Introduction
This four part series focuses on recent improvements of the two most effective potential field methods used in hydrocarbon exploration, namely gravity and magnetics. Both of these methods have been used extensively over decades by the oil industry, but with a varying degree of success. A successful survey was almost always the result of the proper application of gravity and/or magnetics, hand in hand with realistic expectations of results. "Overselling" of the methods as well as unrealistic expectations on the part of some users discredited both approaches in an unjustified manner. The potential for dissatisfaction with gravity and magnetics results was often also the result of bad survey parameterization and poor data acquisition without competent quality control, as well as "run of the mill" processing, analysis and interpretation.
Data resolution, often confused with and advertised as data accuracy, reached its useful limit for exploration purposes well over a decade ago. However, significant technological improvements have been made over the past five years which allow for much better data accuracy in gravity and magnetics data acquisition. These improvements as they pertain to aeromagnetic surveys will be described in this part of the review.
Early Techniques
The earliest aeromagnetic surveys for hydrocarbon exploration were carried out with Fluxgate (Gulf) magnetometers in the early 1950's. The resolution and accuracy of these instruments generally did not exceed 5 nT (gamma). Data were recorded in analogue form. Data quality by today's standards was poor, especially for surveys in lower magnetic latitudes, but good enough to entice most multi-national oil companies into using the approach and obtaining basin delineation information. One of the chief problems for the early aeromag surveys was navigation and data positioning, both of which relied nearly one hundred percent on visual observations only.
The Proton Precession Magnetometer
In the 1960's two major improvements were made in aeromagnetic surveying. The first proton precession magnetometers were introduced and navigation was supported by simple Doppler systems. Magnetic data resolution improved eventually to the one gamma level and data accuracy increased slowly due to significantly better data positioning. Still, all data were recorded in analogue form until the late 1960's. The majority of the Western Canadian Basin was covered by this and the above described type of data acquisition. Amazingly enough, maps from these surveys are still being marketed.
Proton precession magnetometers were improved a great deal in the 1970's. Achieving a resolution of 0.1 gamma was no problem with IC based electronics. As well, navigation and data positioning went through major upgrades from Doppler to INS and other electronic or electromagnetic short and long range navigation systems. Equally important were advancements made at the same time on the data recording side. With digital recording available, accurate geophysical and positioning data recording made for generally quite good and reliable survey products. The majority of aeromagnetic coverage world wide is of this calibre of data quality.
It also needs to be pointed out that 'towed bird' proton precession sensors often were noise prone, even if it was never quite obvious on good systems. An appropriate tow cable length linked elastically to a stable bird design could almost always assure that the aircraft magnetic influence was nearly eliminated. However, towed bird sensor configurations were greatly influenced by air speed variation and cross winds which produced major shifts in position relative to the aircraft. These changes often were responsible for an intermediate to low frequency noise envelope of several gamma.
Most other forms of magnetic data acquisition (ground and marine applications) standardly use proton precession magnetometers. The quality of the instrumentation is well in line with data quality requirements. More surprising is that some aeromag contractors still use proton precession magnetometers as ground station monitors.
Optically Pumped Magnetometers
"Optically pumped" magnetometers were first used in the early 1970' s, just when major improvements were made on proton precession magnetometer systems. Technical installation complications however prevented ready acceptance of optically pumped magnetometers by the industry for almost a decade. Optically pumped magnetometers reliably provide a resolution and accuracy (in controlled circumstances) of 0.001 nT, thus far exceeding the performance of any proton precession magnetometer. The major draw-back of optically pumped magnetometers is that the sensor element is basically uni-directional, which means that any change in sensor alignment to the earth magnetic field produces a magnetic field measurement change. In contrast, proton precession magnetometer sensors are nearly omni-directional and thus quite immune to sensor orientation changes. It is for this reason that optically pumped sensors can not be used in a 'towed bird' configuration. Optically pumped sensors need to be fix mounted on the aircraft, generally in a tail "stinger" installation, to remove the sensor from "aircraft noise" as far as possible.
Data Noise Reduction
All survey aircraft contain a significant amount of magnetic material (structural and engine components, steel control cables etc.), which in flight are not stationary in relation to the sensor because of aircraft control functions and flex. The problem is further compounded by the proximity of the sensor to the aircraft engine(s), which can generate a significant and potentially quite variable noise envelope. Modifications to the survey aircraft as well as a complex compensation system are required to eliminate or at least greatly attenuate all these noise sources and to minimize the directionality of the sensor, which is amplified by the static and dynamic magnetic components of the aircraft itself. Looking at these problems in detail, it is not surprising that only more recently, low noise magnetic survey aircraft with very low "bias" changes have become available.
Sensor Compensation
From the perspective of magnetic data quality, the most important progress in aeromagnetic data acquisition in the last few years was made in the improvement of magnetic sensor compensation. Computer controlled compensation systems allow for the elimination of the "static" magnetic aircraft components and the minimization of dynamic magnetic changes. Thus the total intensity of the magnetic field is sensed nearly equal in absolute terms, independent of heading and normal aircraft attitude changes. The quality of the system compensation is standardly expressed in the Figure of Merit (FOM) for a survey aircraft. The FOM sums up the experimentally determined sensor bias over a very low gradient magnetic area for at least four different headings with +/-5 degree changes in pitch, roll and yaw, with all ancillary survey instrumentation on board in operation. Although the FOM is measured in absolute magnetic units, it is a relative measure, as it is dependent on the total intensity of the magnetic field in which the tests are performed. An FOM of 1.2 gamma in central Alberta is good, while the same figure would be rather poor in western Brazil, where the total intensity of the magnetic field is only 23,000 nT. FOM figures have improved from a realistic 3 to 5 gamma and worse five years ago to just about the one gamma level now for good survey aircraft. The FOM represents however only one component of the eventual quality of a survey. Several other very important factors are integral to data acquisition quality, and need to be considered very closely in the parameterization and specifications.
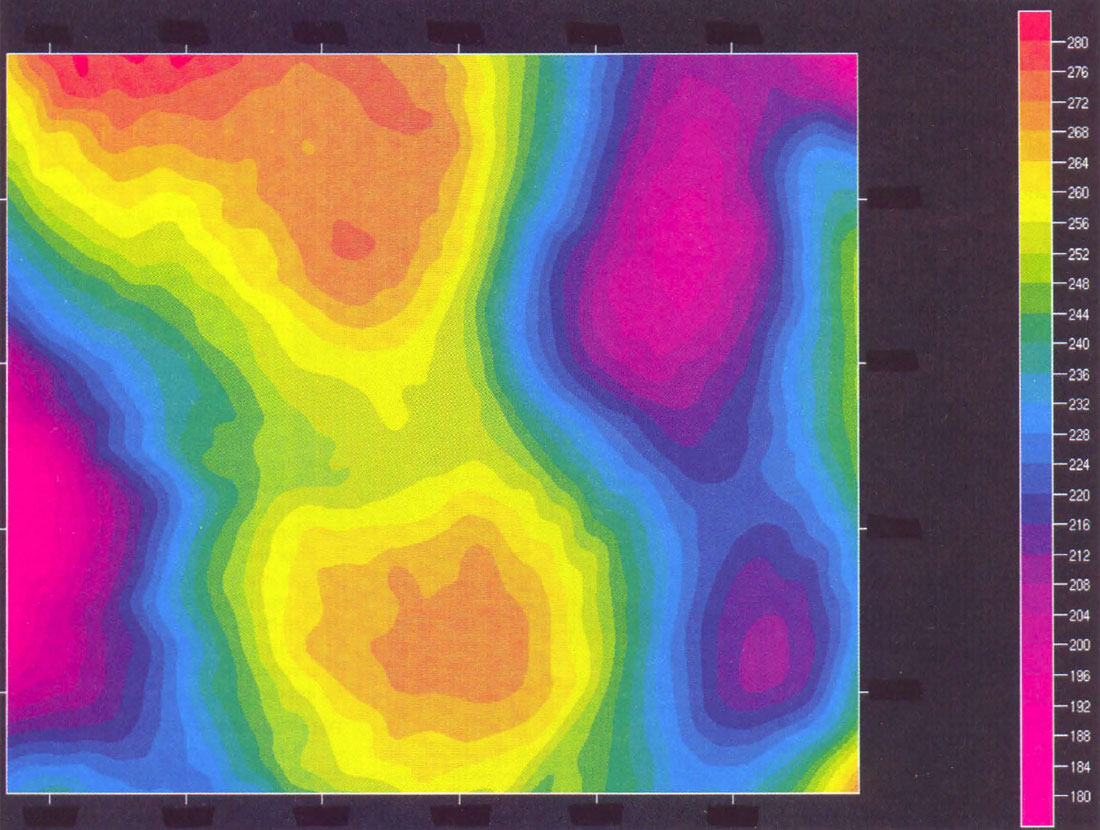
Diurnal Recording
The total intensity of the earth magnetic field changes continuously. These changes can be minimal for hours but also occur at a rate of several hundred gamma in just one minute, depending on sun spot activity. These so-called diurnal variations are of course of major concern in data acquisition. The variable frequency-amplitude product induced by the diurnal needs to be avoided or at least minimized in data acquisition if the survey data are to represent the true and (constant) induced magnetic geological response. Allowable diurnal activity levels need to be specified sensibly for each survey and the magnetic diurnal variation must be recorded time synchronized with any magnetic data acquisition. As the final product is only as good as the weakest link in the contributing chain, the ground station magnetometer should also match (the performance) of the airborne system. The ground station system is to be set up properly in a magnetically constant and noise free environment inside the actual survey area to record the diurnal amplitude/frequency changes encountered by the airborne sensor.
Navigation and Positioning
Good navigation and data positioning has been one of the biggest problems in aeromagnetic data acquisition from the beginning. The quality of visual navigation depended a lot on the individual pilot and navigator and was greatly influenced by the variety of identifiable features on the surface. Data positioning was usually based on continuous strip or frame camera film, which allowed for more or less accurate "picks" only at random intervals. Actual data positioning resulted mostly in pro-rated interpolation of the magnetic data between identified points. This approach resulted in considerable wave form distortion which often caused serious mismatches from profile to profile and at intersections with control lines. These mismatches were then "levelled" in data processing. One can easily see that many minor but important features in a magnetic data set were obliterated by this adjustment and levelling process.
Aeromagnetic data acquisition was always one of the first civilian industries to apply technology usually developed for military purposes. First it was Doppler, which helped actually very little in navigation, but considerably in data positioning. Then came INS (inertial navigation system, which, by the way, navigate cruise missiles). INS allowed for improvements in navigation as well as in data positioning. It should be known however that both Doppler and INS are subject to considerable drift. They are relative positioning systems which require frequent "updates" to known positions in flight. A number of other low and high grade navigation and positioning systems such as Omega (VLF), Shoran, Loran and "Mini-Ranger" were used over the years, but none of these systems was satisfactory either because of range or accuracy limitations.
The advent of GPS (satellite based global positioning system) provided for the solution the aeromagnetic data acquisition industry was waiting for. Even at the earliest stage, when there were only five satellites operational, the system was made use of with navigation and data positioning accuracies never achieved before. Currently, all aeromagnetic data acquisition is carried out with GPS. The present number of GPS satellites in place along with major improvements made in GPS receiver and processing technology allow for very accurate navigation and data positioning world wide. For increased absolute accuracy, post flight differential GPS corrections are being applied in much the same way as diurnal magnetic data corrections are done to eliminate satellite position and signal transmission errors. Where feasible, real time differential GPS navigation has been used more recently, and both approaches are nearing the sub-meter accuracy level in X,Y and Z at a position update rate of III 0 second, the usual aeromagnetic sampling rate. The GPS time base is also used to trigger simultaneous recording of the magnetic data sample, the aircraft GPS position, the stationary, ground based GPS position for differential corrections, as well as the diurnal magnetic recording.
Ancillary Equipment
Necessary ancillary instrumentation for aeromag surveys, such as radar and barometric altimeters and the former "positioning" camera, need to be mentioned briefly. The barometric altimeter is/was used to record the elevation of the aircraft above sea level. It has become basically obsolete as the GPS Z-component is much more reliable and not influenced by atmospheric pressure gradients and inherent instrument drift. Radar altimeters have not much improved over the years, but are generally sufficient for the purposes required in aeromagnetic surveys, as long as they are mounted properly on the aircraft to offset the average pitch held by the aircraft during data acquisition. A real improvement is provided by good video cameras replacing the old positioning cameras and the cumbersome rolls of film. As far as positioning is concerned, the video is only used for spot checks. Nevertheless, the video is not obsolete, as its use and emphasis have shifted to helping in the identification of cultural and other surface anomalies which need to be edited out in the preparation of the final data set of an aeromag survey.
Finally, constantly increasing portable computer power and data display facilities are being made use of in on board installations and provide also for very quick and high volume turn around in data quality control on site at the data acquisition base. This allows for nearly immediate and comprehensive feed back concerning any data acquisition quality problems. Given competent personnel, a reasonably good system will facilitate the preparation of the final survey data set on site very shortly after conclusion of the survey, a step which took weeks if not months of office work just a few years back.
Conclusions
To take full advantage of the technical improvements made over the past five years, it is essential that aeromagnetic surveys are parameterized properly and executed strictly within the given parameters. Good survey parameterization goes far beyond the basics of line spacing, direction and survey altitude. Details about parameterization will be addressed in the fourth article of this series under Project Management.
Proper survey parameters, entrenched in the survey contract specifications and enforced by competent quality control can ensure that the data acquisition truly benefits from all technical improvements. Experience has shown that the data set accuracy will easily improve by a factor of 5 to 20 or more over earlier surveys. This accuracy increase provides not only for much more dependable detail in magnetic basement analysis, but even more importantly, it allows for the reliable resolution of weak, but indicative intra-sedimentary magnetic anomalies, which was previously not possible.
Acknowledgements
Thanks to Brian Henry for his review and editing of this article.
Join the Conversation
Interested in starting, or contributing to a conversation about an article or issue of the RECORDER? Join our CSEG LinkedIn Group.
Share This Article