Abstract
The subsurface zone in which the pressure and temperature conditions are conducive to the occurrence of gas-hydrates is areally extensive beneath most of the Mackenzie Delta-Beaufort Sea region, with the base of the gas-hydrate stability zone more than 1,200 m deep on Richards Island. In a recent study, gas hydrates have been inferred to occur in nine Richards Island exploratory wells on the basis of well-log responses calibrated to the response of the logs within the cored gas-hydrate-bearing intervals of the JAPEX/JNOC/GSC Mallik 2L-38 well. The integration of the available well log data with more than 240 km of industry acquired reflection seismic data has allowed the mapping of four significant gas hydrate and associated free-gas accumulations in the Ivik-Mallik-Taglu area on Richards Island. The occurrence of gas hydrates on Richards Island is mostly restricted to the crest of large anticlinal features that cut across the base of the gas-hydrate stability zone. Combined seismic and well log data analysis indicate that the known and inferred gas hydrate accumulations on Richards Island may contain as much as 187,178x106 cubic meters of gas.
Introduction
Even though gas hydrates are known to occur in numerous arctic sedimentary basins (Collett and Dallimore, 2000), little is known about the geologic parameters controlling their formation and occurrence. The primary objective of this report is to review the results of a recently completed study in which available geologic and geophysical data were used to assess the distribution and size of the gas hydrate and associated free-gas accumulations in the area of the Ivik, Mallik, and Taglu conventional gas fields on Richards Island in the outer Mackenzie Delta (Figure 1) (Dallimore et al., 1999). This study identified four distinct gas hydrate accumulations on Richards Island. In addition, the geologic controls on the occurrence of these four inferred gas hydrate accumulations was evaluated. This resulted in the development of a hydrate “play” model that can be used to explain the occurrence of the Ivik- Mallik-Taglu gas hydrate accumulations and predict where similar occurrences might be found in unexplored regions.
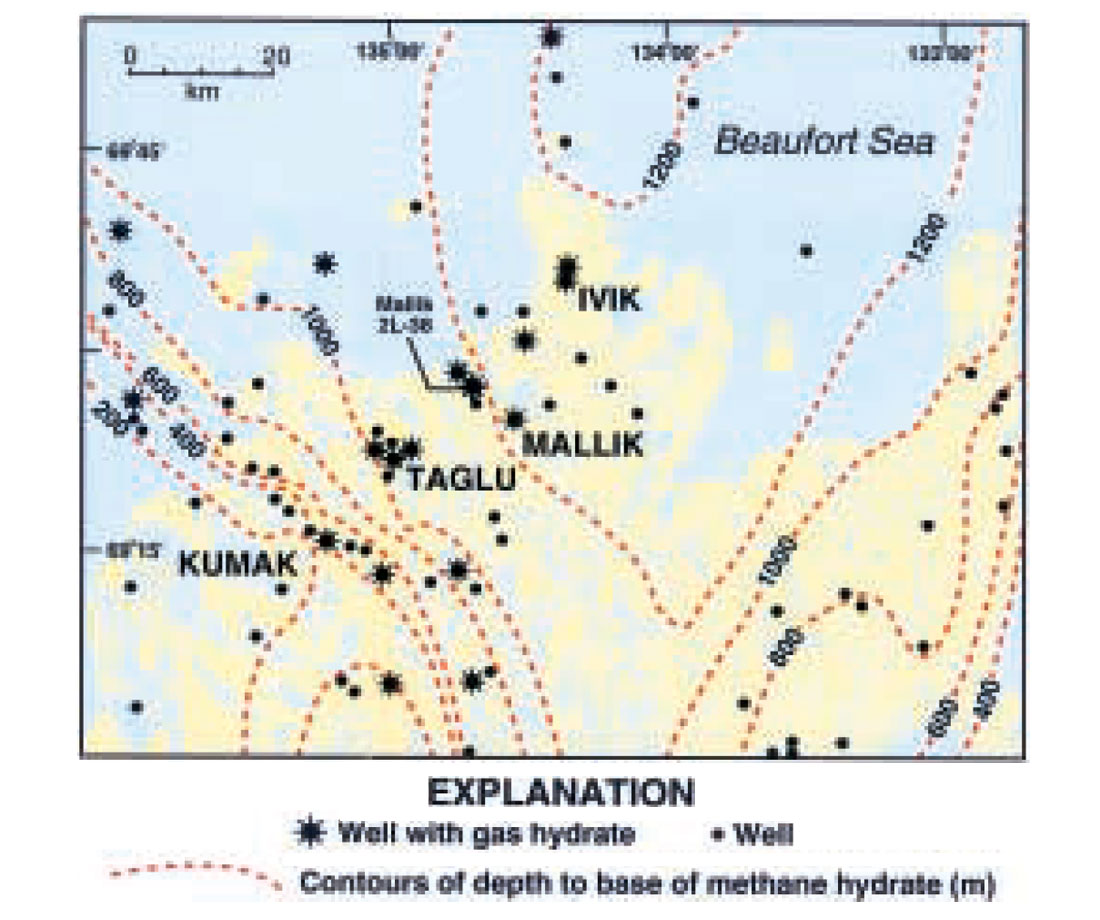
This report begins with a detailed review of the methodology used to process and interpret the available industry open-hole well-log and reflection seismic data from Richards Island, which is followed by a discussion of the results of the log and seismic data interpretation. This report concludes with a description of four inferred gas hydrate accumulations, including an estimate of the volume of gas within the delineated gas hydrate accumulations.
Methodology
Open-hole conventional well log data from 11 industry exploratory wells (including the JAPEX/JNOC/GSC Mallik 2L-38 gas hydrate research well; Dallimore et al., 1999) located on Richards Island and the eastern part of the modern Mackenzie Delta were obtained to assess the occurrence of gas hydrates and associated free-gas accumulations in the Ivik-Mallik-Taglu area (Figure 2). With the exception of the Mallik 2L-38 well, all of the wells examined in this study were drilled in the early 1970’s as industry exploration wells designed to test the more deeply buried conventional hydrocarbon accumulations in the region.
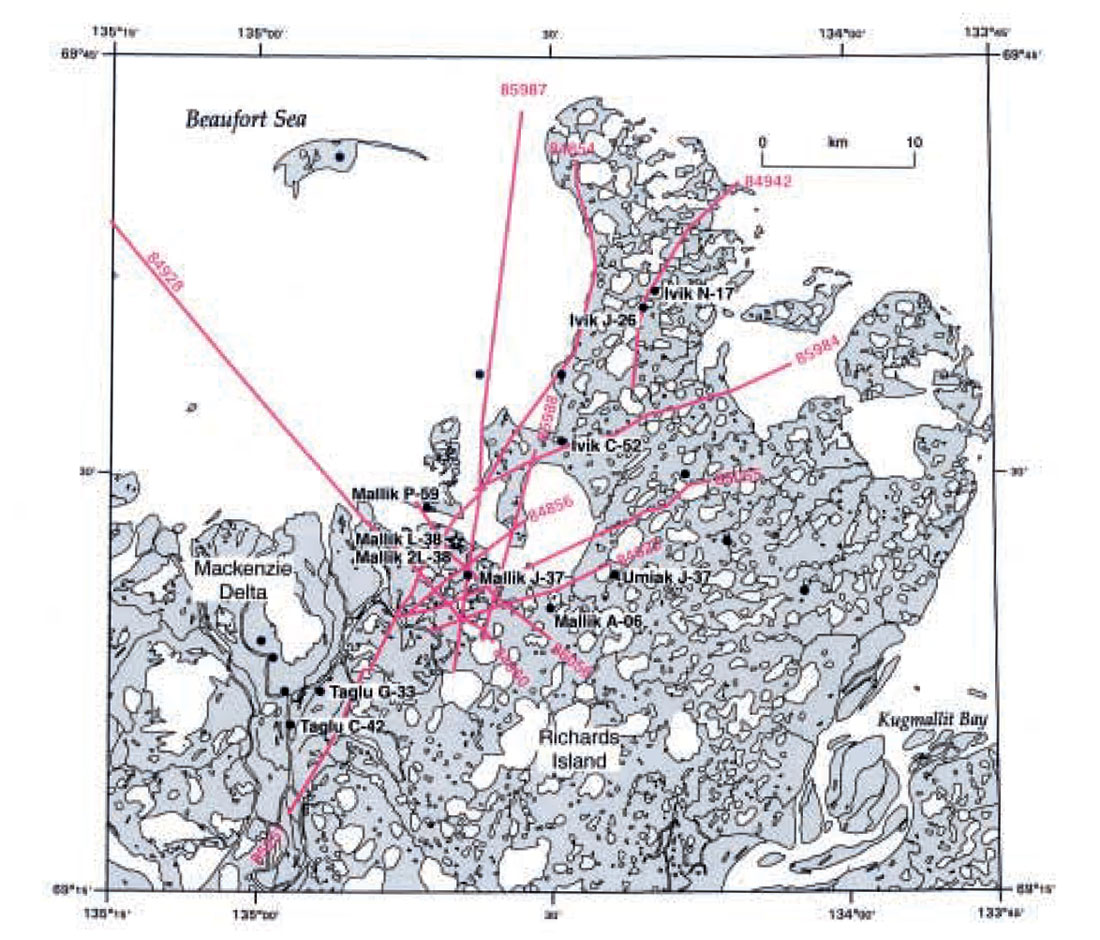
Digital files for thirteen reflection seismic profiles, totaling about 240 km of data, were obtained from Imperial Oil Limited (IOL) for a portion of Richards Island within the outer Mackenzie Delta (Figure 2). These seismic data were acquired for conventional oil and gas exploration purposes in 1984 and 1985, so the field parameters were not optimum for the evaluation of shallow gas hydrate related features. However, specialized reprocessing provided the information needed to understand the distribution of gas hydrate and associated free-gas accumulations.
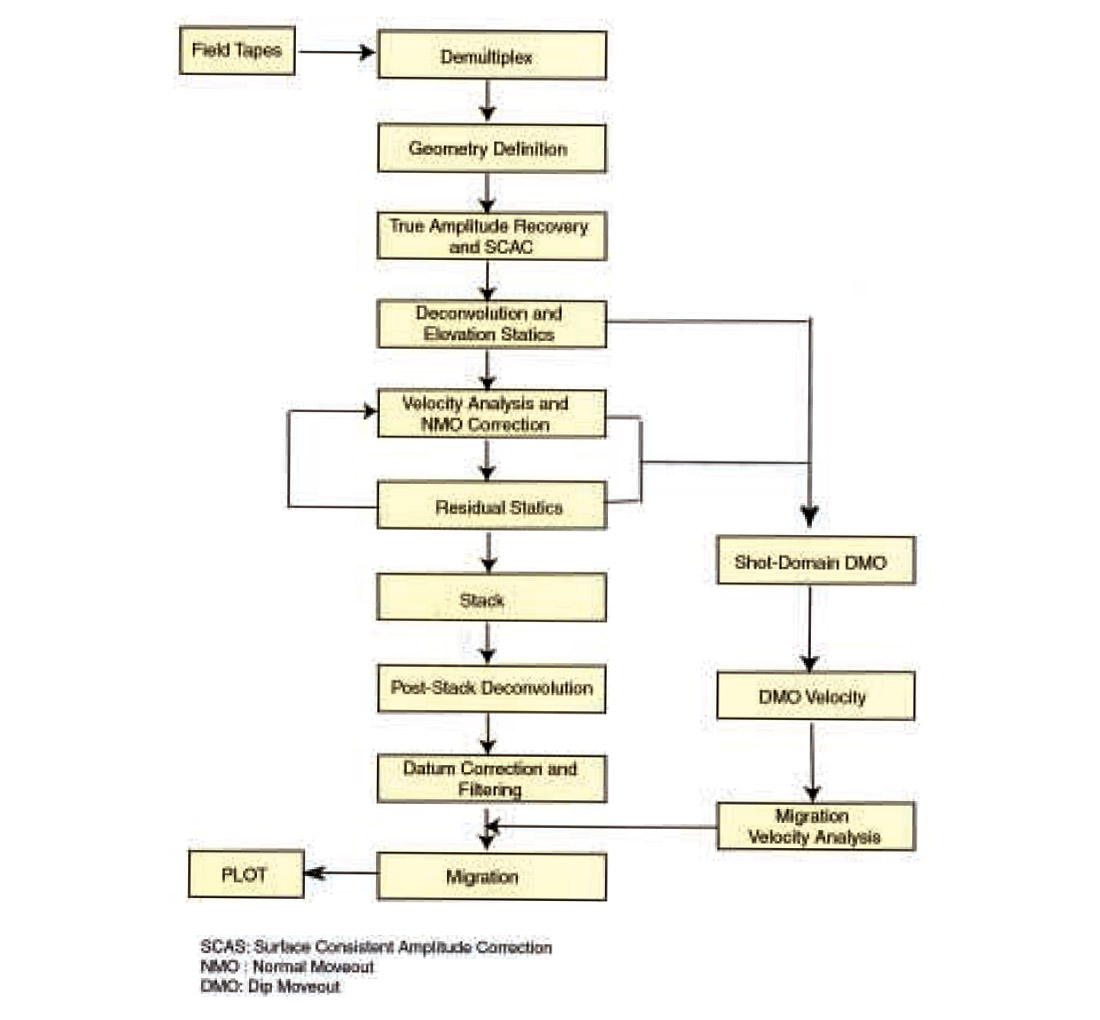
The methods and processing steps used to reprocess the IOL seismic data are displayed in the processing flowchart in Figure 3. In order to use reflection amplitudes to map the distribution of gas hydrate and free-gas, a processing strategy capable of preserving the relative true amplitudes (RTA) was applied. The important steps in RTA processing are (1) editing noisy traces, (2) applying programmed gain functions, and (3) compensating for differences in the source strength, receiver coupling, and other surface related amplitude variations. A programmed gain function, T2, was applied to the data and a spectral balancing was applied as a deconvolution procedure. In order to compensate for the amplitude variation related to the surface conditions, a process called surface consistent amplitude correction (SCAC) was applied. This process compensates for variations in amplitudes owing to the strength of the shot, the response and coupling of the receivers, and the performance of amplifier channels, all of which are not related to reflection amplitudes in a surface consistent manner. This process estimates the trace amplitude based on source, offset, CMP and channel; it contributes and decomposes each contribution and adjusts the amplitude variation. Post-stack wavelet deconvolution and zero-crossing predictive deconvolution were applied to all seismic data in order to increase temporal resolution and to remove the differences of the source wavelets. For a more complete review of the seismic data processing techniques used in this study, including pre-stack depth migration and migration velocity analysis, see the complete seismic data processing report by Lee and Agena (1998).
Geologic setting
The geology of the Mackenzie Delta region has been described in numerous publications (reviewed by Dixon et al., 1992, 1994). Surficial sediments of the Mackenzie Delta are composed in part of modern deltaic sediments and of older fluvial and glacial deposits of Richards Island and the Tuktoyaktuk Peninsula (Figure 2). At depth, this region is underlain by deltaic sandstones and shales of Mesozoic and Cenozoic age that thicken to more than 12 km over a short distance seaward from the present shoreline. This sedimentary section overlies faulted Paleozoic rocks stepping down steeply beneath the Mesozoic and Cenozoic section.
The post Paleozoic sedimentary rocks of the Beaufort Sea continental shelf are subdivided into two major sections: pre-Upper Cretaceous and Upper Cretaceous to Holocene strata. A major regional unconformity marks the boundary between the Upper Cretaceous and older strata (Dixon et al., 1992). Above this regional unconformity, sedimentation was dominated by deltaic processes, resulting in a series of thick, generally northward prograding delta-complexes, to which lithostratigraphic terminology can be applied (reviewed by Dixon et al., 1992). Nine sequences have been identified in the study area using reflection seismic records, well data, and outcrop data (Figure 4). Within the Ivik-Mallik-Taglu area of Richards Island the zone of predicted methane hydrate stability (discussed later in this report) is spatially limited to portions of only four of the delineated sequences: Iperk Sequence, Mackenzie Bay Sequence, Kugmallit Sequence, and Richards Sequence.
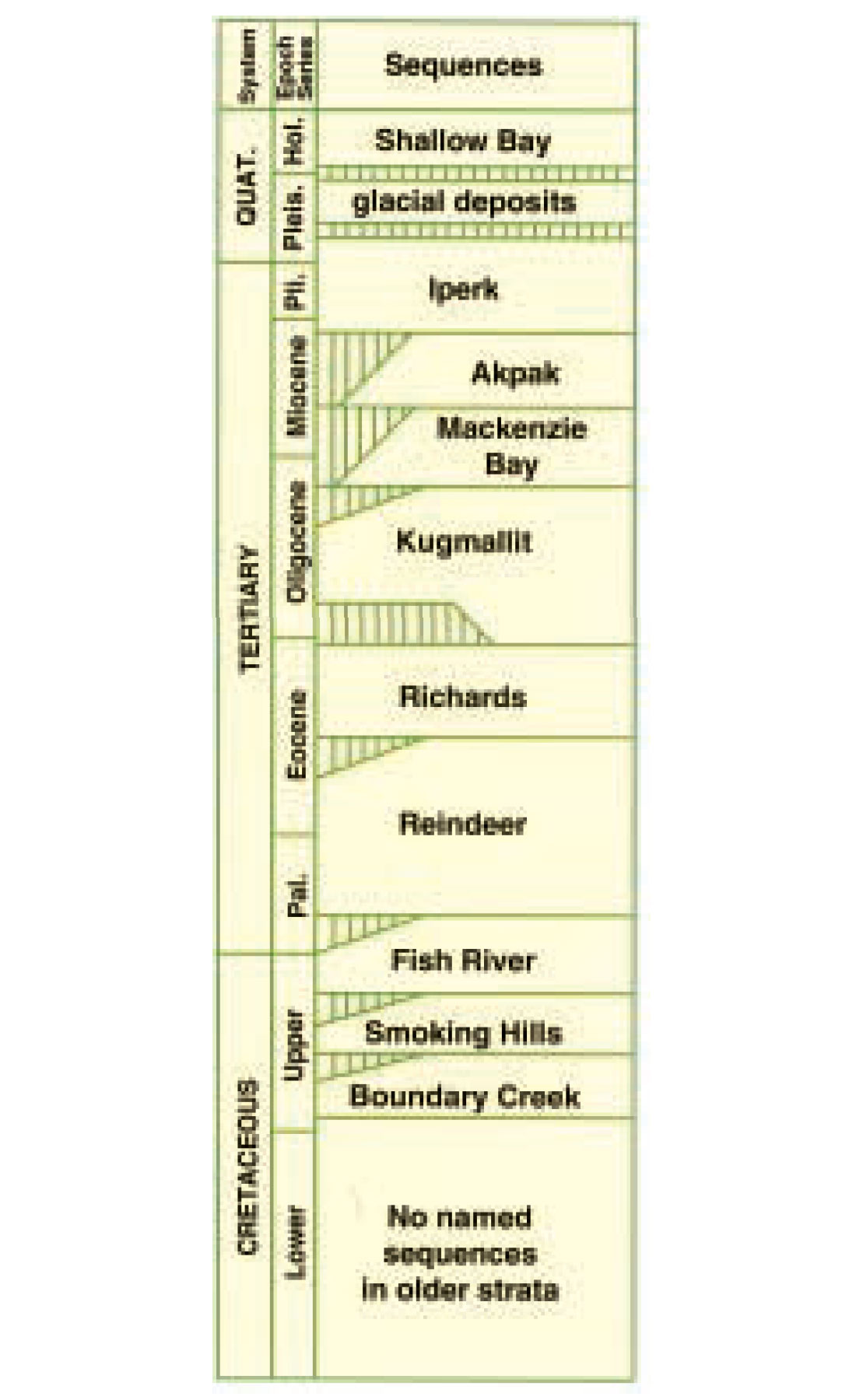
The Tertiary sedimentary rocks of the Mackenzie Delta region are deformed by large-scale growth faults, particularly along the basin margin. A distinct tectono-stratigraphic break occurs between the Late Oligocene to Middle Miocene Mackenzie Bay Sequence and the upper Miocene to Pleistocene Iperk Sequence. Most of the early to mid- Tertiary structural features are truncated at the unconformity surface marking the base of the Iperk Sequence. This late Miocene unconformity marks the end of significant tectonism within the Mackenzie Delta region.
Since the beginning of significant oil exploration in 1962, over 240 petroleum exploration wells have been drilled in the Mackenzie Delta-Beaufort Sea region. More than 40 hydrocarbon accumulations have been discovered, containing an estimated 238 to 317 million cubic meters (1.5 - 2.0 billion barrels) of recoverable oil and 292 to 356 billion cubic meters (10.4 - 12.6 trillion cubic feet) of recoverable gas (Dixon et al., 1994). At least 26 of these oil and gas fields have been discovered in the Tertiary rock sequences of the Mackenzie Delta-Beaufort Sea region (Procter et al., 1984).
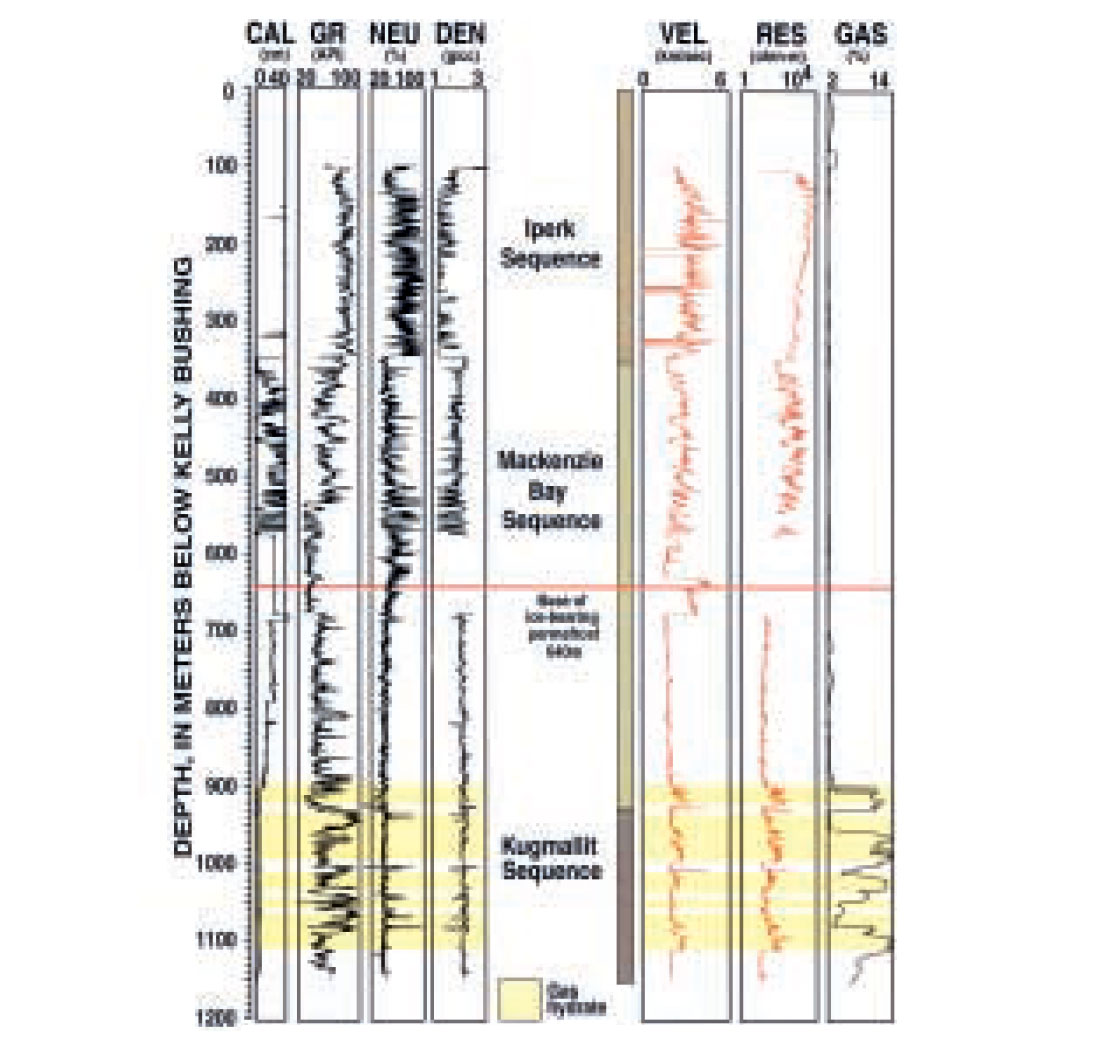
The occurrence of gas hydrates is limited to a certain range of temperature and pressure conditions (reviewed by Sloan, 1990); therefore, the physical limits of the zone in which conditions are suitable for gas hydrate formation can be determined. Maps of the methane-hydrate stability zone in the Mackenzie Delta-Beaufort Sea sedimentary basin (Figure 1) show that the zone in which methane hydrates can occur extends to depths greater than 1,200m on Richards Island and is extensive beneath most of the continental shelf area (Judge and Majorowicz, 1992). Natural gas hydrates are thought to occur in 25 Mackenzie Delta-Beaufort Sea exploratory wells (reviewed by Smith and Judge, 1993). All of these inferred gas hydrates occur in clastic sedimentary rocks of the Tertiary Kugmallit, Mackenzie Bay, and Iperk sequences (Procter et al., 1984; Dixon et al., 1992, 1994; Smith and Judge, 1993). Gas hydrates were also recovered in a core at a depth of 336.4 m in the 92GSCTAGLU core hole (Dallimore and Collett, 1995).
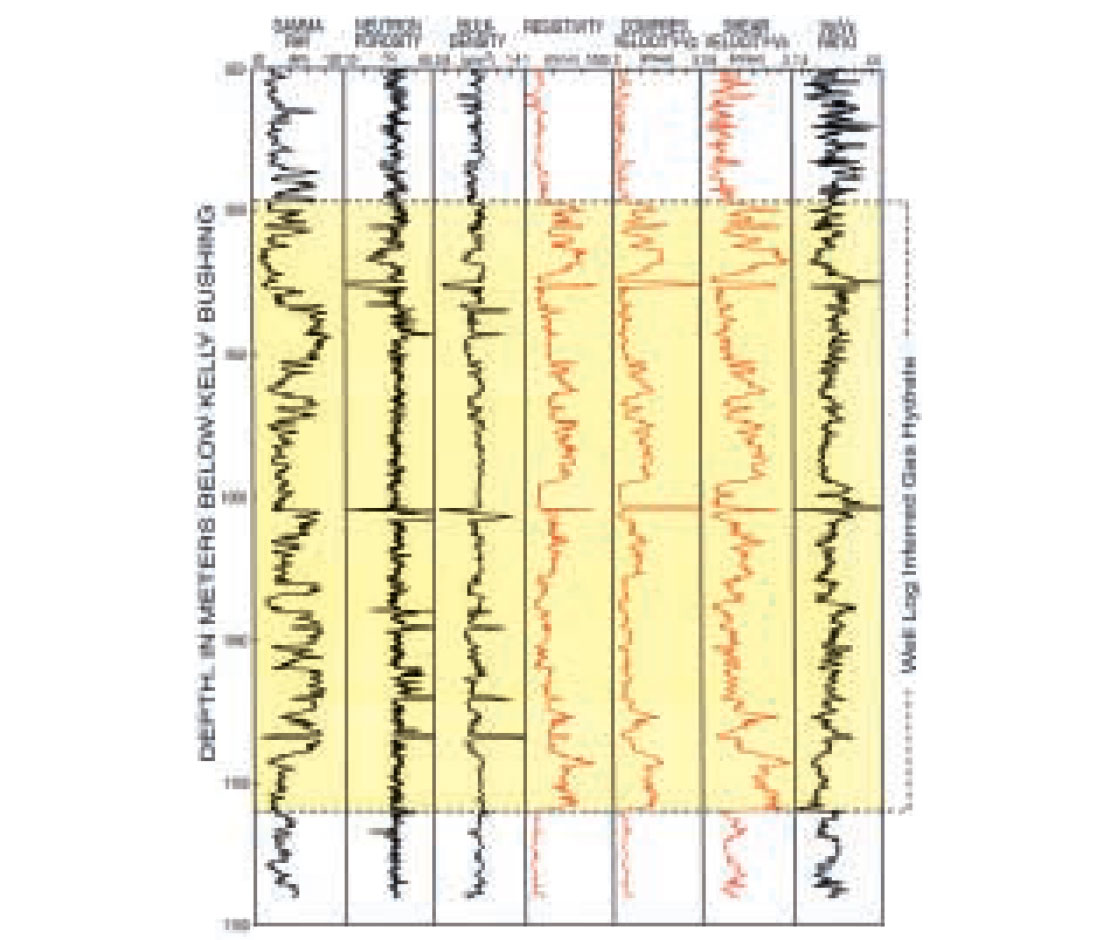
Prior to the completion of the JAPEX/JNOC/GSC Mallik 2L-38 gas hydrate research well in 1998 (Figures 5 and 6), the most extensively studied gas hydrate occurrences on Richards Island were those drilled in the Mallik L-38 and Ivik J-26 wells (Bily and Dick, 1974). It is estimated that the Mallik L-38 and Mallik 2L-38 wells encountered more than 100 m of gashydrate-bearing sediments (Dallimore et al., 1999), and the Ivik J-26 penetrated about 25 m of gas hydrate (Bily and Dick, 1974). The gas hydrate occurrences in the Ivik J-26 well are very similar to those in the Mallik L-38 and also occur within the predicted methane-hydrate stability zone and below the base of ice-bearing permafrost. All of the well-log inferred gas hydrates in the Mallik and Ivik area occur in clastic sedimentary rocks of the Kugmallit and Mackenzie Bay sequences.
Well log evaluation of gas hydrates
In most gas hydrate studies only two well logging devices are consistently used to identify potential gas hydrates: they are the electrical resistivity and acoustic transit-time logs. In comparison to water saturated sediments, gas-hydrate-bearing sediments exhibit anomalously high electrical resistivities and rapid acoustic transit-times (high acoustic velocities).
The velocity of a compressional acoustic wave in a solid medium, such as gas-hydratebearing sediment, is usually several times greater than the velocity of a compressional acoustic wave in a gas-bearing sediment. In most well-log studies, gas-bearing zones highly attenuate compressional acoustic waves and appear as anomalous low velocity zones. Therefore, at the base of the gas-hydrate stability zone, which marks the contact between gashydrate- and free-gas-bearing sediments, the acoustic log is often characterized by a distinct drop in acoustic velocity from the gas-hydrate-bearing section above into the underlying freegas-bearing interval.
The subsurface depths used for each well in this report are given in depth below ground surface, which was calculated by subtracting the height above ground surface of the Kelly Bushing on the drilling rig from the downhole measured log depths.
Bily and Dick (1974) determined that the Ivik J-26 well penetrated about 25 m of gashydrate- bearing sediments within the depth interval from 973.9 to 1,014.1 m. The well-log inferred gas-hydrate-bearing sediments in Ivik J-26 exhibit very high acoustic velocities and electrical resistivities on the recorded downhole logs. The analyses of available acoustic transit-time and electrical resistivity logs also revealed a relatively thick gas hydrate occurrence in the Ivik N-17 well within the depth interval from 960.6 to 1057.8 m, which is near the same depth of the gas hydrate occurrence in the Ivik J-26 well. Analyses of downhole well logs from the Ivik J-26 and N-17 wells revealed no evidence of free-gas below the log inferred gas hydrate occurrences in the Ivik area.
Based on the analyses of the downhole recorded electrical resistivities and acoustic velocities, it appears that the Ivik C-52 well also penetrated a relatively thick gas-hydrate-bearing sedimentary section within the depth interval from 793.8 to 923.8 m.
The Mallik L-38 well was drilled by Imperial Oil Limited in 1972 to a depth of 2,524 m. The base of ice-bearing permafrost in the Mallik L-38 well is estimated at 613 m and the base of the methane hydrate stability zone, predicted from borehole temperature surveys, is at a depth of 1,100 m. As previously discussed, the Mallik L-38 well is believed to have penetrated about 110 m of gashydrate- bearing sandstone units within the depth interval from 810.1 to 1,102.3 m (Dallimore et al., 1999).
Bily and Dick (1974) originally interpreted the presence of free gas in contact with gas hydrate in several intervals of the Mallik L- 38 well. However, the analyses of log data from the Mallik 2L-38 well, confirmed the occurrence of only one relatively thin free-gas zone (1,100.5-1,101.9 m) at the base of the deepest downhole log inferred gas-hydrate at the Mallik drill-site (Collett et al., 1999b).
The Mallik 2L-38 gas hydrate research well was drilled by JAPEX/JNOC/GSC in early 1998 to a depth of 1,150 m (Dallimore et al., 1999). Similar to the Mallik L-38 well, the base of ice-bearing permafrost in Mallik 2L-38 is estimated at 613 m and the base of the methane hydrate stability zone, predicted from borehole temperature surveys, is at a depth of 1,100 m. Downhole electrical resistivity and acoustic transit-time (both compressional- and shear-wave) logs from the Mallik 2L-38 well confirm the occurrence of in-situ gas hydrates at the Mallik drill-site within the subsurface depth interval between 888.84 and 1,101.9 m (Figures 5 and 6). The cored and logged gas hydrate occurrences in the Mallik 2L-38 well exhibit deep electrical resistivity measurements ranging from 10 to 100 ohm-m and compressional-wave acoustic velocities (Vp) ranging from 2.5 to 3.6 km/sec. In addition, the measured shear-wave acoustic velocities (Vs) of the confirmed gas-hydrate-bearing horizons in the Mallik 2L-38 well range from 1.1 to 2.0 km/sec.
Based on the analyses of the downhole log data, it appears that the Mallik P-59 well penetrated a relatively thick gas-hydrate-bearing sedimentary section within the depth interval from about 840.1 m to 996.9 m, and a distinct drop in acoustic velocity at a depth of 996.9 m marks the contact between gas-hydrate- and freegas-bearing sediments. Similar to the downhole log responses in the Mallik P-59 well, it appears that the Mallik J-37 well may have penetrated a relatively thick gas-hydrate-bearing sedimentary section within the depth interval from about 800 to 1,100 m, and the well inferred gas hydrate may be underlain by free-gas; however the well log evidence is inconclusive. Our well log analysis studies further suggest that the Mallik A-06 well may have penetrated two distinct gas-hydrate-bearing sandstone units within the depth interval from 965.4 to 1,148.2 m.
Downhole log data reveal that the Taglu G-33 well penetrated three thin (thicknesses ranging from 2 to 5 meters) gas-hydrate-bearing sedimentary units within the depth interval from 925.7 to 944.7 m. Log data from the Taglu C-42 well also infer the occurrence of about 12 gas-hydrate-bearing stratigraphic units within the subsurface depth interval between 770.1 and 1,085 m, with thicknesses of the individual gas-hydrate-bearing units ranging from 2 to 25 meters.
In this study, we have used the available downhole log data from the Ivik-Mallik-Taglu wells to calculate sediment porosities and gas-hydrate saturations within the log inferred gas-hydrate-bearing stratigraphic units. When available, the unedited bulk-density (ρb) log measurements were used to calculate sediment porosities (∅) in the wells examined using the standard density-porosity relation: ∅ = ρm-ρb /ρm-ρf (reviewed by Collett, 1998b). In this study, the Archie relation (Archie, 1942) has been used to calculate water saturations (Sw) [gas hydrate saturation (Sh) is equal to (1.0-Sw)] from the available electrical resistivity log data in some of the wells from the Ivik-Mallik-Taglu area (Archie equation: Sw = (a Rw /∅m Rt )1/n; reviewed by Collett, 1998a).
The downhole log derived sediment porosities in the assessed Richards Island wells were relatively high, ranging from about 29 to 39 percent in the permafrost-associated gas hydrate accumulations. Average gas-hydrate saturations within the clastic dominated gas hydrate accumulations were relatively high, ranging from an average value of about 33 to 61 percent. These estimates were used to calculate the in-place gas hydrate volumes (discussed later in this report).
Seismic detection of gas hydrates
In studies of deep marine gas hydrate accumulations, reflection seismic surveys are often used to identify, map, and quantify the occurrence of gas hydrates (reviewed by Dillon et al., 1993). Gas hydrate has a very strong effect on acoustic seismic reflections because it is characterized by very high acoustic velocities (about 3.35 km/sec, reviewed by Collett, 1998a). In comparison, sediments below the zone of predicted gas hydrate stability, if water saturated, have lower acoustic velocities (acoustic velocity of water is about 1.5 km/sec), and if gas is trapped below the gas hydrates, the velocity is much lower even if just a few percent of gas are present. Because of the strong acoustic impedance difference between gas-hydrate-bearing sediments and underlying free-gas-bearing sediments, the base of the gas-hydrate-bearing zone produces a strong seismic reflection. In marine environments this strong reflector at the base of gas-hydrate-bearing sediments has become known as the bottom-simulating reflector or BSR. An additional feature of a gas-hydrate-bearing sediment is caused by the abrupt acoustic velocity decrease at the BSR which is caused by moving from gas-hydrate-bearing sediments above to commonly free-gas-bearing sediments below. This downward velocity decrease, known as an “inversion”, can cause a amplitude phase reversal which can be detected on reflection seismic profiles (reviewed by Dillon et al., 1993). The high amplitude nature of a BSR is often compared to bright spots in petroleum exploration which can be characterized by a phase shift (reversal), velocity pull-down, and strong intrinsic attenuation. It should also be noted that gas-bearing sediments, even in the absence of overlying gas hydrates, often appear as high amplitude anomalies and can be easily identified on seismic profiles from both marine and terrestrial environments.
Until this study, BSR’s or other acoustic seismic anomalies associated with the occurrence of gas hydrates in onshore settings had never been reported in the literature. The lack of reported acoustic seismic anomalies associated with onshore gas-hydrate accumulations is probably due to the geologically complex nature of most terrestrial gas hydrate accumulations. In general, the geology of onshore gas-hydrate accumulations is relatively more complex than the geology of most marine gas-hydrate accumulations. Marine gas-hydrate accumulations, such as those along the east coast of North America, occur within a thick uniform stratigraphic section of mostly silt and clay (reviewed by Collett, 1998a). However, terrestrial gas hydrates, such as those on the North Slope of Alaska (Collett, 1993) or in the Mackenzie River Delta (Collett et al., 1999a, 1999b), occur within complexly interbedded sandstone and shale sequences with the gas hydrate concentrated in the high-porosity reservoir-quality sandstone units. Therefore, a reflection seismic profile across a known terrestrial gas-hydrate accumulation will not exhibit a classical marine type BSR. Instead, the interbedded gas-hydrate-bearing sandstone layers and non-gashydrate-bearing shale layers appear as a series of interbedded high and low amplitude reflectors and in some cases at the base of the gas hydrate stability zone along a given seismic reflector a distinct phase reversal may be detected.
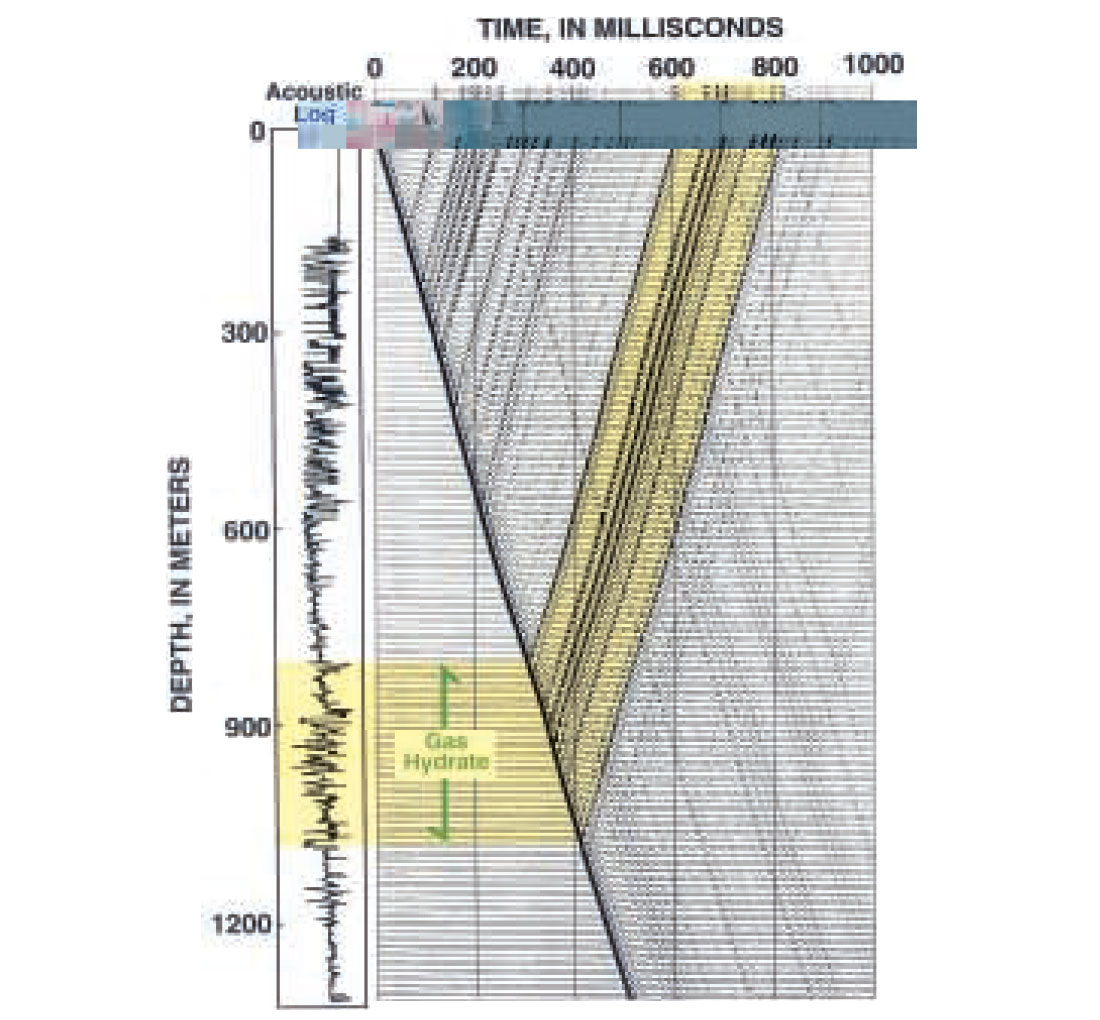
Synthetic seismograms constructed from acoustic transit-time and electrical resistivity logs from the eleven wells examined in this study were used to correlate the surface seismic data with the well information in the Ivik-Mallik-Taglu area (Figure 7). We were then able to interpret regional geology and determine the occurrence of gas hydrate and associated free-gas in the study area more precisely. To further characterize the acoustic velocity structure of the near-surface sediments in the Ivik-Mallik-Taglu study area, we have also generated acoustic transit-time depth profiles (Figure 8) as measured directly from the acoustic log data in nine of the wells examined in this study. The interpolated seismic time-depth plots in Figure 8 show a relatively consistent velocity structure with depth in the upper kilometer of sediment in the Ivik-Mallik-Taglu study area.
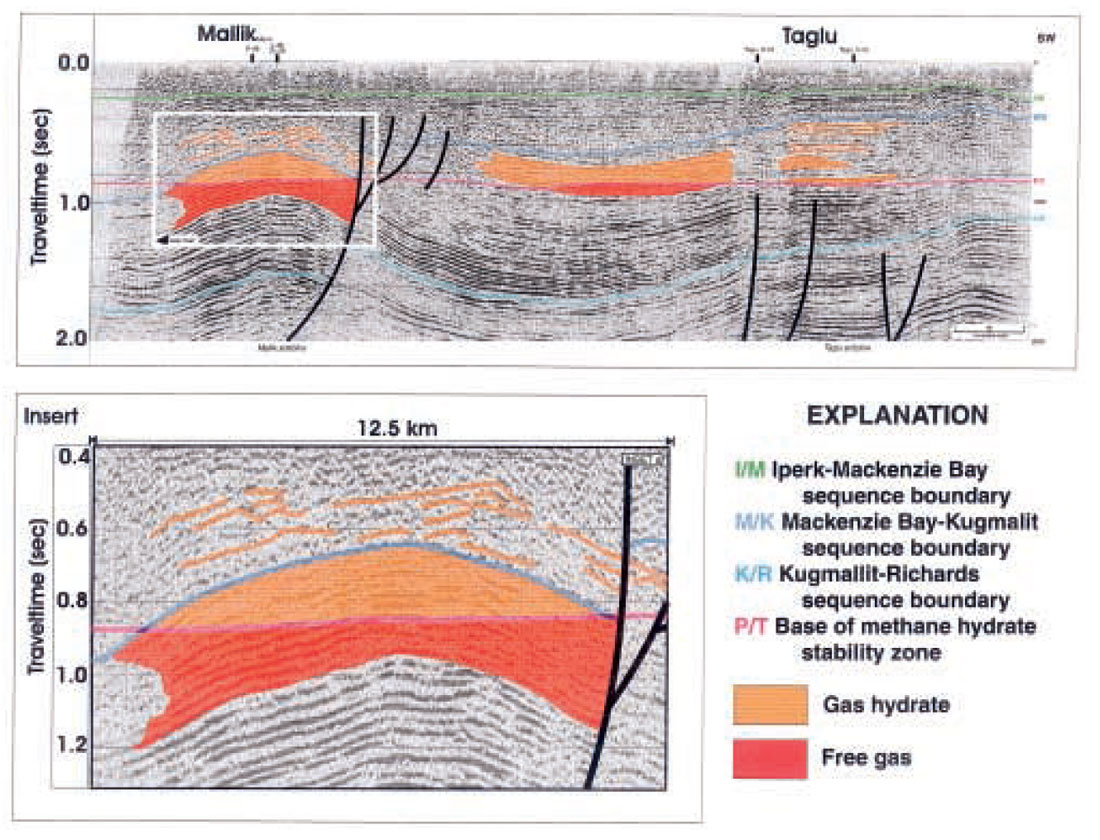
We have thoroughly analyzed all of the thirteen reflection seismic profiles (Figure 2) provided by Imperial Oil Limited. In Figure 9 we have shown one representative seismic profile from the Taglu-Mallik-Ivik area. The sequence boundaries depicted on the seismic profile in Figure 9 have been shown mainly for reference purposes. Beneath the Iperk strata, the Mackenzie Bay and Kugmallit sequences are disrupted by numerous large scale listric faults, with predominantly down-to-basin throws. The throw on these large scale listric faults often exceeds 100 to 500 m. Closer examination of the available seismic data also reveals numerous small scale faults over the crest of the major anticlinal features, which are characterized by small offsets ranging from 20 to 50 m. The other most prominent seismic feature of the Taglu-Mallik-Ivik area are two large southeast-northwest trending anticlines in the area of Mallik and to the north of Mallik where the Ivik C-52 well drilled the crest of a very large anticlinal structure (Figure 10). The Ivik-Mallik-Taglu seismic data also reveals several more subtle anticlinal structures in the area of Taglu (near Taglu C-42 and Taglu G-33) and to the north near the Ivik J-26 and Ivik N-17 wells (Figure 10). For discussion purposes we have named the large scale anticlinal features in the Mallik area and at the site of the Ivik C-52 well the Mallik and Ivik C-52 anticlines, respectively. We have also named the more subtle anticlinal features in the Taglu area and at the site of the Ivik J-26 and Ivik N-17 wells the Taglu and North Ivik anticlines, respectively.
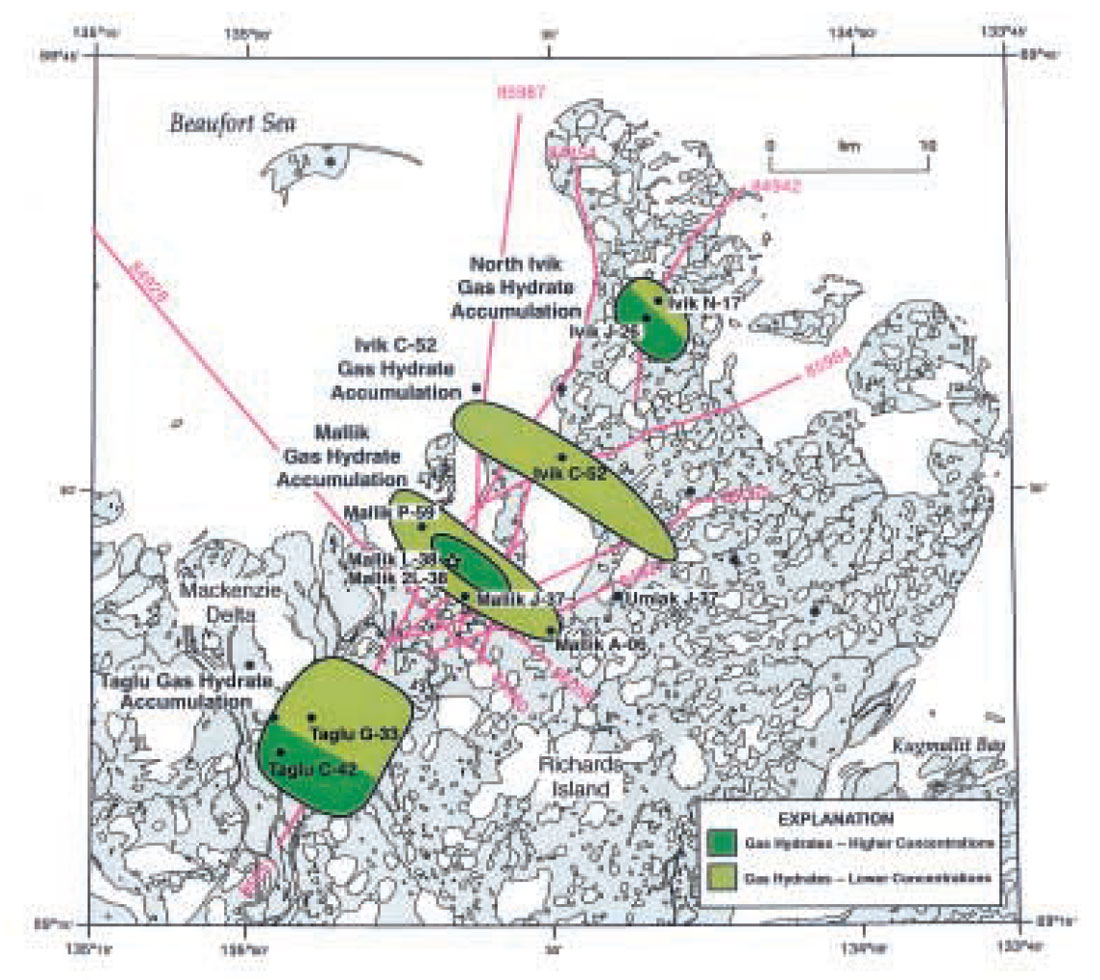
The primary goal of the seismic analyses portion of this study was to directly detect and map the gas hydrate and associated freegas accumulations in the Ivik-Mallik-Taglu area using the available seismic data. The seismic interpretation of gas hydrates and freegas in the Ivik-Mallik-Taglu study area relied on the integration of information on the depth of the pressure-temperature predicted base of the gas hydrate stability zone with the acoustic properties (i.e., amplitude anomalies, phase reversals, etc.) of the sedimentary section imaged on the available seismic profiles. On the representative seismic line in Figure 9 we have shown the base of the gas hydrate stability zone. This phase boundary between gas-hydrates and free-gas has been estimated from temperature profiles in area wells and directly interpreted by the appearance of amplitude phase reversals and other amplitude anomalies at the predicted base of gas hydrate. We have also attempted to identify amplitude anomalies (correlated with well log data interpretations) associated with the occurrence of free-gas and gas hydrates.
At the site of the known gas hydrate accumulations in the Mallik wells, the available seismic profiles reveal numerous high amplitude reflectors (Figure 9) along the crest of the Mallik anticline, extending from a seismic time of about 0.6 seconds to below 1.0 seconds. This relatively thick package of high amplitude reflectors is probably the gas-hydrate- and free-gas-bearing units encountered in the Mallik wells. Of particular interest is the apparent seismic phase reversal associated the base of the gas hydrate stability zone beneath the crest of the Mallik Anticline as shown on the seismic profile in Figure 9. Further review of the other available seismic profiles, also reveals that the well-log inferred gas hydrate accumulations in the Taglu and Ivik areas are characterized by relatively thick packages of high amplitude reflectors associated with the Taglu, Ivik C-52, and North Ivik anticlines. In general, the structural geometry of these structures appears to have concentrated gas, which likely migrated from depth (Lorenson et al., 1999), in the crest of these regional features as gas hydrates. It also appears that both smaller local and large-scale regional faults control the migration and accumulation of hydrocarbon gases on Richards Island.
Our assessment of the regional seismic profiles has revealed the occurrence of significant gas-hydrate related free-gas accumulations along the crest and flanks of both the Mallik and Ivik C-52 anticlines. The apparent high amplitude nature of the seismic reflectors along the flanks of these structures has been used as the primary evidence for the occurrence of free-gas associated with the overlying gas hydrate accumulations. Of interest, however, is the apparent lack of well log inferred free-gas below the Mallik, Ivik C- 52, and North Ivik gas hydrate accumulations. It has been shown that the impedance of acoustic sound waves is relatively insensitive to the concentration of free-gas in a sedimentary section. Thus, the seismically inferred free-gas below the Mallik and Ivik C-52 gas hydrate accumulations are probably at low concentrations as indicated by the available downhole well log data.
Integrated seismic and log data interpretation
The following section of this report includes descriptions of the four well-log and seismically inferred gas hydrate accumulations on Richards Island [i.e., (1) Mallik, (2) Ivik C-52, (3) North Ivik, and (4) Taglu gas hydrate accumulations] and estimates of the volume of gas trapped as gas hydrates in each of the four accumulations. The gas hydrate resource estimates described below assume that one cubic meter of gas hydrate holds 164 m3 of gas at STP.
Mallik gas hydrate accumulation. As interpreted from the available seismic profiles, gas hydrates are inferred to occur along the crest of the Mallik Anticline. The Mallik gas hydrate accumulation appears to be laterally continuous and is estimated to cover an area of about 51 km2 (Figure 10). However, analyses of downhole log data from the Mallik L-38, 2L-38, P-59, and A-06 wells reveal highly variable gas hydrate saturations throughout the Mallik accumulation. Seismic mapping and the analysis of the Mallik L-38 and 2L-38 downhole well log data reveal that the gas hydrates are more highly concentrated within the uppermost crest of the Mallik Anticline. It was determined that the Mallik gas hydrate accumulation contains approximately 110,003x106 m3 of gas in hydrate form.
Ivik C-52 gas hydrate accumulation. The seismic and well-log inferred Ivik C-52 gas hydrate accumulation, as depicted in Figure 10, is also restricted to the crest of a major northwest-southeast trending anticlinal structure. The Ivik C-52 gas hydrate accumulation is estimated to cover an area of more than 46 km2. The Ivik C- 52 gas hydrate accumulation is estimated to contain 42,928x106 m3 of gas.
North Ivik gas hydrate accumulation. The North Ivik gas hydrate accumulation appears to be limited to a series of relatively thin sandstone units at the crest of the North Ivik Anticline (Figure 10). Our systematic gas hydrate resource appraisal of the North Ivik gas hydrate accumulation yielded a calculated gas volume of 22,851x106 m3 of gas trapped as hydrates in the North Ivik Anticline.
Taglu gas hydrate accumulation. Seismic data from the Taglu area along with downhole log data from the Taglu G-33 and C-42 wells reveal that the Taglu gas hydrate accumulation is restricted to a series of relatively thin sandstone units along the northern flank of the Taglu Anticline. Our integrated assessment of the downhole well log data and reflection seismic profiles from the Taglu area yielded a calculated gas volume of 11,396x106 m3 of gas trapped as hydrates in the Taglu Anticline.
Summary
The primary objective of this study was to assess the distribution and size of the gas hydrate accumulations on Richards Island in the outer Mackenzie Delta. Gas hydrates have been conclusively identified in the Mallik 2L-38 gas hydrate research well and are inferred to occur in an additional eight industry exploratory wells on Richards Island. The integration of industry acquired reflection seismic data with the available open-hole log data from the 11 wells examined in this study documents the occurrence of four distinct gas hydrate accumulations on Richards Island: those being the (1) Mallik, (2) Ivik C-52, (3) North Ivik, and (4) Taglu gas hydrate accumulations. The total volume of gas (in place) trapped as hydrates in these four gas hydrate accumulations is estimated at 187,178x106 cubic meters.
For the most part, the seismic and welllog inferred gas hydrate accumulations on Richards Island are restricted to the crest of large anticlines where the base of the gas hydrate stability zone intercepts the crest of these structural features. The distribution of these four distinct gas hydrate accumulations appear to be controlled in part by the presence of anticlines that may have acted as conventional hydrocarbon traps. Considering that the gas hydrate accumulations on Richards Island are restricted to conventional hydrocarbon traps and the gas associated with the sampled gas hydrate accumulations in the Mallik 2L-38 well may have migrated from a more deeply buried thermogenic source (Lorenson et al, 1999), it is likely that some portion of the Richards Island gas hydrate accumulations originated from migrated thermogenic gas that was first concentrated in shallow traps as conventional free-gas fields that were later converted to gas hydrate fields in response to climate cooling or changes in surface conditions. The occurrence of gas hydrates in conventional hydrocarbon traps provides us with an exploration model and “play” concept that may be used to predict the occurrence of gas hydrates in unexplored arctic regions.
Join the Conversation
Interested in starting, or contributing to a conversation about an article or issue of the RECORDER? Join our CSEG LinkedIn Group.
Share This Article