Rock physics describes a reservoir rock using physical properties such as porosity, rigidity and compressibility; these properties affect how seismic waves physically travel through the rocks. The rock physicist seeks to establish relationships between these material properties and the observed seismic response, and to develop a predictive theory so that these properties may be predicted from seismic data.
Rock physics has become a crucial tool in quantitative seismic interpretation over the last 30 to 40 years. Even though it is considered to be a niche discipline amongst the oil and gas community, it spans the entire oil and gas exploration and exploitation lifecycle. Understanding which properties influence the seismic amplitudes is of outmost importance for play de-risking. In order to constrain the de-risking process, some prior knowledge of geophysics, petrophysics, geopressure and geomechanics is needed. Complications arise due to the inherent differences across basins and geographical distance, the variability of the rocks, and differences in the burial and diagenetic histories of the rocks. These differences make it challenging to see the universality of the various elastic parameters across basins, prompting the use of analogues and empirical models.
If a pragmatic view is taken, it is possible to simplify rock physics models based on an understanding of three important parameters that drives the elasticity in the sub-surface: temperature, pressure and gravity. Whilst gravity is (for the sake of argument) constant, we know that both temperature and pressure can fluctuate considerably, and that these variations impact the elastic properties of rocks.
We discuss in this paper how these uncertainties can be constrained and integrated into a rock physics model. This enables us to predict the elastic properties of both shales and sands of different ages and depths, and has applications to un-drilled areas offshore Newfoundland and Labrador, as well as to other frontier basins where well control data are sparse.
A primer on rock physics models
As previously stated, the primary purpose of rock physics models is to relate the seismic elastic rock properties to the rock properties measured in the well. There are many types of models including purely theoretical, empirical, heuristic and bound filling models. Within each of these groups, there are many more models, each of which has its own areas of applicability, pros and cons. For instance, empirical models may be used during early exploration, or in frontier areas where the need to start to understand seismic behavior is the first step in de-risking the prospect. However, empirical models may not add much value during the formal de-risking process when the seismic encounters geology not used in the model building. At the same time, more advanced and rigorous physics-based models, with their many input parameters, can be too uncertain to use when it is impossible to constrain all of the model parameters without calibration points from well data and/or laboratory measurements.
We choose to use a hybrid approach, where we combine theoretical, empirical and heuristic models to describe medium-to-high porosity clastic sediments. Three commonly used heuristic hybrid models are the friable-sand model (Dvorkin and Nur, 1996), the contact-cement model (Dvorkin and Nur, 1996), and the constant-cement model (Avseth et al., 2000). A thorough explanation of these models may be found in Avseth et al. (2010).
Universality of elastic properties – rock physics analogues from offshore Newfoundland and offshore Norwegian Sea
The concept of having common rock physics relationships, regardless of distance, time and geology was explored by Dvorkin et al. (2002) in the paper On the Universality of Diagenetic Trends. This paper shows that air and wind, gravity, pressure, and temperature are the main natural forces that affect sediments all over the world. By incorporating these forces into our rock physics models, we are in a better position to make models that may be extrapolated from one area to another.
Figure 1 show data from the North Sea (grey), and from the Gulf of Mexico (black). The upper trend indicates the chemical compaction trend of sands, and the lower trend is the mechanical compaction trend of shales. One can argue that the abrupt change in gradient of the modulus of the lower trend line is associated with the smectite to illite transformation, and that the rapid increase in modulus following the upper trend line is associated with quartz cementation at the grain contacts for sands.
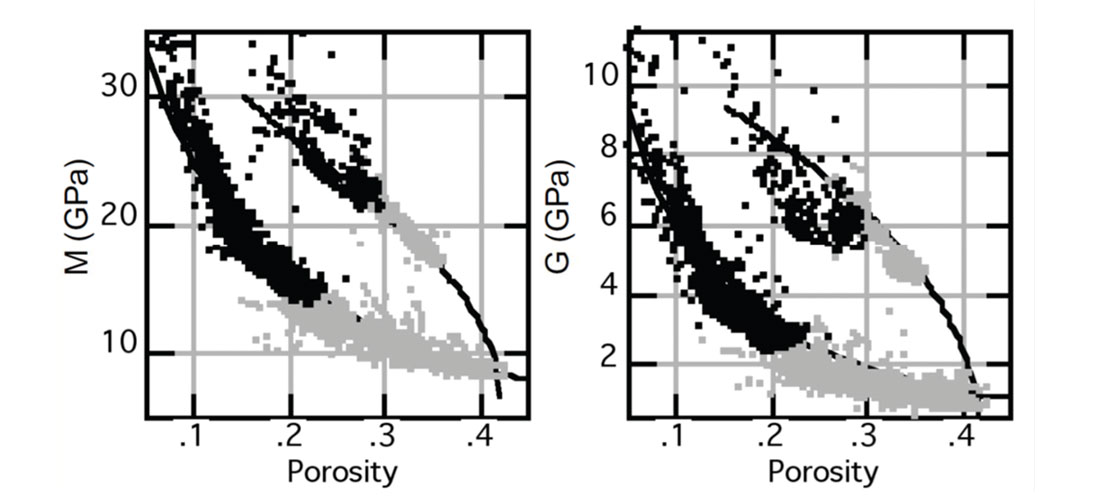
Similar characteristics for sandstones of various depths and geological ages were shown in Selnes et al. (2015) using sandstones from Newfoundland and from the Norwegian Sea. Can we find something similar for shales? A common misconception is that shales are too different in origin (pelagic versus hema-pelagic), mineral constituents and texture, to being able to be properly generalized. Certainly, anisotropy has a major impact on exploration, as it impacts AVO behaviour. We would like to make a general and predictable shale trend that takes into account compaction and mineral alteration due to geochemical processes. This would be a very powerful tool if fully generalized. Once a model for the shales has been derived that describes its elastic properties, the effects of anisotropy on interpretation may be understood using forward modelling.
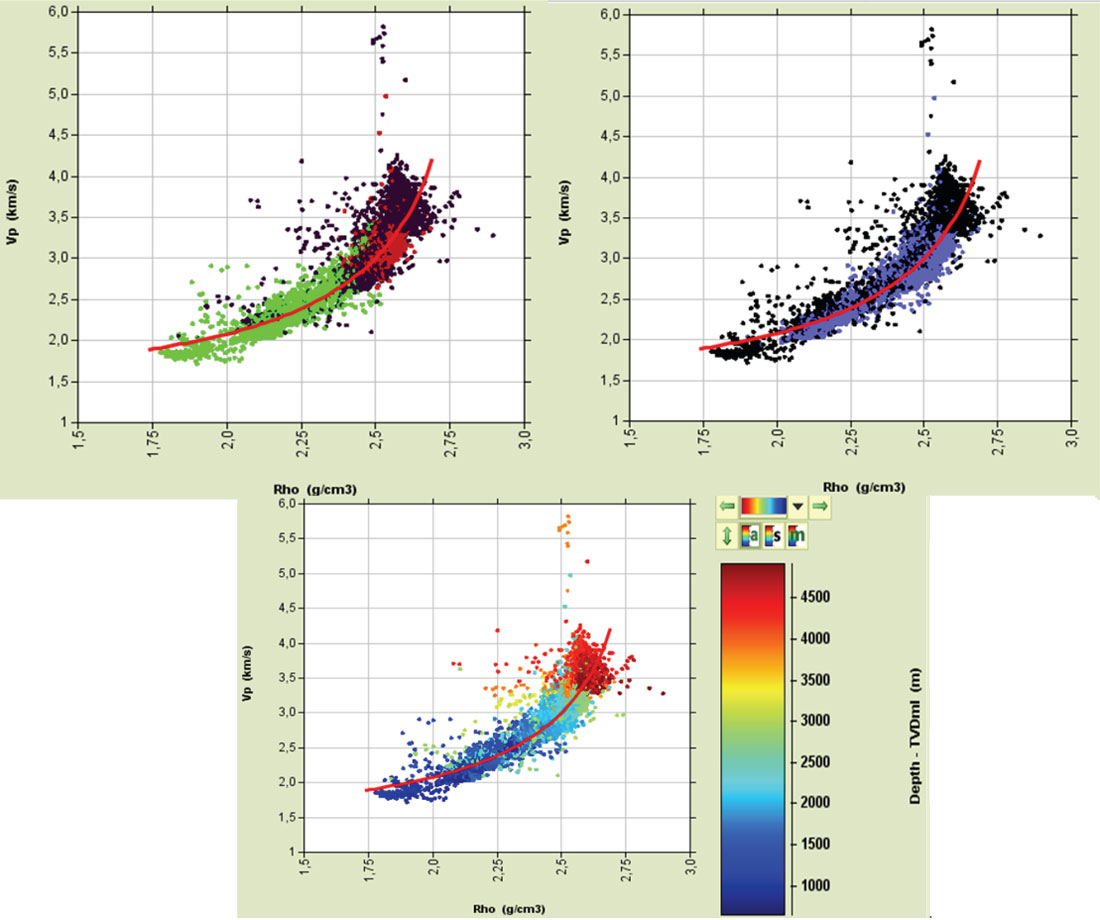
Looking at data from offshore Newfoundland and offshore Norwegian Sea (Figure 2), we see a clear correlation to the data points in Figure 1 for the lower trend, which is normally associated with more shale rich sediments. This suggests that deposition and compaction trends from one location may follow the same effective-medium model as sediments from elsewhere on the planet, regardless of distance or geology. With an effective-medium model for shales (and sands (Selnes et al., 2015)), where the underlying physics is understood theoretically and generalized, we’re in a better shape to make a specific shale trend general for the area of interest by taking into consideration the geology and depositional setting. And, by doing so, we are in a better position to address uncertainties in the de-risking process in new areas where calibration data points aren’t abundant.
Universality of geopressure – pressure analogues from offshore Newfoundland and offshore Norwegian Sea
A recent paper by Green et al. (2014) highlighted the use of analogues offshore Labrador. In this work the authors used recent seismic data to demonstrate the similarity between Labrador and basins such as the Vøring Basin in Mid-Norway (Figure 3). Specifically, the authors showed that deep-water settings generally have a series of common features; these include being highly shale-prone and clay-rich, having less faulting and less uplift. There is not much evidence for additional mechanisms of overpressure generation besides disequilibrium compaction.
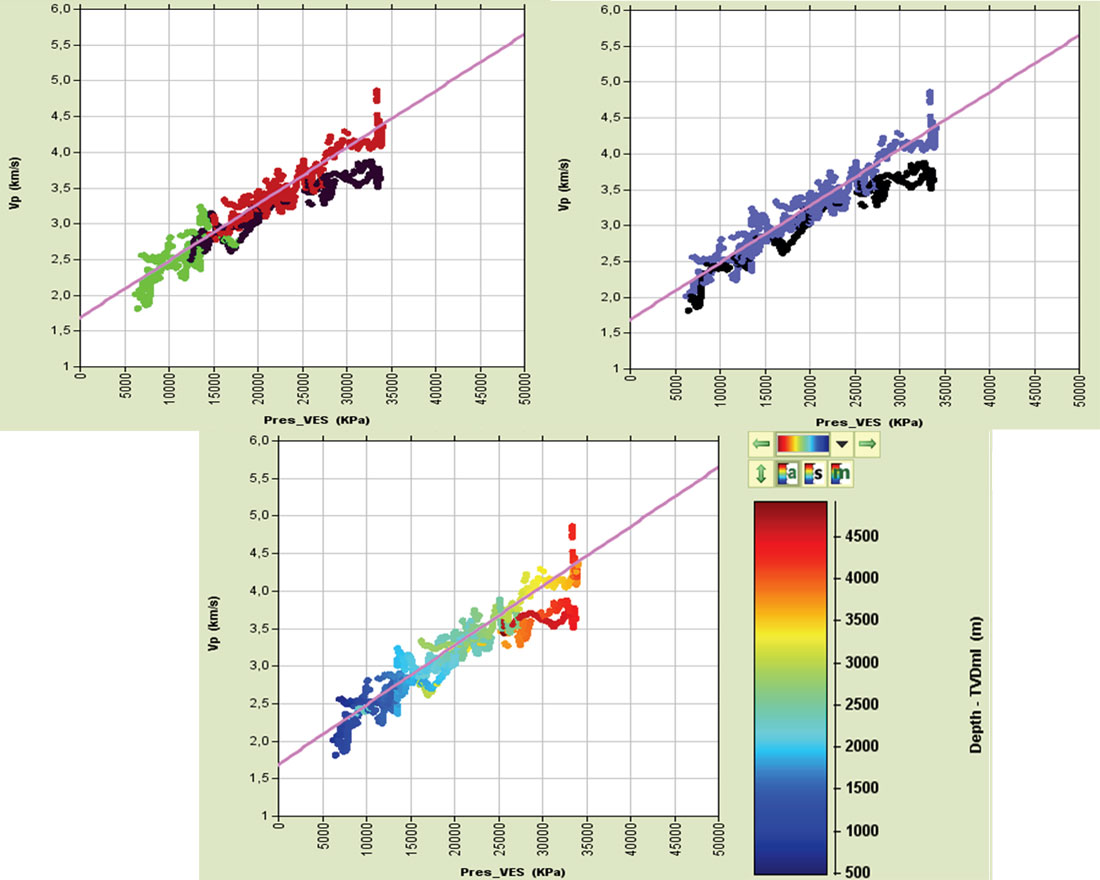
With respect to analogous areas such as the Vøring Basin in Mid-Norway, shale lithologies dominate, and many reservoirs form stratigraphic traps where the sands have the same pressure as the shales. Regional pressure trends can be defined. Deep-sea fans are also visible on the seismic. In the case of the latter, the feeder channel acts as a pressure release valve allowing the sands to de-pressurize, creating a mobile aquifer. Similar deep-water hydrodynamic fan systems are reported in the Tertiary of the Central North Sea (Dennis et al., 2005), although current water depths are shallow. Here, hydrodynamic trapping results in tilted fluid contacts. Enhanced seal capacity is also a feature, as is primary migration out of source rocks.
Applications to Frontier Basins
With a good understanding of rock properties and how they behave and evolve during burial at increased temperatures and pressures, we can start to build rock physics models and better understand seismic amplitudes (Figure 4). We are able to make transforms that use effective stress to back out shale velocities (and shale densities) if the overburden and pore pressure gradients are known in the area of interest, and compare, for instance, to seismic interval velocities.
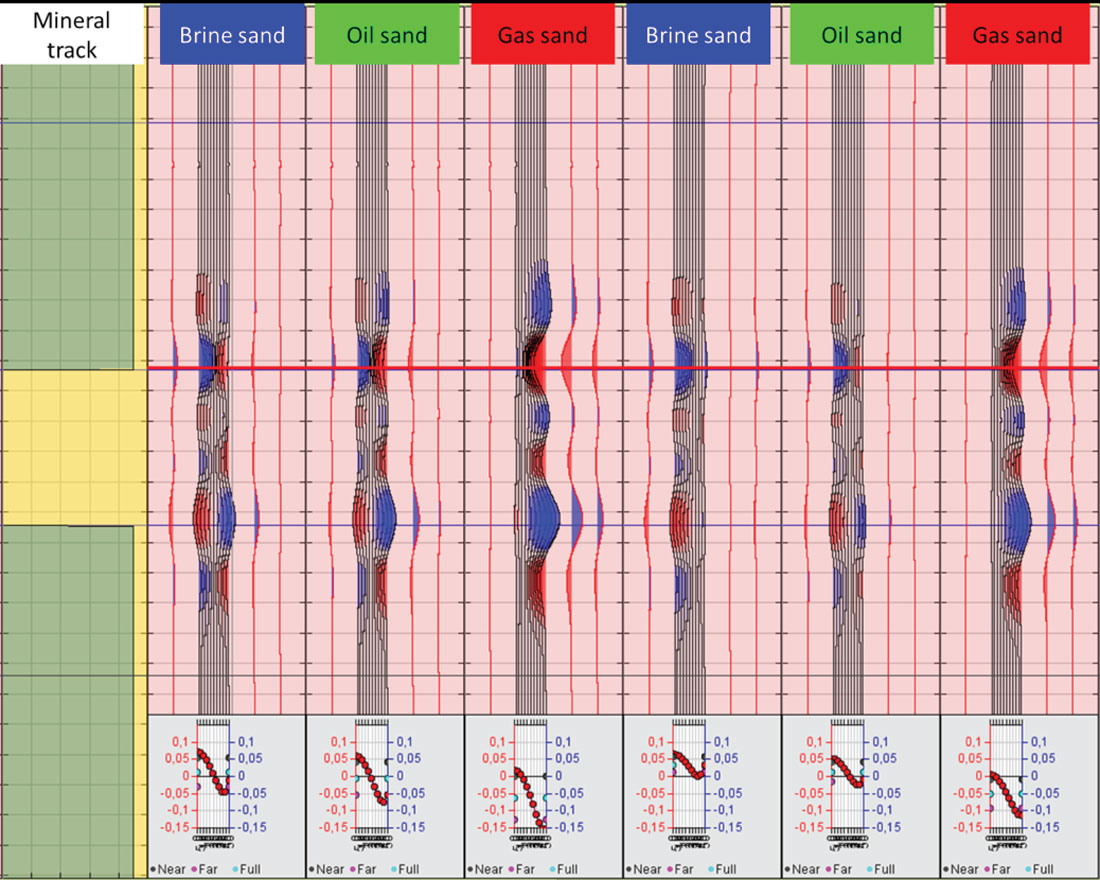
Figure 4 shows what happens if we have potential target sand, encased in shales that follow the model we have established – gather tracks 2, 3 and 4 indicate an abnormal shale for the expected depth (due to disequilibrium compaction); gather tracks 5, 6 and 7 indicate a normal shale for the same burial depth. Using the same shale model, we see differences in the gather response which is mainly due to the differences in acoustic impedance and Vp/Vs ratio between the two shale cases. This is used to build a catalogue of AVO responses targeting the AVO anomaly of interest.
Conclusions
With analogues and effective-medium models, we can extrapolate rock physics models to new areas with more confidence than before. We gain confidence in this due to the resemblance of rock properties and geopressure relationships from two widely different areas. This enables us to more confidentially predict a narrower range of rock properties that cause seismic anomalies away from well control, which are confined within a geologically sound framework.
Acknowledgements
We would like to thank Nalcor Energy and Wintershall Norway for permission to show their data in this paper.
Join the Conversation
Interested in starting, or contributing to a conversation about an article or issue of the RECORDER? Join our CSEG LinkedIn Group.
Share This Article