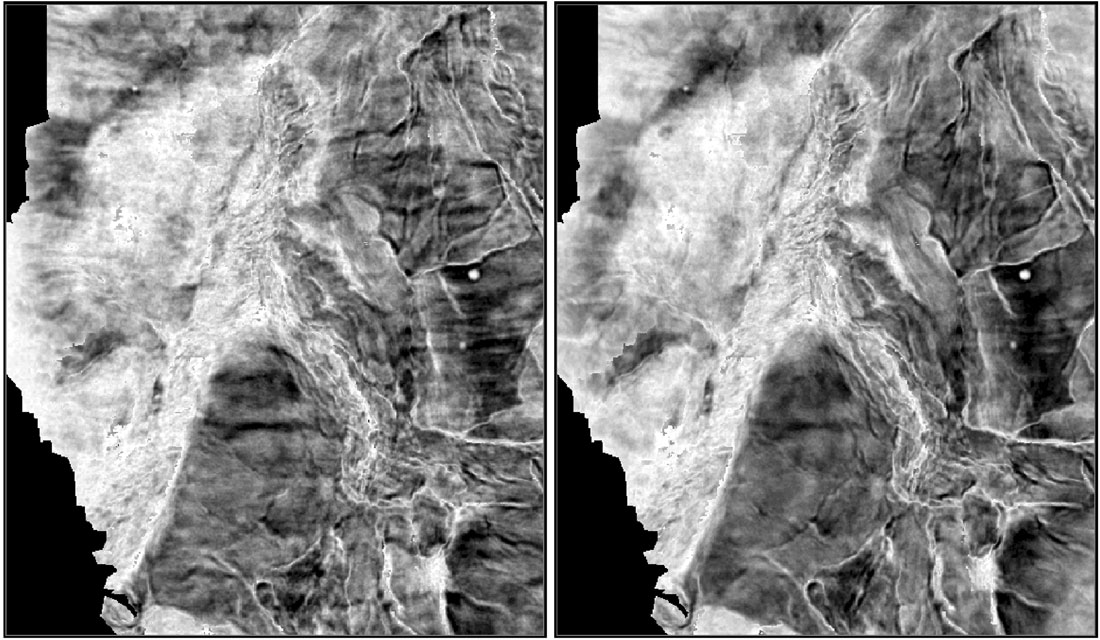
Longer offsets may benefit imaging quality for very deep targets. Many surveys are now acquired with 8 km long streamers, and we may require even longer offsets in areas such as the GOM. Very long offset acquisition presents significant logistical risks and challenges, and will typically be associated with expensive infill requirements. Continuous Long Offset (CLO) is an alternative technology to long streamers that uses an additional shooting vessel placed directly forwards of the streamer vessel, with both vessels towing source arrays, as described in van Mastrigt et al (2002). Consequently, the effective far offsets acquired are twice the streamer length deployed. The shorter streamer deployment allows the efficient towing of very high streamer counts, even at very close separation, with minimal infill requirements, easier line turns, and the benefit of very long offsets.
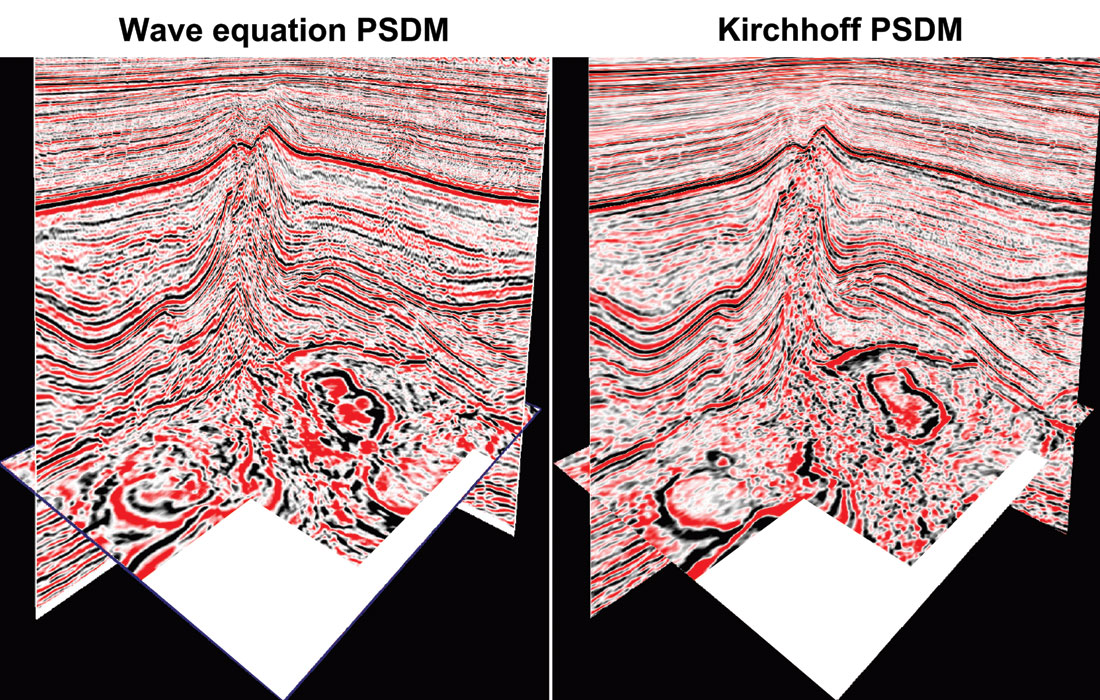
As discussed earlier, complex ray-pathing through complex geology render standard migration algorithms useless in severe scenarios. Kirchhoff PSDM cannot use all arrivals from multipathing, and also suffers from the assumption that local velocity anomalies do not exist (the velocity model is smooth). The introduction of PC cluster technology is now making so-called wave equation PSDM (WEPSDM) affordable (e.g., Ren et al 2004). WEPSDM can handle multi-arrivals and complex velocity regimes. Figure 3 provides a comparison between Kirchhoff PSDM and WEPSDM in the North Sea. Note the better steep dip imaging and the better imaging below the high velocity layer when using WEPSDM. A notable caveat is that WEPSDM requires a very accurate velocity model. Fortunately, 3D velocity model building is rapidly maturing due to advances in the tools used to compute velocity functions and the availability of high performance visualization technology on the desktop of the processing geophysicists.
Seismic improvements for reservoirs masked by complex multiples
Seismic multiples can have a multitude of mechanisms, and are a tremendous problem in many areas. Surface Related Multiple Elimination (SRME) has emerged in recent years as the first realistic processing method to handle multiples in 3D geology. The technique itself is very elegant as it predicts all the multiples reflected from the free surface from the data itself. The surface-related multiples are computed by convolving all possible common shot gathers with all possible common receiver gathers. The 3D implementation of SRME assumes that sources and receivers are available in all possible surface bin locations. This is impossible in practice. Hence, the challenge to 3D SRME lies in the interpolation or inversion of missing data (e.g., van Borselen et al., 2005). 3D SRME has been applied to most streamer geometries, primarily due to significant improvements in “data regularization” technology, but it is proven that denser sail line spacing combined with denser streamer configurations yield optimal results.
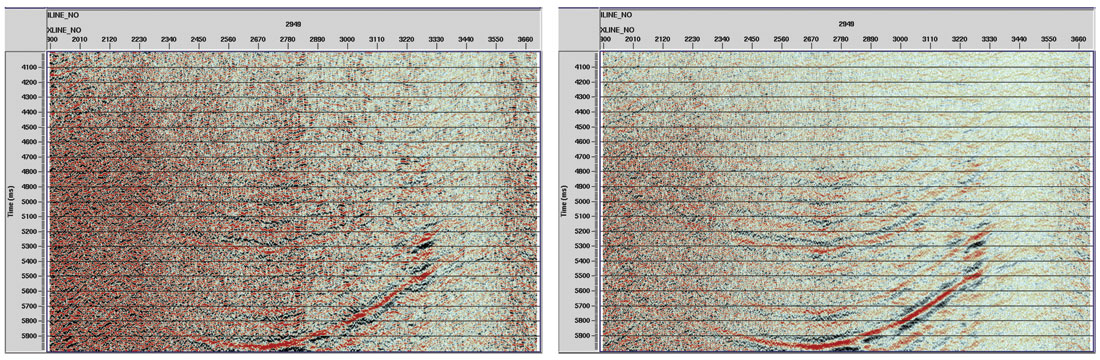
3D SRME is commonly touted as a potential solution to “multiple diffractions”; the most challenging multiple scenario, where the apex of diffractions associated with surface or near-surface heterogeneities is out-of-the-plane, and multiples of such diffractions obscure the target geology. As an alternative acquisition-based solution, a customized variant of Wide-Azimuth has been proven to suppress multiple diffractions. The configuration used was a dual-source eight-streamer vessel and an additional source vessel. Nine different sail line passes were made over the same location, each pass with a different lateral separation between the two vessels, and the data were binned into eight different azimuths. Figure 4 shows a comparison of a single azimuth and all azimuths combined. The figure clearly illustrates the improvements in image quality obtained by using stacking to exploit the differential moveout of diffraction multiple events after the primary events are flattened.
Technological solutions for noise reduction in acquisition and processing
Swell noise is problematic in marginal weather conditions because “bulge waves” propagated along the skin of fluid-filled streamers create unwanted low frequency noise bursts. One attempt to overcome this problem has been implemented by WesternGeco, who apply mixing and dip filtering routines in processing to closely-spaced single hydrophone recordings. The parameterization of the filtering weights will be statistically based upon overall survey conditions, and may thus vary throughout the survey area. Another approach to this problem is the introduction of solid streamers. Solid streamers have become increasingly popular due to the improved performance in marginal weather and the absence of potential fluid leakage into the environment when damaged. A new buoyant void filler (BFV) technology has been developed by PGS with several advantages over other solid streamer technologies (Figure 5). The BVF is a hydrocarbon-based material that cures into a solid with specifically engineered properties. In contrast to a fluid, the BVF cannot flow, and therefore cannot support waveforms such as bulge waves. Furthermore, unlike most solid materials, the BVF cannot transmit energetic shear waves very well, and therefore provides a low noise platform for the hydrophones. Figure 6 compares noise levels between fluid filled and BVF filled streamers over the frequency spectrum acquired by a hydrophone group. Note the significant reduction in noise level where the liquid filled streamer has a noise burst. For more details about this technology, refer to Hoogeveen et al. (2005).
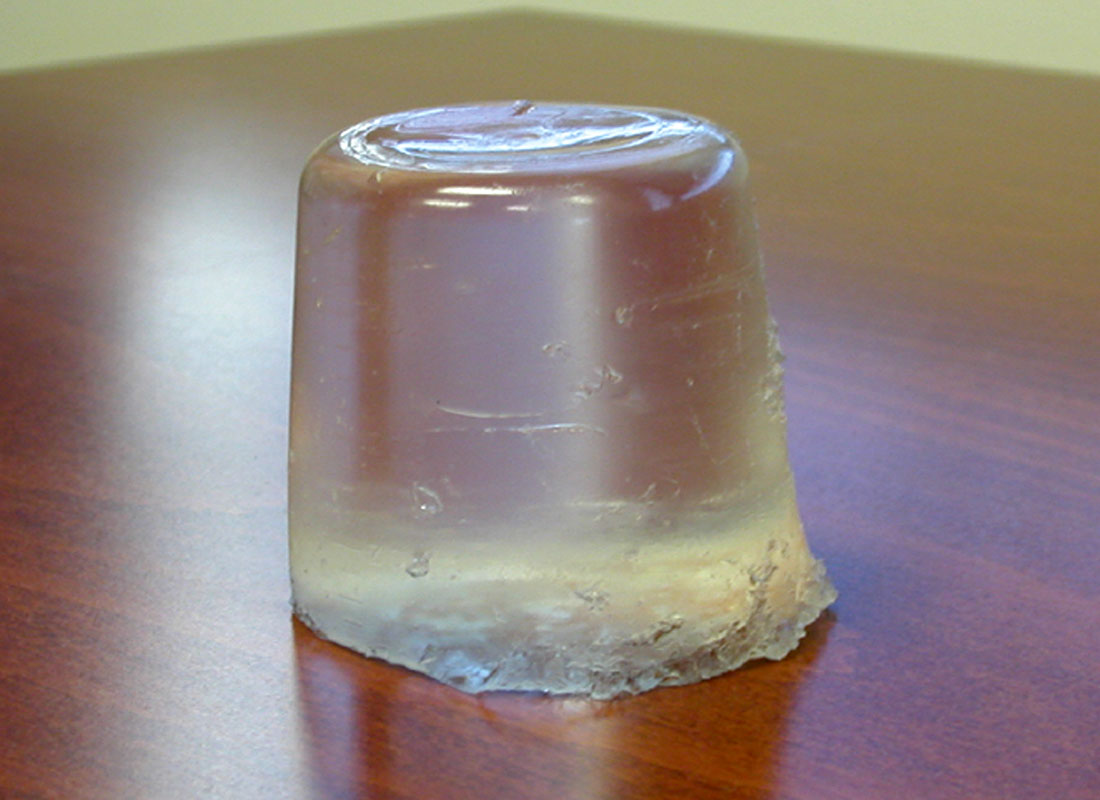
Another type of problematic seismic noise arises from production- related activity near the seismic survey location. Examples include vessel interference, fixed installations and drilling. “True 3D” processing solutions developed recently remove such noise in an elegant manner by modeling, aligning, and then removing each 3D noise source in an iterative manner from all traces in each gather. For more information, refer to Fookes et al. (2003).
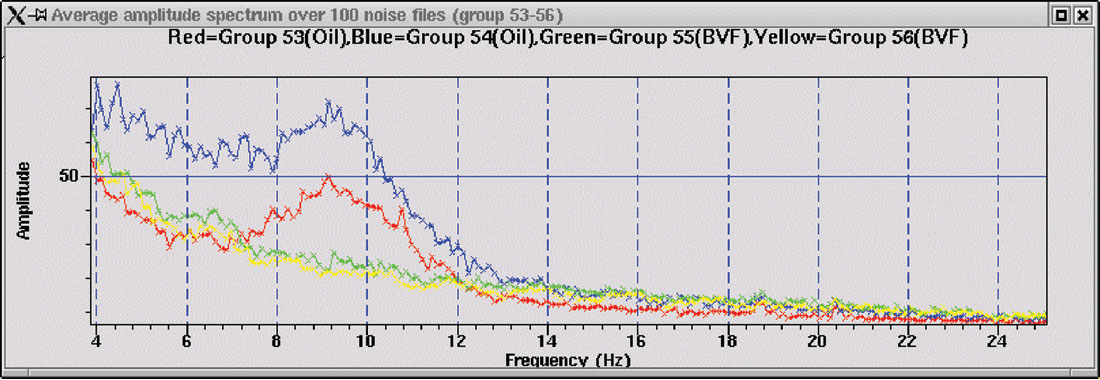
Note that as most multiple and noise types are generated in the near-surface, their apparent dip on seismic gathers will be large, and such events will be particularly prone to aliasing. Dense 3D spatial sampling will be critical to the success of any effort in processing to attenuate noise and multiples whilst simultaneously preserving the high frequency content of the underlying primaries – even when the primary targets are very deep. This fact is often overlooked. Fortunately, modern vessel technology allows the efficient towing of high-density streamer spreads with very high streamer counts.
Advances in 4D seismic for the monitoring of small changes in reservoir state during production
Time-lapse (4D) streamer seismic monitoring of reservoir production has matured significantly in recent years. 4D monitoring by towed streamer surveys is now applied routinely on several fields in the North Sea and is on the verge of mainstream application in most other areas around the world. Permanent seabed installations for “life of field seismic” (LoFS) monitoring (e.g. the Valhall field; Barkved, 2004) offer step improvements in the sensitivity of monitoring, but at step increase in cost. Nevertheless, many oil companies are now recognizing the strategic merits of LoFS for certain fields. The decision to pursue streamer 4D or installed LoFS depends upon factors such as the cost of deployment versus the return on recovery, the strength of the 4D signal, and how often the survey will be repeated. Seasonal effects such as weather must be considered, as must be the likely availability of appropriate streamer vessels within desired time slots. The acclaimed success of the Valhall LoFS, coupled with better and cheaper installation technology may lead to more installations in the future. One potentially applicable example is optic sensor technology, which promises lower cost, lightweight instruments capable of operating for two decades in very deep water and with very lower power requirements. For more details, refer to Maas et al. (2004). Streamer seismic now dominates the 4D market, and has matured in quality rapidly within recent years.
As every source and receiver position in a baseline survey can never be precisely repeated in a monitor (repeat) survey, the source-receiver ray path geometries through the Earth will differ, and acquisition-related effects may obscure the 4D signal. This issue of “non-repeatability” is most relevant for near-surface scatterers (Calvert, 2005), which are naturally prone to aliasing. Widmaier et al. (2005) describe a proven strategy (Figure 7) to optimize seismic repeatability that has become the common foundation of 4D streamer seismic tenders:
- Repeated sail line direction,
- Repetition of shot lines and shot positions,
- Streamer overlap applied to maximize source-receiver azimuth repeatability at sail line seams,
- Dense streamer separation to maximize source-receiver repeatability and reduce cross-line receiver aliasing, and
- Customized 4D binning and regularization to minimize residual small errors in source and receiver positions.
Dynamic trimming of streamer feathering using steerable birds is an alternative technology used to improve the repeatability of source- receiver azimuths. This technique may reduce the necessity of overlapping streamers, but will introduce turbulence noise when the streamers are forced against the natural feathering path. Correction for errors in the position of the source array between repeat surveys has never received much attention to date. The exception is the SOS (self-overlapping-system) approach of Naess (2005) that uses the availability of excess sub-arrays to switch the choice of active sub-arrays contributing to the total array when the source array centre position exceeds some lateral threshold.
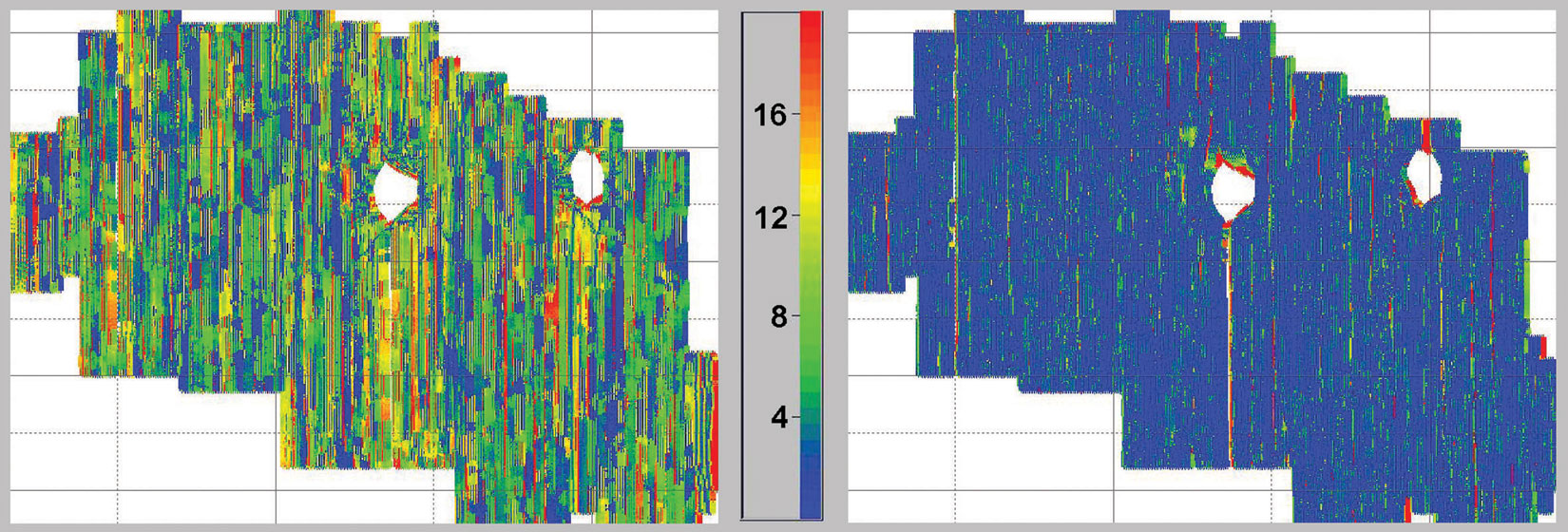
Overall, it is critical that 4D acquisition is robust and transparent. Eiken et al. (2003) demonstrate that dense streamer towing complemented by accurate data regularization can reduce the NRMS repeatability error to about 6%, which corresponds to the minimum theoretical 4D threshold in ideal conditions as described by Calvert (2005).
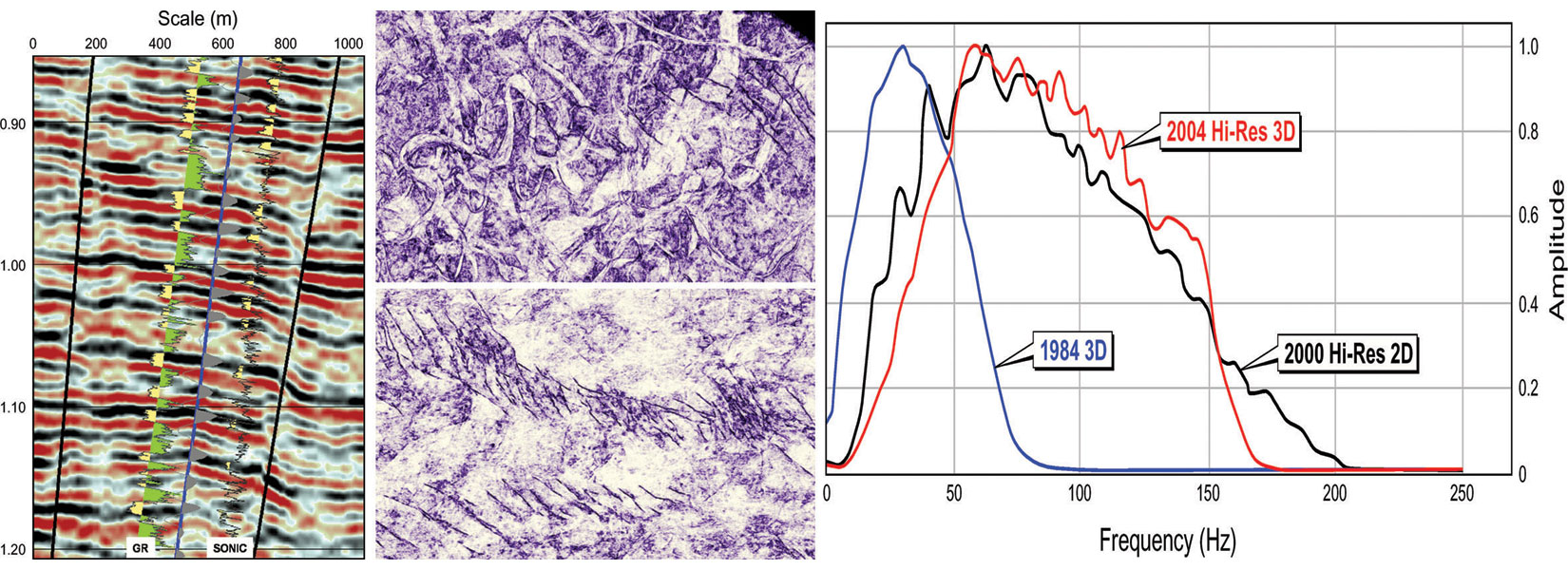
4D streamer seismic will never be perfect, and significant differences between repeat survey geometries are still common. The QC and processing of 4D surveys is thus critical to the ultimate success of any project. Multi-attribute volumetric data QC with visualization tools has rapidly matured for applications to both the acquisition and processing of 4D data (Brittan et al., 2002).
Better resolution
High resolution of seismic data results when high frequency geological features in close proximity can be precisely focused without any contamination from artifacts or noise (i.e. high signal-to-noise ratio). In general, recoverable frequency bandwidth is an important factor for vertical resolution and dense 3D spatial sampling is an important factor for spatial resolution. Vertical and horizontal resolution are linked by the success of high frequency focusing and imaging.
High resolution of 3D seismic data is achieved by the application of rigorous geophysical principles. In particular, the systematic pursuit of: 1. Uniform target illumination, 2. Dense spatial sampling of the reflected wavefield, and 3. Careful processing and imaging, will collectively deliver an optimum result (Ramsden et al., 2005). Note that the final step can never be successfully completed without the platform of proper target illumination and wavefield sampling. The use of Multi-Azimuth and Wide-Azimuth acquisition to improve target illumination was discussed earlier.
Modern vessels such as the Ramform technology allow up to 16 streamers to be towed at separation as small as 25 m, provided that streamer lengths are not larger than about 4000 m. Improved streamer construction, such as the BVF solid streamer technology discussed earlier, are contributing to reduced streamer turbulence noise in the data. Beyond the fundamental issues of uniform target illumination and dense wavefield sampling, secondary contributions to seismic artifacts are variability in source signatures and navigation mis-positioning for source and receiver positions. Most modern vessels have source monitoring capabilities that enable shot-by-shot signature deconvolution. Dense acoustic networks are fixed to both the source sub-arrays and streamers, reducing positioning errors to less than a few meters. High-order data regularization technology can also compensate for both coarse and minor errors in source and receiver positions, yielding high fidelity seismic images with large frequency bandwidth for both low and high-dip events (Schonewille, 2003).
As the quality of acquisition geometry improves the applicability of “true 3D” algorithms such as out-of-the-plane noise removal, 3D SRME and pre-stack migration (notably WEPSDM variants) correspondingly increases. The continuous improvement in seismic resolution thus follows from a symbiotic link between better acquisition and better processing.
Conclusions: The next five years
Complementary non-streamer exploration tools such as electromagnetic (EM) measurements for the monitoring of hydrocarbon movements in resistive reservoir rocks was not discussed here, but their popularity will clearly increase. The use of autonomous seafloor nodes as a sparsely sampled, low-cost alternative to seafloor cables will apparently increase within the next few years, but this technology appears to be either a temporary solution to “proper acquisition”, or another complementary (but secondary) tool for reservoir monitoring.
In the first order, more oil companies are increasingly acknowledging that more expensive and customized streamer seismic solutions are necessary to overcome long-standing challenges to seismic data quality and value. The obvious technology benefit to such pursuits arises from continuous improvements in the ability of vessels to tow denser streamer spreads, longer streamers, and with greater operational flexibility in a larger range of survey environments. True 3D processing and imaging technologies will correspondingly improve with the availability of better data platforms.
In the next five years the average trace density of all surveys will increase – for both exploration and monitoring applications. As the shift in industry attention increasingly moves to deeper water and deeper target pursuits, attention will obviously focus upon means to efficiently acquire very long offsets, how to acquire better low frequency content, and how to create better seismic images in areas prone to poor target illumination and complex wave propagation effects. Multi-Azimuth and Wide-Azimuth clearly will thus have prominence. 4D seismic will probably become routine for more than half of new reservoir discoveries, and will thus influence the parameterization of exploration surveys (which may later become baseline surveys). Several instrumented (LoFS) oilfields will probably be installed each year, particularly if optical sensor technology reaches full commercialization.
In the second order, continuous improvements in the application of QI pursuits, better visualization technology, and an increasing focus upon reservoir management from earlier stages in the overall exploration-to-exploitation sequence will collectively shorten the turnaround from acquisition to simulation. All of these ideals are of course meaningless without the provision of better seismic data with higher resolution, more repeatability and greater clarity.
The only guarantees when attempting to predict the future is that some predictions will look foolish, but an attention to best practice geophysics can have no downside.
Join the Conversation
Interested in starting, or contributing to a conversation about an article or issue of the RECORDER? Join our CSEG LinkedIn Group.
Share This Article