Summary
Based on test work over known Ni-Cu sulphide deposits, in Sudbury (Watts A., 1997, King A., 1994) as well as Voisey’s Bay (Balch S.J., 1998), it has become increasingly clear that pyrrhotite hosted sulphide deposits possess conductances on the order of 100 kS to 10,000 kS and even greater depending on percent sulphide and ore body thickness. Using standard time-domain off-time EM surveys, sulphide targets with greater than 1 kS conductances were essentially non-decaying and impossible to detect in the off-time component of the measured field. The introduction of the time-domain step response EM systems resulted in greatly improved discrimination capabilities for these extremely high conductance targets when compared to off-time EM system as well as improved S/N when compared to conventional frequency-domain EM systems (West G.F., 1984, Ravenhurst W.R., 1998). However, the quality of the survey results were very dependant upon the accuracy of the calculated primary field strength at each survey station, which is controlled primarily by the transmitter-receiver geometry. To avoid errors associated with normalization, particularly when surveying with smaller loops in moving loop mode, the introduction of the SQUID B-field sensor to the standard off-time EM system (Foley C.P., 1998) provided a potentially more effective alternative for the detection of relatively small (less than 1.5 million tonne) highly conductive sulphide targets at depths of more than 100 m. This is typical of the geological environment found at the Raglan Ni-Cu-PGE mine in northern Quebec. The purpose of our study will be to evaluate the potential of using time-domain step response EM surveys for exploration at Raglan as well as to compare those results to that of a SQUID B-field sensor when integrated into the timedomain off-time EM survey.
Introduction
The Raglan Ni-Cu-PGE mine is located along the northern tip of the Ungava Peninsula (Nunavik) region of northern Quebec, 65 km south of the Arctic coast between Hudson Bay and Ungava Bay and 1800 km north of Montreal. The mine occurs within the central portion of the Cape Smith Belt, a 375 km long Proterozoic (1.9Ga) volcano-sedimentary sequence formed during the rifting of Superior Province Craton with subsequent accretion. The Ni-Cu-PGE deposits are stratabound to the ultramafic cumulates within lava channel complexes of the Raglan Formation. There are currently ten zones of significant Ni-Cu-PGE mineralization, making up total reserves of 19.5 millions tonnes of 2.87 % Ni and 0.79% Cu (Figure 1). The sulphide mineralization (pyrrhotite > pentlandite > chalcopyrite) occurs as relatively small less than 1.5 million tonne ore lenses (average 400,000 tonnes), with Ni tenors (Ni in 100% sulphide) vary from 5-15% with an average of 12%. Current mining operations are underway at Katinniq and Zone 3 while exploration efforts are ongoing across the 70 km long property most of which has not been explored to depths of greater than 100m.
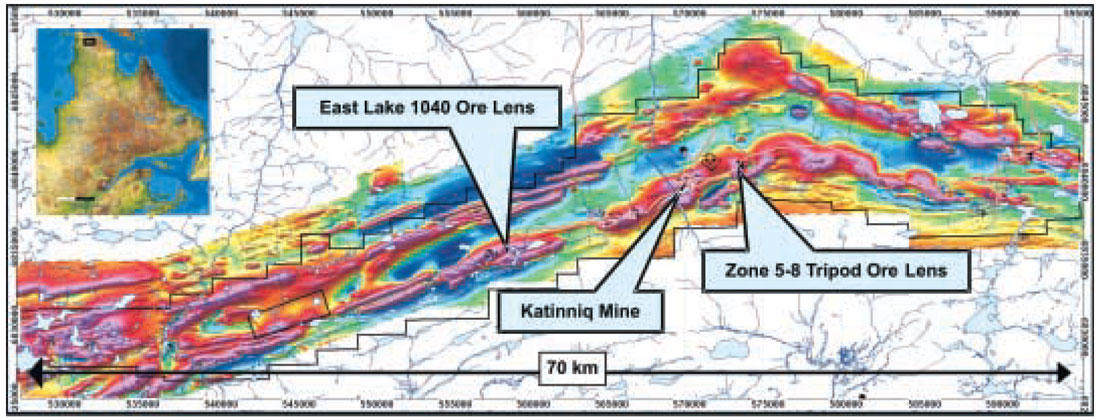
Prior to the mid 1990’s, the level of integration of geophysics into the exploration program was very limited. A series of test surveys were carried out by Tays (1968), in 1968 over existing ore lenses in the Katinniq mine area using IP, HLEM, Turam large loop EM, total field magnetics, and seismic methods. The results of the test surveys, mainly showed that the ultramafic rocks hosting the Raglan Ni-Cu-PGE ore lenses had much higher magnetic potential field values relative to the surrounding country rocks of the Raglan Formation and that horizontal loop and Turam EM methods were the most effective for outlining existing near surface Ni-Cu-PGE sulphide mineralization.
In subsequent years, total field magnetic surveys were carried out in conjunction with HLEM to target shallow EM conductors coincident with the highly magnetic ultramafic bodies. In 1988, continuing with the same exploration strategy, a 6000-line km helicopter- borne EM and total field magnetics survey was also carried out over the entire Raglan property at flight line separation of 100 meters. The survey provided a quality total magnetic field plot of the entire Raglan property and continuous EM coverage needed to target ore lenses to depth of up to 100 m. The survey results were used extensively over the next 10 years to target areas for follow-up ground HLEM and drilling.
With the start-up of the Raglan mine operations in 1997, a new exploration initiative was also established to explore for larger ore lenses of greater than 1 million tonnes to depths of up to 300 m and to depths of 500 m around existing mine infrastructure. This required a drastic change in the exploration strategy and resulted in a much larger geophysical contribution. As part of the initial geophysical program, borehole geophysical surveys were carried out on all drill holes to target ore lenses occurring less than 200m from existing hole. Rock properties data were acquired systematically to help define variations in physical properties of known rock types as well as variations in conductivity from low to high percentage sulphides. A series of MAG3D inversion models were also developed at 100 m and 50 m cell resolutions to help map out the regional scale distribution of ultramafic rocks and to explore for more detailed potential footwall embayment structures or sulphide traps along the base of these ultramafic units.
To this point, however, all ground and airborne geophysical EM methods tested at Raglan had failed to detect any ore lenses located at depths of greater than 100m. A second 3000-line km deeper penetrating time-domain airborne GeoTEM Deep survey was also carried out in 1998 over the entire Raglan property at a line separation of 200m. At this stage, B-field readings were provided along with standard off-time dB/dT EM data as part of the standard survey specifications (Smith R.S., 1998). The purpose for the GeoTEM deep survey was to outline greater than 1 million tonne sulphide targets at depths of more than 100 m. During this time, the processing and measuring of the B-field were in the early stages of development and were found not to provide the resolution needed in the highly conductive Raglan environment to detect new deeper sulphide targets as well as existing ore lenses located at depths of greater than 100m. As a result, recent exploration programs were limited to systematic fence drilling with follow-up borehole EM surveys. This strategy proved very expensive with little overall success. The integration of a cost effective surface EM program, capable of detecting Raglan style ore lenses at depths of greater than 100m, was thought to represent a critical component missing from the Raglan exploration program.
Methods
Tuning EM to Target
The initial stages of surface EM testing involved the generation of simple plate models for known ore lenses based on drill hole geology, surface and borehole EM and rock property results. Standard EM methods were assessed based on conductance discrimination as well as the depth of penetration capabilities of the system. Based on these criteria, it was decided that test surveys would be carried out using the time-domain EM system in large fixed loop and moving loop survey modes. The late off-time results were also compared to the late channel calculated step-response (Ravenhurst W.R., 1998). Initial models, using large fixed loop surveys in Slingram and in-loop modes, suggested that it was possible to detect Raglan type moderate to high conductance targets to depths of greater than 200m. However, with the addition of large moderate conductance hanging wall and footwall regional conductors, large fixed loop surveys proved inadequate due to saturation of the late channel decays by decays from these well coupled regional conductors. Moving loop models were then tested in Slingram and in-loop modes and showed significant improvements at minimizing the effects from these large regional conductors while enhancing the secondary field response associated with the more confined high conductance sulphide targets.
To test these models, field test surveys were carried out initially over the East Lake 1040 ore lens, a 1.2 million tonne sulphide lens located from 100 to 200 m depth. The surveys were conducted in large fixed loop and moving loop survey modes using the Crone time-domain EM system at standard base frequencies of 15 Hz. Step response processing was carried out on each station using post-processed GPS data accurate to within 2-3 meters. The late off-time survey results agreed with existing models in both large fixed loop and moving loop survey modes and were effective at outlining the 1040 ore lens at a depth of greater than 100m. The late off-time response from the moving loop survey provided the clearest anomalous response; however, preliminary step response results were significantly affected by poor geometric controls.
Adding the SQUID B-field Sensor
At this stage, a test survey was carried out with a prototype SQUID B-field sensor developed by CSIRO of Australia. The B-field sensor was integrated into the Crone time-domain EM system by a simple replacement of the Crone receiver coil. The sensor was initially tested over the 1040 ore lens and run in parallel with the standard Crone dB/dT induction coil sensor in both large fixed loop and moving loop survey modes. The SQUID B-field results were compared directly to the coil dB/dT off-time as well as the derived calculated step response. Initial tests were completed at standard base frequencies of 15 Hz. In moving loop survey mode, the late channel B-field results showed a significant improvement in S/N to that of the calculated late channel step response but only minor differences to that of the late channel coil response at 15 Hz. A second test survey was carried out in moving loop survey mode at a base frequency of 5 Hz. The B-field results from this survey outlined a subtle, potentially deeper, longer time constant anomaly superimposed on the more obvious shorter wavelength shorter time constant anomaly visible in both the B-field and coil response data. This result suggested that a lower base frequency was required to detect the deeper long time constant massive sulphide portions of the 1040 ore lens located at a depth of 200m (Figure 2).
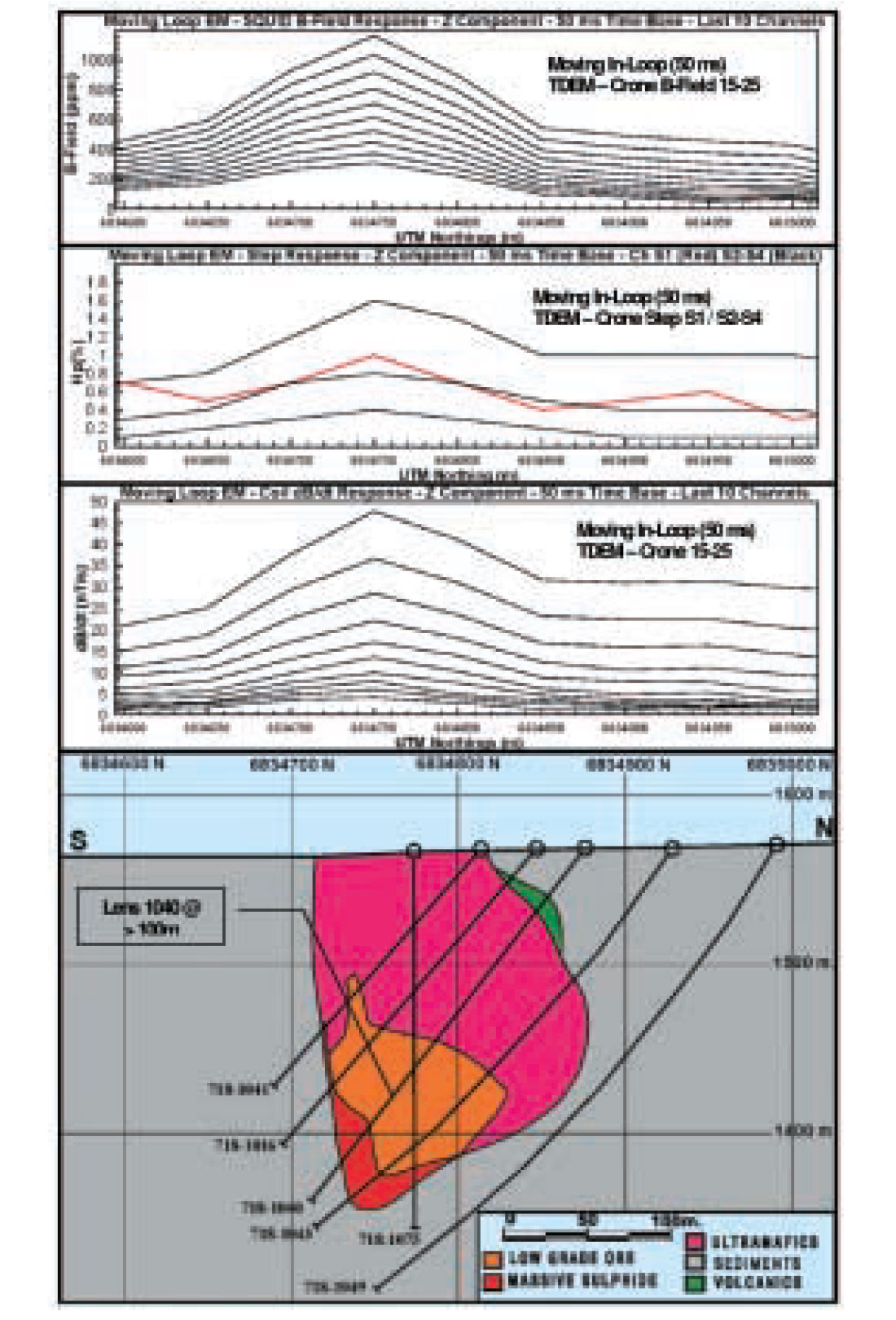
The 1040 ore lens field trials, as well as previous work on SQUID B-field sensors, indicated that the real advantage to using the SQUID lay in the ability to measure the B-field at substantially lower base frequencies while maintaining a good S/N ratio (Foley C.P., 1999). However, surveying at frequencies of 5 Hz or less proved challenging from a production point of view, where readings were taking on the order of 30 minutes per station. To facilitate this problem, Crone agreed to finalize the development of a new 24-bit receiver capable of taking readings at up to 16 times the rate of the existing Crone receiver. The new receiver provided the recording rates necessary to drop the base frequency to less than 5 Hz while achieving standard survey level productions.
To test this idea, a second set of moving loop test surveys were carried out at 5 Hz and 1.67 Hz over the Tripod ore lens, located at Zone 5-8, approximately 5 km east of the Katinniq mine. The Lower Tripod ore lens is a smaller 800,000 tonne highly conductive massive sulphide body located between 120 and 180 m depth. Again the B-field results were significantly better in S/N to that of the calculated step response (normalized to the theoretical primary field using GPS survey data accurate to within 0.6 of a meter), and outlined a subtle long time constant anomaly directly over the lower Tripod ore lens which was not recognized in the late channel coil dB/dT off-time and step response data at 5 Hz. The survey was later repeated without the Crone dB/dT induction receiver coil at a base frequency of 1.67 Hz. This survey proved even more effective and outlined the Lower Tripod ore lens as a long time constant anomaly clearly visible into the late 150 ms time channels (Figure 3).
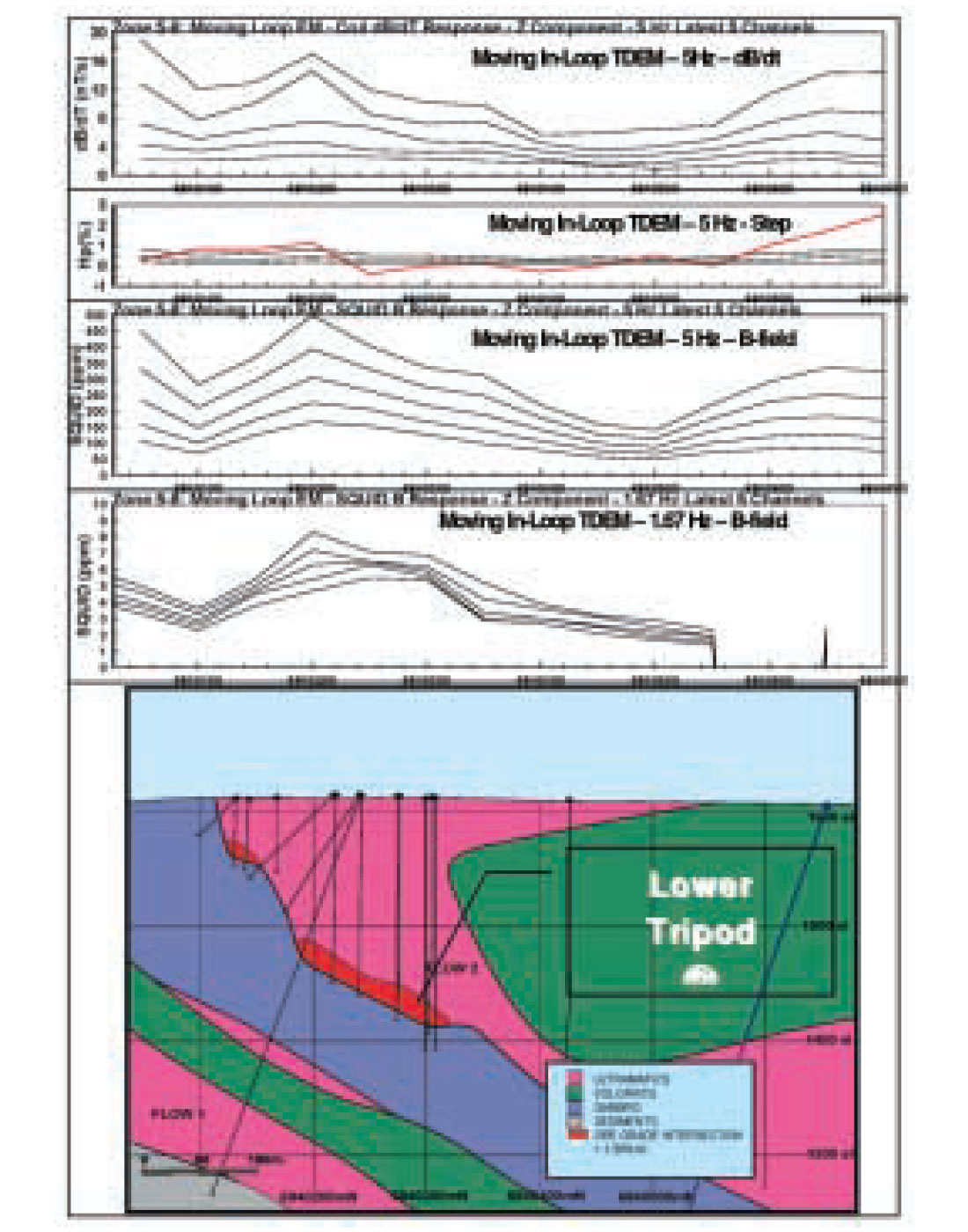
Conclusions
Based on the test results over the 1040 and Tripod ore lenses, the moving loop EM survey configuration provided the most effective approach to exploring for Raglan type ore lenses in low resistivity geological environments at depths of greater than 100m. However, measuring the step response, while providing the conductance discrimination capabilities needed to detect high conductance pyrrhotite sulphide targets, lacked the level of S/N required to detect these one million tonne sulphide targets at depths of greater than 100 m. The addition of the SQUID B-field sensor to the standard time-domain off-time EM system appears to have been the logical progression towards acquiring better quality EM results with improved S/N while providing the conductance discrimination capabilities needed to detect small, long time constant (>>10ms), pyrrhotite hosted, Ni-Cu sulphide targets at depths of greater than 100 m.
Acknowledgements
The author would like to thank the Falconbridge Raglan Exploration team and Geophysical Services Department for providing the support and having the patience needed to make the development of this promising technology a reality. The author would also like to thank Bill Ravenhurst of Crone Geophysics & Exploration Ltd. and his support staff, particularly Ryan Kilty, for their efforts and support, Cathy Foley of CSIRO and the support staff, particularly Keith Leslie, for their hard work over the past two years, and Ken Witherly of Condor Consultants Inc. for his initial help in researching SQUID technology, and his encouragement and continued interest along the way.
Join the Conversation
Interested in starting, or contributing to a conversation about an article or issue of the RECORDER? Join our CSEG LinkedIn Group.
Share This Article