Abstract
The prospect of new hydrocarbon production as well as gas pipelines from the Beaufort-Mackenzie region, and from Alaska has resulted in increased attention to the substantial earthquake hazard in the Yukon and westernmost Northwest Territories. In this article we describe the distribution of past earthquakes and the hazard estimates based on the earthquake data file of the Geological Survey of Canada, and geophysical and geological constraints. There is exceptional seismicity in the S.W. Yukon where the Yakutat terrane is colliding with the Pacific continental margin in the Gulf of Alaska. The collision is pushing up the spectacular St. Elias Mountains including Mt. Logan, Canada’s highest mountain. The region has one of the greatest seismic hazards in Canada, with numerous recorded magnitude 7 to 8 earthquakes. Less well appreciated is the strong seismicity in the Mackenzie Mountains, Richardson Mountains, and the Beaufort Sea regions, with a number of magnitude 6 to 7 recorded earthquakes. The earthquake record and new high-precision GPS deformation data support a tectonic model of margin terrane collision driving the whole northern Cordillera Yukon block to the north-northeast at about 5 mm/yr. This motion is accommodated at the Cordillera eastern mountain front by overthrusting of the strong craton, as expressed by mainly thrust earthquakes in the Mackenzie Mountains. The northerly motion also produces frequent strike-slip earthquakes in the Richardson Mountains region, and perhaps the concentration of seismicity in the Beaufort Sea. Although there have been no historical large events under the Mackenzie Delta, there is a possibility that the Delta thrust front is capable of infrequent but large earthquakes. The very wide spacing of seismograph stations in northwestern Canada limits the epicentre accuracies, and correlation of the seismicity with geologically mapped faults is possible only for a few of the larger structures. Even recently, only earthquakes of magnitude above about M3 have been consistently recorded and depth determination has been possible for only a few of the larger recent events; they generally are in the upper crust. With the available earthquake catalogue and other information, hazard maps of ground shaking probability are as yet only very regional.
Introduction
The prospect of new hydrocarbon production and a gas pipeline from the Beaufort-Mackenzie region and a pipeline from Alaska has resulted in increased attention to the earthquake hazard in the Yukon and western Northwest Territories (Figure 1). The substantial seismic hazard is not always appreciated; it was highlighted by the recent M7.9 earthquake on the Denali fault in adjacent Alaska that ruptured almost to the Canadian border. This earthquake generated up to 10 metres of strike-slip displacement. It produced huge landslides and it intersected the main Alaska oil pipeline which survived because of its earthquake resistant design. Because of the very low population density and few earthquake-sensitive structures, the Yukon region has received little special seismic hazard analysis. There are only very widely spaced seismograph stations, and those only since the 1970s. The area has been studied largely as part of Canada-wide earthquake hazard mapping. However, there is sufficient information for initial hazard characterization. To raise awareness of the earthquake hazard, we present a summary of the seismicity distribution, the current tectonics, earthquake statistics, and a seismic hazard map that is a basis for the 2005 earthquake provisions of the National Building Code of Canada.
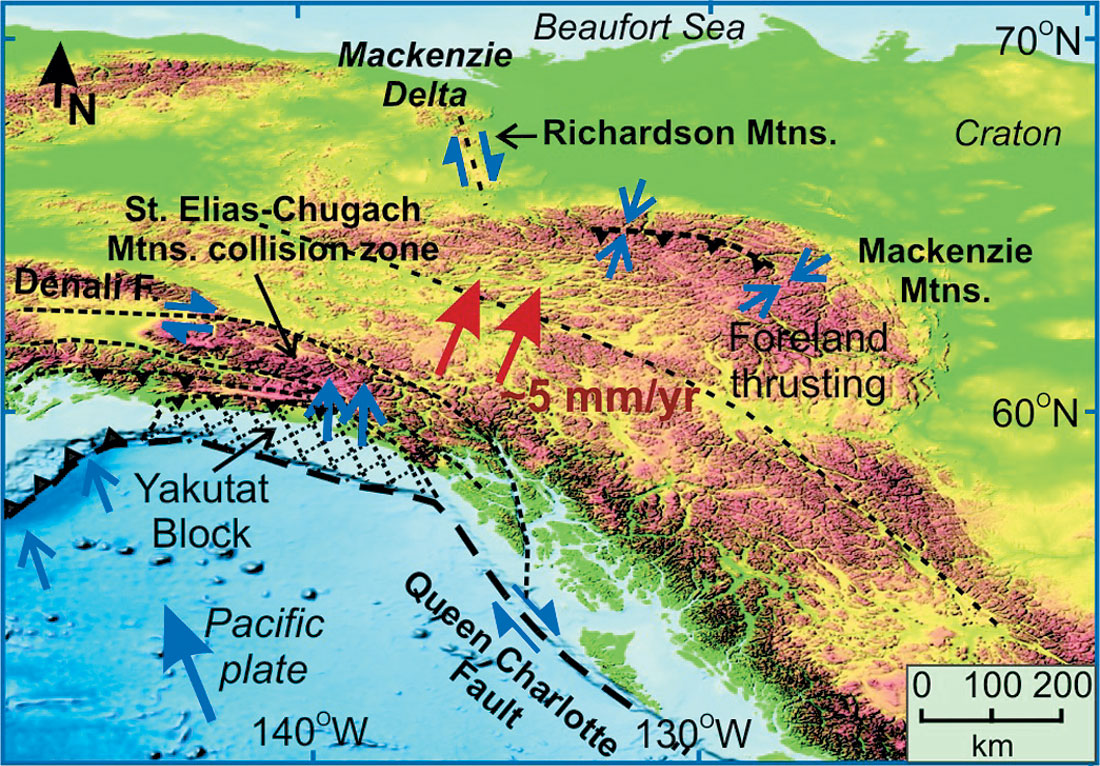
Progress has been made recently in understanding the current tectonic regime that is responsible for the seismicity, and new high precision Global Positioning System (GPS) determinations have been made of the current crustal deformation that is the source of the seismicity (Figure 2). The deformation data are especially important for estimating the hazard from faults or regions that may exhibit very large earthquakes, but few if any small earthquakes. Such large “characteristic” earthquakes may have recurrence times that are very long, such that none have occurred in the period of earthquake monitoring. We provide a description of the regional seismicity and hazard for all of the Yukon and adjacent areas, but with focus on the northern Yukon-Beaufort-Mackenzie region.
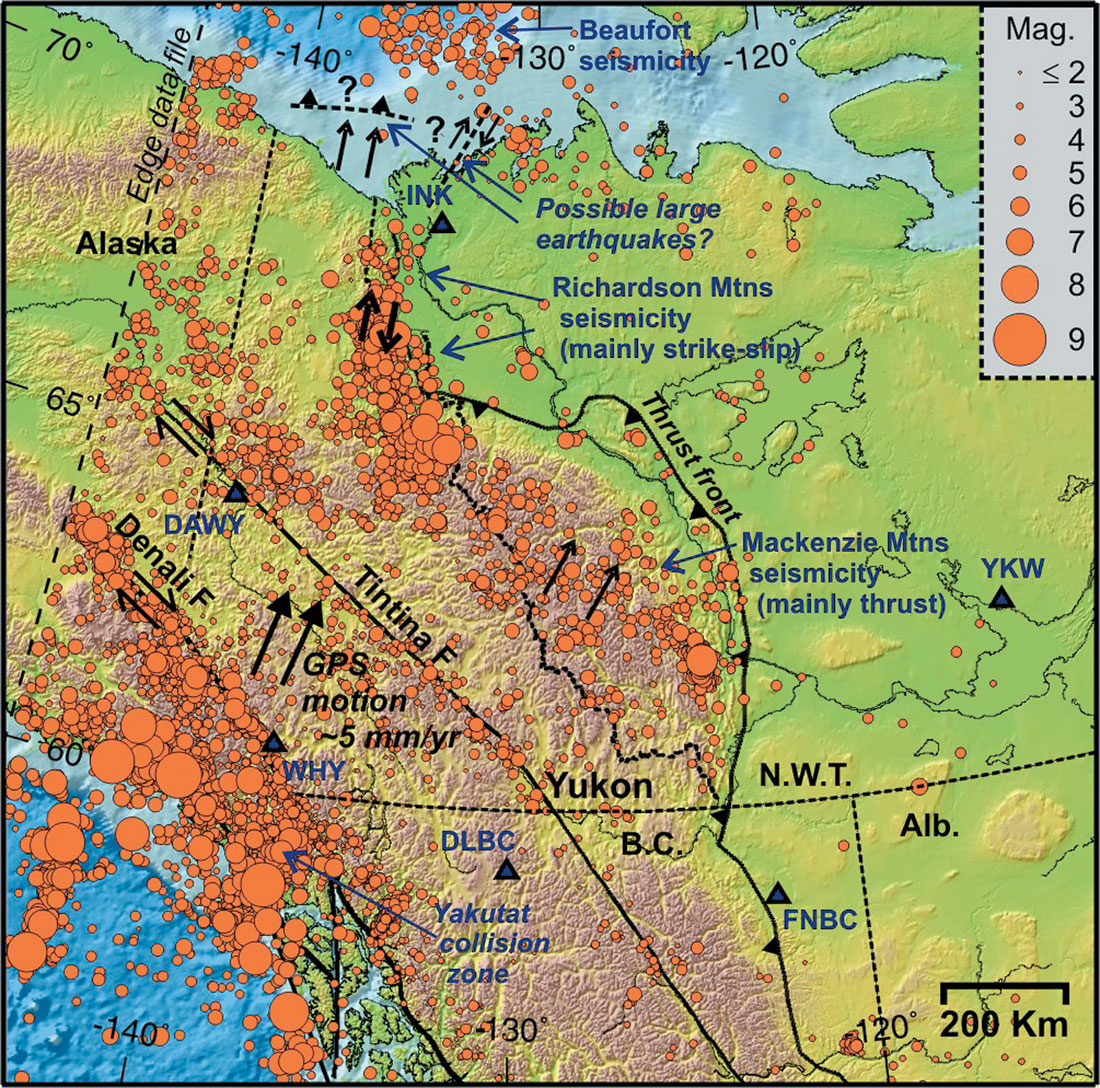
Earthquake Monitoring and Earthquake Catalogue
Instrumental monitoring of earthquakes in western Canada began with the first seismograph in Victoria in 1899. However, only the very largest events could be located until the early-1960s when high gain seismographs began to be installed in western Canada and Alaska such that earthquakes as small as M~5 could be routinely located in the Yukon and western NWT. Even today there are only three permanent stations in the Yukon (Whitehorse, Haines Junction, and Dawson), and two in western NWT (Inuvik and Yellowknife); some additional information is provided by stations in northern B.C. and Alaska (Figure 2). This is a very sparse network with limited epicentral resolution and, except for a few large recent events, with little to no control on focal depths (e.g., Cassidy et al., 2005; see also Leblanc and Wetmiller, 1974).
The primary constraint for earthquake hazard characterization is the distribution and frequency of earthquakes in the earthquake catalogue. Reliable earthquake statistics depend on complete detection of all events for each magnitude used. Since large earthquakes are infrequent, their frequency is poorly defined and their statistics are usually estimated by extrapolating the rate of occurrence of smaller more frequent earthquakes using the Gutenberg-Richter recurrence relation (see below). The estimated dates for when all earthquakes of a certain magnitude began to be recorded in the region are (Adams and Halchuk, 2003): M=5.3 since 1951; M=3.8 since 1972; M=3.0 since 1982. We emphasize that only in the past 25 years has it been possible to locate most minor events (M>3). Routine location uncertainty is generally 10-20 km for land events, and there is little or no depth constraint. The location errors are even greater for the Beaufort Sea earthquakes because of the complex continental margin structure and because they are monitored only by distant land stations.
The large uncertainties in earthquake location, combined with the lack of depth control, makes it very difficult to associate earthquakes with specific faults or other geological structures. However, several detailed studies provide some constraints on earthquake depths and faulting styles.
Earthquake Distribution
The distribution of earthquakes from the Geological Survey of Canada (GSC) data file (Figures 2 and 3) shows the strong seismicity of the northern Canadian Cordillera. Earthquake activity is exceptionally intense in southwestern Yukon and adjacent Alaska and B.C. This is the region of the Yakutat terrane collision that is creating the St. Elias Mountains, including Mt. Logan, Canada’s highest mountain. The region has some of the highest seismic activity in Canada, i.e., several M~8 earthquakes and eight events of magnitude over 7 since 1899. The strong seismicity results in a high seismic hazard in the region of the Alaska highway in southwestern Yukon.
To the east, in the central part of the Yukon Cordillera, there is only minor historical seismicity, including a small concentration that may be associated with the Tintina fault which has been a major strike slip fault through much of the Tertiary. To the northwest, near the Alaska border and into Alaska, there is a greater concentration of seismicity near and to the north of that fault.
The main focus of this article is further to the east in the area of the eastern Cordillera mountain front, where the seismicity is again strong in the regions of the Mackenzie and Richardson mountains, and to the north beneath the Beaufort Sea (Figures 2 and 3). Very few earthquakes have been recorded in the adjacent craton. Although potentially damaging earthquakes, M>6, may occur in that region, they are expected to be very infrequent (e.g., Fenton et al., 2005) and of consequence chiefly to structures with a very high reliability requirement.
The region of the Mackenzie and Richardson mountains has by far the highest seismicity for the whole eastern Canadian Cordillera mountain front, and is in marked contrast to the low earthquake activity in the Rocky Mountains fold and thrust belt south of 60°N (Figure 2). The Mackenzie Mountains are mainly a contractional fold and thrust belt in both earthquake mechanisms and in geological expression. In contrast, the Richardson Mountains have had both shortening and strike-slip motion in the Cenozoic geological record (e.g., Lane, 2002), but the earthquake mechanisms are mainly strike-slip. The large M=6.6 and 6.9 Nahanni earthquakes of 1985 (Horner et al., 1990; Wetmiller et al., 1988) were located in the southern Mackenzie Mountains (Figure 2), and earthquakes of M=6.3 and 6.4 occurred in the mid-1950s in the northern Mackenzie mountains (Cassidy et al., 2002). It is noteworthy that many of the large Mackenzie Mountains events were not located at the mountain deformation front, but rather 50-150 km to the west (Figure 2).
In the southern Richardson Mountains, strong seismicity and earthquakes of M=6,3 and 6.6 have occurred (Cassidy and Bent, 1993) (Figures 2 and 3). The number of events decreases to the north and there is little historical instrumental seismicity in the region of the Eskimo Lakes fault zone toward the Beaufort Sea coast but, as discussed below, there is the possibility that this region could be subject to relatively infrequent but large earthquakes.
There has been a significant number of earthquakes beneath the Beaufort Sea margin to the north of the Mackenzie Delta that are of special concern for offshore exploration in the region. They form a distributed cluster about 250 km across beneath the continental slope, lying between the 200 and 2500 m bathymetry contours. There is about one earthquake of M>4 per year and one M>5 expected about every 10 years (see Hasegawa et al., 1979). There is a strong positive gravity anomaly under the landward edge of the seismicity cluster, and Forsyth et al. (1990) considered that the earthquakes may occur where thick sediments have prograded over oceanic crust. An alternative view is that this cluster, although especially strong, is one of several along Canada’s Arctic and Atlantic rifted margins and may not be special (e.g., Adams and Basham, 1991). There is some evidence that the earthquakes are unusually deep, possibly subcrustal (see Hasegawa et al., 1979; Rogers and Horner, 1991), but the depths are poorly constrained in the offshore region.
Detailed Microseismicity Study
A microseismicity study was carried out in the northern Yukon region in the 1970’s with an array of nine temporary seismographs (Leblanc and Wetmiller, 1974). The study provided more accurate locations for some earthquakes compared to the ongoing catalogue events. However, it employed only short-period seismometers and analogue paper recording, and modern location and analysis techniques were not then available. A total of 27 events of ML=1.2 or greater were recorded, including one M=4.8 earthquake in the Richardson Mountains that was recorded at more distant seismograph stations. The study recognized two cluster of earthquakes, one in the southern Richardson Mountains, and the other in the northern Mackenzie Mountains, in agreement with the distribution for the longer term catalogue events. Both areas have a concentration of mapped surface faults, and have experienced large (M>6) historical earthquakes. Although poorly constrained, the earthquakes in the Richardson area were estimated to be in the mid-lower crust and the M=4.8 event to be mainly strike slip motion along a near-vertical NNW-striking nodal plane.
The Larger Earthquakes
Only two earthquakes in the Beaufort Sea area have been studied in any detail. The largest recorded earthquake was the M=6.5 event in 1920 (Table 1) (Basham et al., 1977; Hasegawa et al., 1979; Rogers and Horner, 1991), and there was a M5 event in 1975. There also have been several other mechanism solutions. They are interpreted to be due to tension normal to the margin, although there is limited constraint. A considerable number of large potentially damaging earthquakes (M>6) have occurred in the northern Yukon-western NWT region (Table 1, Figure 3). A pair of large earthquakes (M=6.3 and 6.6) occurred in the Richardson Mountains in May and June of 1940. A detailed analysis of these events (Cassidy and Bent, 1993) showed that they exhibited strike-slip faulting. They occurred at a depth of 7- 14 km and had no detected aftershocks. It is likely that they were associated with right-lateral motion on the predominant N-S striking Richardson Fault system.
Year | Latitude | Longitude | Mag. | Region |
---|---|---|---|---|
Table 1. Earthquakes M>5.5 in the northeastern Yukon-Beaufort-Mackenzie | ||||
1920 | 72.70 | -130.90 | 6.5 | Beaufort Sea |
1937 | 71.40 | -137.70 | 5.5 | Beaufort Sea |
1940 | 66.87 | -135.33 | 6.3 | Richardson Mtns. |
1940 | 66.85 | -135.80 | 6.6 | Richardson Mtns. |
1952 | 65.61 | -134.85 | 5.5 | N.Mackenzie Mtns. |
1953 | 65.27 | -132.73 | 6.3 | N.Mackenzie Mtns. |
1955 | 65.16 | -133.10 | 6.4 | N.Mackenzie Mtns. |
1956 | 65.22 | -133.47 | 5.8 | N.Mackenzie Mtns. |
1956 | 65.84 | -133.47 | 5.8 | N.Mackenzie Mtns. |
1957 | 65.29 | -133.42 | 5.8 | N.Mackenzie Mtns. |
1957 | 65.34 | -133.50 | 5.7 | N.Mackenzie Mtns. |
1957 | 65.50 | -133.00 | 5.7 | N.Mackenzie Mtns. |
1985 | 62.21 | -124.22 | 6.6 | S. Mackenzie Mtns. |
1985 | 62.19 | -124.24 | 6.9 | S. Mackenzie Mtns. |
1988 | 62.12 | -124.21 | 6.2 | S. Mackenzie Mtns. |
Further to the south, a sequence of strong earthquakes (M=5.5- 6.4) occurred in the northern Mackenzie Mountains between 1952 and 1957. A detailed study (Cassidy et al., 2002) showed that they were quite different from those in the Richardson Mountains just to the north. They had well-defined aftershock sequences, occurred in the upper crust at a depth of 7-15 km, and were associated with thrust faulting along shallow-dipping planes dipping to the NNE.
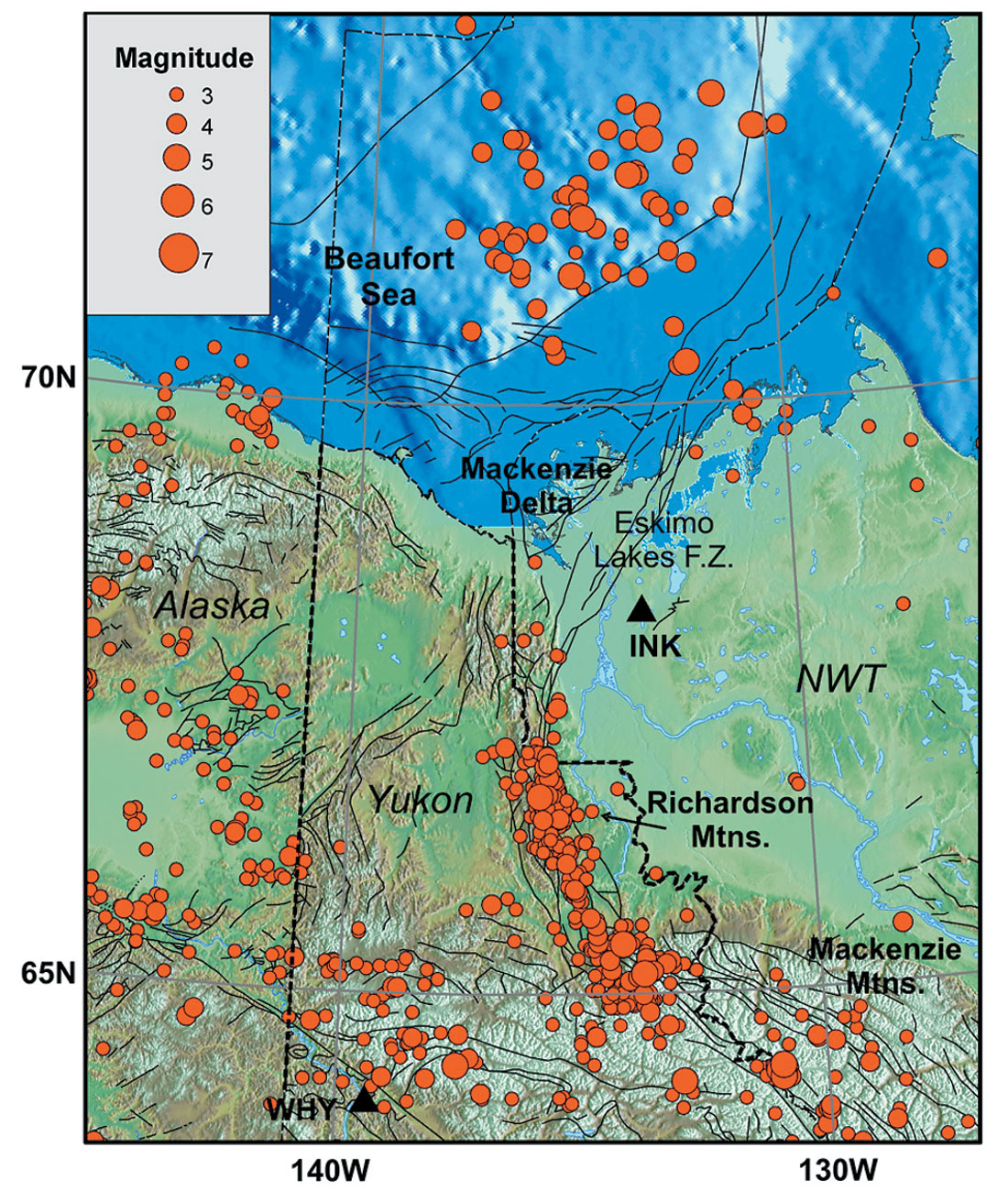
The largest events in the general region were the Nahanni earthquakes (Figure 2), including M=6.6 in October 1985, M=6.9 in December 1985, and M=6.2 in March 1988. The M6.9 was two magnitude units larger than any previous event in the southern Mackenzie Mountains and all were larger than the largest assumed for the 1985 National Building Code, emphasizing that a region with only small past recorded events may still have future large earthquakes. A number of portable recorders were deployed after the first event; they recorded the second and largest earthquake and many aftershocks. The largest event had exceptionally strong local ground motion (Weichert et al., 1986). There have been a number of detailed studies of these events (Horner et al., 1990; Wetmiller et al., 1988), as well as examination of satellite optical and SAR images (Moon et al., 1991). The focal depths were shallow, ranging from 4-10 km, and the earthquakes a re interpreted to have occurred on a reactivated buried Laramide-age fault that extends through the Proterozoic and Paleozoic rocks above the crystalline basement. No surface fault breaks were identified, but this area has no roads and has very difficult access. The preferred focal mechanisms are thrust on north-striking shallow west-dipping fault planes. These events suggest that potentially damaging earthquakes may occur anywhere in the Mackenzie Mountains, Richardson Mountains, and adjacent areas of the northern Cordillera.
Fault Mechanisms, and Stress Directions
The nature of faulting and the directions of regional crustal stress responsible for the earthquakes may be inferred from earthquake focal mechanisms, (rupture plane and slip direction). These have been determined by detailed studies of older paper seismograph records for the larger historical earthquakes noted above (Figure 4). For the more recent events of M>4 that have high quality digital broadband recording, mechanisms have been obtained by Ristau (2004) using a moment tensor routine. The results have been summarized by Cassidy et al. (2005) and Hyndman et al. (2005). The moment tensor method provides earthquake mechanism, seismic moment (related to fault area times average slip), moment magnitude (Mw) and focal depth. In the coastal region of SW Yukon and adjacent Alaska and British Columbia, there are numerous mechanism solutions that show mainly thrust and right-lateral strike slip. The regional stress direction responsible for the earthquakes (earthquake composite stress direction) is nearly N-S (e.g., Hyndman et al., 2005; Cassidy et al., 2005). In the interior of the Yukon there are a few focal mechanisms for events located near the Tintina fault but the fault planes do not align with the Tintina fault. They are a mixture of right-lateral and thrust earthquakes. Near the central Yukon-Alaska border, the mechanisms show NE-SW compression indicating that some of the northerly motion from the Yakutat terrane collision may be accommodated by crustal shortening in this region.
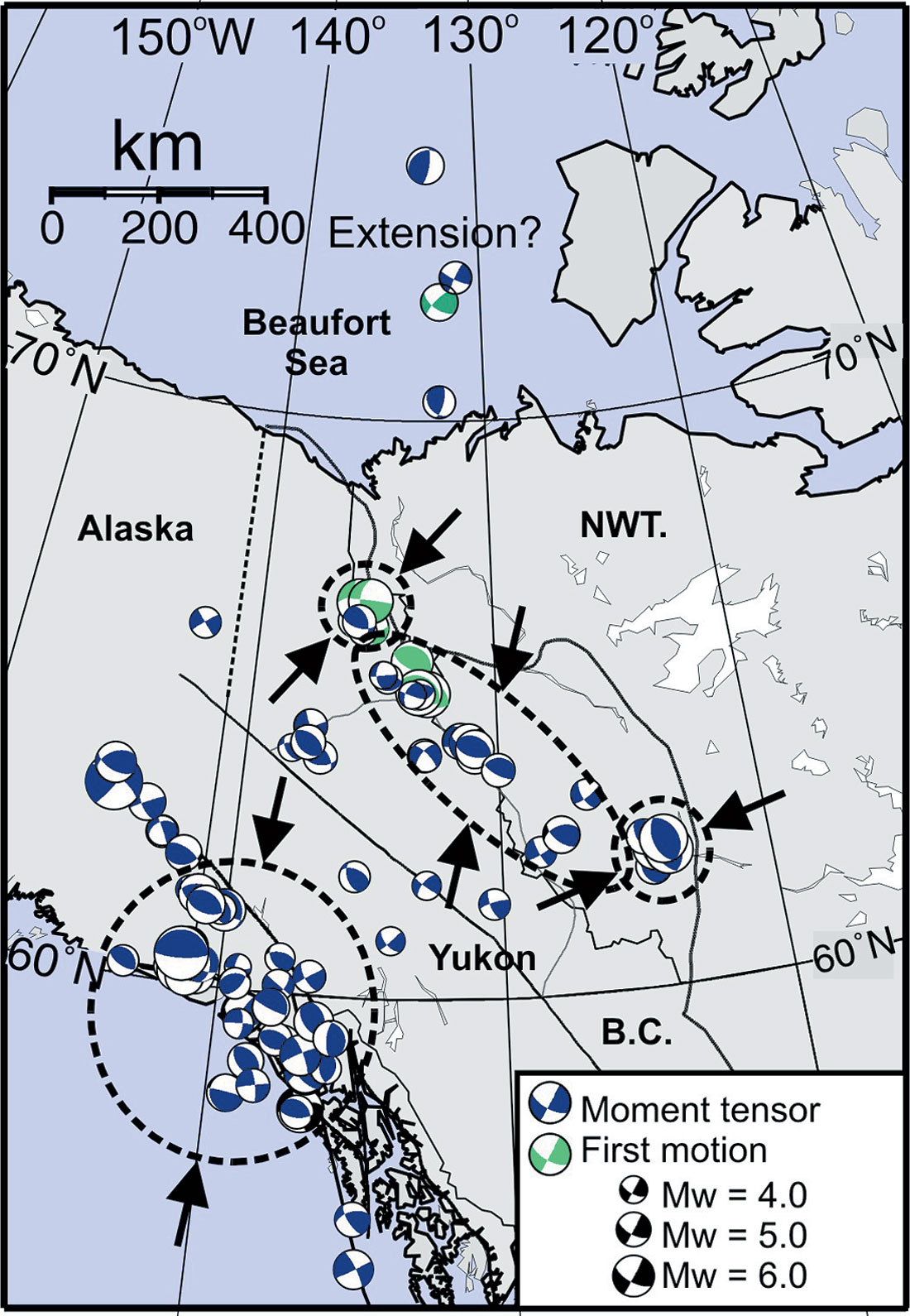
In the Mackenzie Mountains, the mechanisms are mainly thrust with some strike-slip events. In the southernmost Mackenzie Mountains (Nahanni area) the fault planes are oriented N-S in agreement with the orientation of local thrust structures, and the composite maximum stress direction is ENE-WSW (although poorly constrained). In the northern Mackenzie Mountains the mechanisms are a combination of thrust and right-lateral strike slip, and the composite stress direction is NNE-SSW. In contrast, in the Richardson Mountains the focal mechanisms show right-lateral strike-slip faulting, consistent with the Richardson fault system (Figure 4). The pressure axes for these earthquakes is NESW. All of the principal stress directions are in general agreement with the regional stress field that is expected for the Yakutat terrane collision on the Pacific margin and for strain transfer across the northern Cordillera (Mazzotti and Hyndman, 2002 and references therein).
For the cluster of earthquakes beneath the Beaufort Sea, there are only a few mechanisms available. They show normal and strike-slip faulting and a nearly horizontal tension axis. The Beaufort earthquakes therefore do not appear to be accommodating the northerly motion of the Yukon block with respect to the Arctic Ocean lithosphere. These mechanisms are in contrast to the nearly horizontal pressure axes oriented north-northeast observed throughout the northern Cordillera. The Beaufort events are interpreted to occur in the lower crust or uppermost mantle and are unlikely to have a seafloor surface expression. One model for their origin is that the rapidly developing Mackenzie Delta is loading and depressing the oceanic lithosphere. Lithosphere depression is suggested by the very deep sedimentary trough beneath the outer delta (e.g., Lane, 2002). The flexural bending will cause tension in the upper lithosphere that may generate the observed seismicity. Postglacial rebound also may play a role.
Precision GPS Monitoring of Crustal Deformation and Regional Tectonics
High precision Global Positioning System (GPS) and other geodetic data have resulted in a significant recent revolution in earthquake hazard definition. GPS allows mapping of the ongoing crustal deformation that results in earthquakes. In the short term, crustal deformation is accommodated elastically, and in the longer term it is accommodated mainly by large earthquakes (small earthquakes make little contribution to regional deformation, even though they are more frequent). Most present earthquake hazard characterization is based on past earthquake statistics. However, some areas globally have too few small earthquakes to define statistics necessary for extrapolation to the frequency of infrequent large earthquakes. Geodetic quality GPS measurements provide remarkable positioning precision, i.e., a few millimetres. Sites with continuous GPS measurements over a number of years give the highest accuracy but such stations are costly and difficult to maintain in the north. Most data comes from a series of repeated measurements at benchmarks. After a few years of monitoring, crustal deformation rates can be determined to several mm/yr (= geological rate of km/m.y.).
There is limited precision GPS data in the Yukon as yet, but two University of Victoria PhD thesis studies in collaboration with the Geological Survey of Canada have provided sufficient data for initial definition of a regional tectonic model. There is considerable data scatter and uncertainty, but there clearly is a north-northeasterly motion of the northern Cordillera relative to the adjacent craton at a rate of about 5 mm/yr (Mazzotti et al., 2002; Fluck, 2003; Hyndman et al., 2005) (e.g., Figure 2). There probably is also some internal crustal deformation of the northern Cordillera. There are higher rates in the S.W. Yukon. The northerly Yukon block motion is interpreted to be the result of the collision of the Yakutat terrane along the Pacific margin (e.g., Mazzotti and Hyndman, 2002). In contrast, most of the Cordillera of northern British Columbia is moving very little, less than ~1 mm/yr. Deformation is restricted to the coastal region, in agreement with the low inland seismicity.
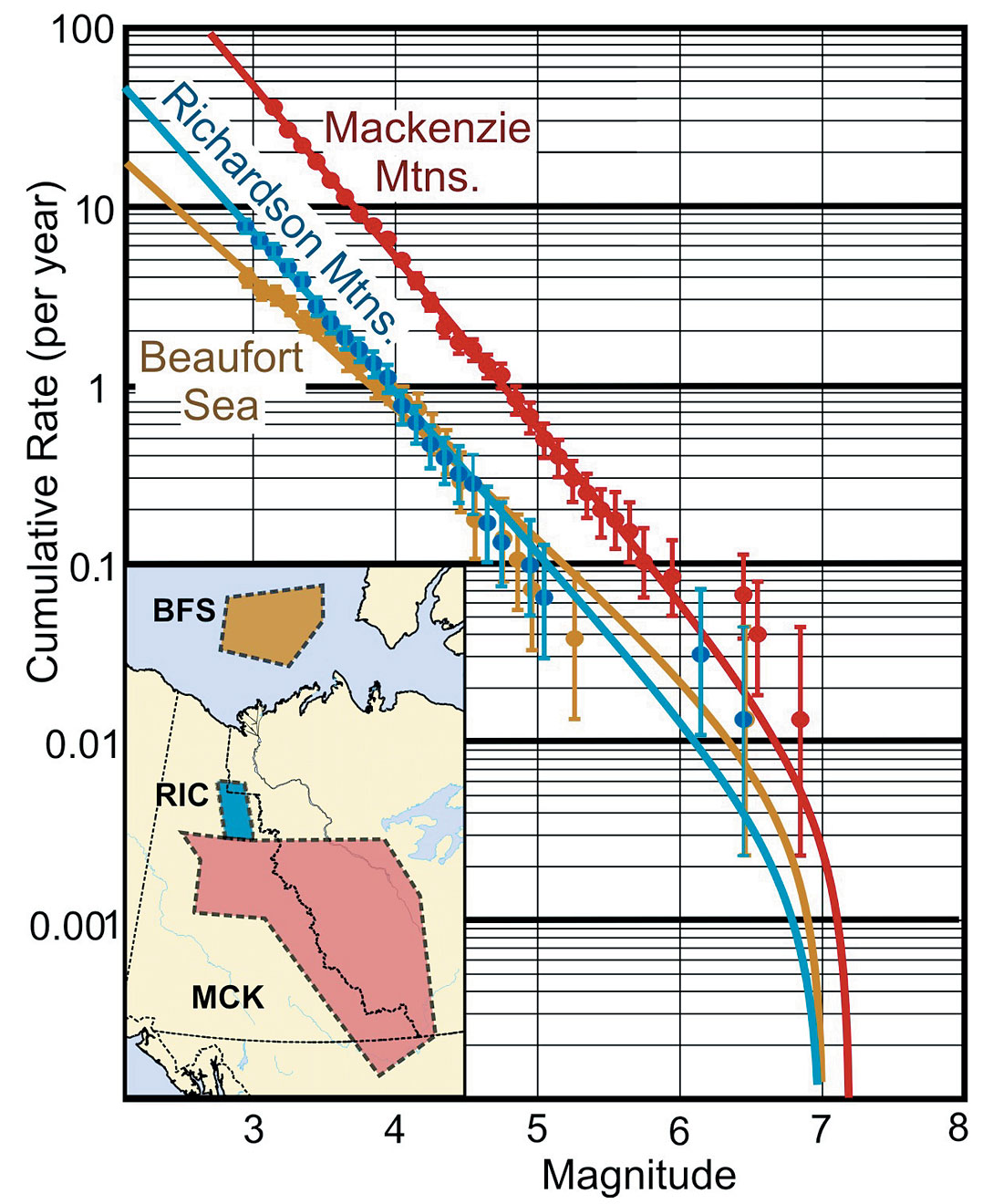
The motion of the Yukon with respect to the craton appears to be accommodated by mainly thrust motion at ~5 mm/yr for the Mackenzie Mountains and a similar rate strike-slip for the Richardson Mountains regions. Much of this northerly motion probably is accommodated in the region of the Mackenzie Delta front. These deformation rates of ~5 mm/yr can be accommodated by magnitude 6 earthquakes at an average frequency of approximately 50 yrs, or magnitude 7 earthquakes every 500 years for each 100 km along the Cordillera. For the whole Yukon eastern Cordillera mountain front (Mackenzie Mountains, Richardson Mountains, Beaufort Sea), the earthquake statistics indicate (Figure 5) an M6 event is expected about every 10 years, and a M7 event every ~ 100 years.
Mackenzie Delta Current Deformation and Possible Large Earthquakes
The strong seismicity in the Richardson Mountains along the edge of the craton declines northward from about latitude 67°N, with few events in the catalogue north of about 68.5°N until the Beaufort Sea concentration north of about 71°N. The simple regional tectonic model that is supported by GPS deformation data suggests that the northerly motion of the Yukon continues to the Beaufort Sea margin. From the seismicity it is not clear where this motion is accommodated. Beginning in the latest Cretaceous, there has been detached folding and thrust faulting in the frontal part of the Mackenzie Delta. The greatest shortening in inferred to have occurred in the Paleocene to early Eocene, but minor deformation has continued regionally to the Holocene (e.g., Lane and Dietrich, 1995). The shortening shows a younging trend seaward with the Holocene-Recent deformation overlying the oceanic crust. The overthrusting and depression of the oceanic crust may be responsible for the thick marginal sedimentary basin (e.g., Lane, 2002), with strike slip motion on the eastern boundary of the Yukon block lying in the area of the Eskimo Lakes fault zone. To the west in Alaska, the seismicity pattern suggests that the N-S shortening appears to transfer inland.
The ongoing shortening near the Delta front is consistent with the ~5 mm/yr northward motion of the Yukon crustal block being taken up by shortening in this region for the past ~5 m.y., i.e., ~25 km. In one possible model, there may be thrusting of the delta sediment section and some continental crust over the oceanic lithosphere, with the detachment at the top of the oceanic crust. This region has relatively few recorded past earthquakes, lying between the Richardson earthquake concentration located to the south of the Mackenzie Delta, and the off shore oceanic events beneath the Beaufort Sea. As discussed below, it is not clear whether the shortening is a seismic or is accommodated by infrequent large thrust earthquakes. The earthquake behaviour of infrequent large events with few smaller earthquakes does occur elsewhere. For example, several M6.6 to M6.9 earthquakes occurred in the southern Mackenzie Mountains (Nahani series) in 1985 where the previous seismicity was relatively low. An extreme case is the infrequent Cascadia M9 earthquakes on the subduction zone thrust that extends from off Vancouver Island to N. California, where there are few if any small events and giant earthquakes are centuries apart.
There are three possibilities to explain the lack of recorded seismicity in the Mackenzie Delta region; (1) The tectonic model is incorrect and there is little northerly motion of the Yukon block north of 68.5°N. This interpretation seems unlikely in view of the GPS data and the strong seismicity in the Richardson Mountain zone representing strike-slip motion of the Yukon block relative to the Craton. It also is not in agreement with the geological inference that Holocene-Recent deformation at the Delta front represents the accommodation of convergence between the Yukon block and the Beaufort Sea crust. (2) There is the possibility that there is seismic deformation in the Delta region but it is characterized by infrequent large earthquakes with very few small events. Such an earthquake behaviour is not common but as noted above, it does occur elsewhere. (3) There is current deformation in the Delta region but it is aseismic. Elsewhere in the Yukon most deformation appears to be seismic and there is good agreement between the deformation rates inferred from GPS and tectonic models, and that indicated by the rates of seismicity. However, the Delta consists of sediments of relatively low consolidation and high pore pressures so deformation within it could be aseismic. The deformation may extend into the basement beneath the Delta where the crystalline rocks might be expected to be seismic. However, at that depth the temperatures could be too high for seismicity which is normally limited to about 350°C, i.e., the seismic “brittle-ductile” transition. We favour the last explanation, that deformation is aseismic, but the possibility of infrequent large earthquakes in the Mackenzie Delta region cannot be excluded with the available information. The low current seismicity should not necessarily be interpreted to mean low probability of future large earthquakes.
If the GPS-defined northerly crustal motion of the Yukon is taken up in the region of the Mackenzie Delta front, the shortening rate may be similar to that of the seismically active Mackenzie Mountains to the south. Therefore, if the shortening is seismic, the earthquake hazard may be similar. The maximum magnitude of thrust events in this area may be estimated from the maximum fault length (e.g., Wells and Coppersmith, 1994), to be about M=7.5. A 5 mm/yr northerly motion can be accommodated in the thrust region by M=7 events at an average interval of a few hundred years. If the motion is seismic, a similar rate for large strike-slip earthquakes also is estimated for the eastern portion of the Delta (Eskimo Lakes fault zone region) which may be the northern extension of the Richardson Mountains strike-slip boundary.
Rates of Seismicity and Seismic Hazard
Most estimates of seismic hazard assume well-behaved statistics with the number of earthquakes decreasing systematically with increasing magnitude. An example is given in Figure 5 of the Gutenburg-Richter relation for the frequency of occurrence of earthquakes as a function of magnitude. The best fit line assume a Poissonian statistics. The uncertainties increase with increasing magnitude because of the fewer events in the catalogue at large magnitudes. The relation, based mainly on the small to intermediate magnitude events, estimates the frequency of the larger earthquakes.
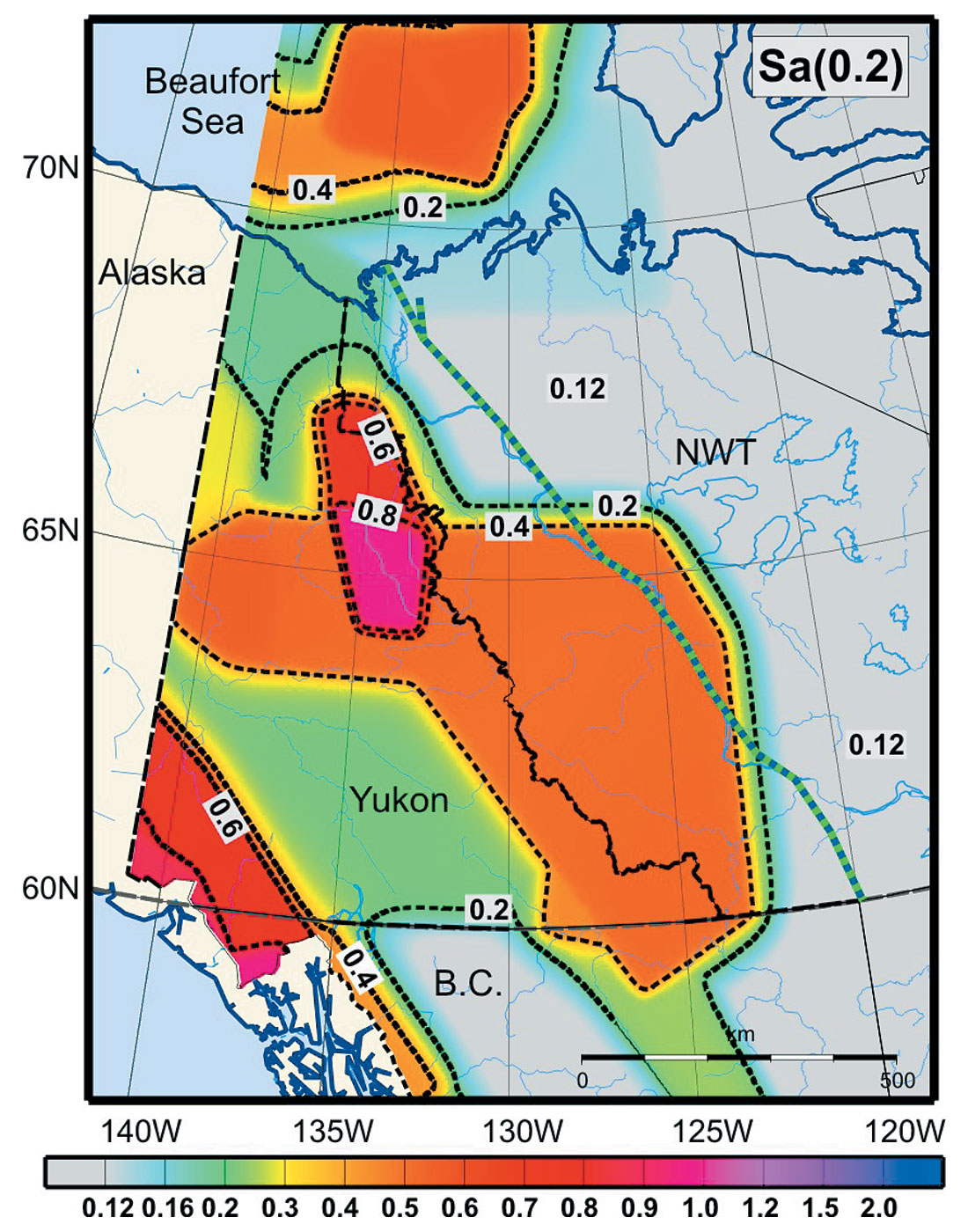
The expected locations and rates of earthquakes and the shaking they will generate are used to quantify seismic hazard. The 2005 National Building Code will be based on 5% damped spectral acceleration at a probability of 2% in 50 years (Figure 6) (Adams and Atkinson, 2003). These hazard descriptions are intended for a specific quantitative engineering design application, but in simple interpretation the contours can be used to differentiate the areas of high and low hazard. The seismic hazard model (Adams and Halchuk, 2003) uses two alternative distributions for the earthquakes, but the high hazard areas of Mackenzie-Beaufort are dominated by the three active source zones shown on Figure 5, with the relative levels reflecting their frequency of earthquake occurrence. Maps like Figure 6 might under-estimate the t rue hazard if large earthquakes in the Mackenzie Delta region are both infrequent and occur without recent small earthquakes. Hence our preceding discussion indicates a continuing need to relate new GPS deformation, recent geological deformation and seismicity information in order to improve current hazard estimates.
Delta Sediments, Permafrost and Ground Shaking
There are several limitations to the quantitative earthquake hazard as in Figure 6, in addition to the limited earthquake history data base, as discussed by Adams and Atkinson (2003): (1) the amplitudes of ground motion for a given sized earthquake as a function of distance (attenuation relation) is taken from relations developed using earthquakes from much further to the south. (2) The ground motion probabilities are given for “firm soil”. For other conditions, generic adjustment factors are used in the 2005 Building Code, but site-specific values may differ considerably. Ground motions may be substantially modified by soil conditions, especially the low-consolidation sediments of the Mackenzie Delta and Mackenzie River valley. For strong shaking on similar deep soft soil deposits elsewhere, high frequencies are usually somewhat attenuated but low frequencies are substantially increased in amplitude. (3) the effect of varying thicknesses of permafrost on earthquake ground shaking has not been evaluated.
In addition, in some areas earthquake triggered landslides, slope failures, and liquifaction may be important secondary effects. Also, if large events can occur beneath the Mackenzie delta front, tsunamis also are a possible hazard either due to the displacement of the seafloor or more likely by earthquake-triggered submarine slumps.
Conclusions
There is exceptionally strong seismicity in southwestern Yukon and adjacent Alaska and British Columbia, and the earthquake hazard is high in that region of the Alaska highway. There is lower seismicity in central Yukon, but increasing toward Alaska. On the eastern Cordillera mountain front, the seismicity and hazard are again high in the areas of the Mackenzie Mountains, Richardson Mountains, and also beneath the Beaufort Sea. The immediate area of the Mackenzie Delta has low historical seismicity, although significant ground motion can be expected from earthquakes to the north and south. In addition, there is a possibility that the Delta region has large earthquakes with few small events. The large earthquakes may be too infrequent to have occurred in the available instrumental record. It is important to recognize that the ground shaking from earthquakes may be substantially modified from the effect of unconsolidated delta or river sediments, and by permafrost. There also may be important secondary effects of earthquakes triggering landslides, slope failures, sediment liquifaction, and tsunamis.
Acknowledgements
We acknowledge contributions over many years of the seismic analysts and seismologists that have generated the high quality Canadian earthquake catalogue and other earthquake information which is the basis of most of our earthquake hazard information. Steven Halchuk provided the data for the earthquake recurrence figure and seismic hazard map. John Ristau provided information on the large earthquakes, and Lucinda Leonard assisted with earthquake maps. Publication of the Geological Survey of Canada.
Join the Conversation
Interested in starting, or contributing to a conversation about an article or issue of the RECORDER? Join our CSEG LinkedIn Group.
Share This Article