Abstract
Seismic reflection events are typically catalogued as primary or multiple depending on whether the arriving energy at the receiver has in its history experienced one or more upward reflections, respectively. We can trace the evolution of progress and effectiveness in seismic processing by following the physics used to describe what the wave experiences between source and receiver. More realism and completeness in the physics, when applied appropriately, has an associated improvement in prediction and reduction in risk. Hence, there is alignment between providing step-change improvement in seismic capability and a central need of the petroleum industry.
We are classifying three types of step-changes in seismic processing that occur when:
- the dimension of the physics moves up-1D to 2D, 2D to “3D”, “3D” to 3D to match the dimension of subsurface variation, (“3D” here refers to current typical 3D acquisition); two other step-change types occur within a given dimension when;
- the theory, and algorithms, for propagation and reflection are expanded to accommodate further significant, prioritized and relevant phenomena, (e.g., including rapid lateral heterogeneous velocity variations and curved, dipping, and diffractive reflectors), and;
- the language and associated mathematics for describing the experiences and history of the recorded wave-field allows for coding and decoding (i.e., processing), or raveling and unraveling, in terms of approximate achievable rather than inaccessible precise subsurface information.
It is much simpler to provide a precise description of an event in terms of accurate rather than approximate subsurface information and the former is the assumption behind Stages (1), (2) and (3) of seismic processing evolution (see Table below). The problem is that if the forward description is e.g., in terms of actual velocity, the reverse or processing of those events within that picture requires actual velocity, as well. While the precise description of events in terms of approximate information is more complicated, and, hence, the processing algorithms more computer intensive, the benefits can be not only cost-effective, but essential and indicated for effective processing- especially under complex geologic conditions. The latter description currently provides the conceptual framework behind the prediction and subtraction of free-surface and internal multiples with only reflection data and no subsurface information whatsoever; and, in addition, the promise and conceptual possibility for providing accurate processing objectives from primaries, that is, accurate imaging in space (depth) and inversion for earth properties - directly in terms of approximate achievable rather than ideal inaccessible subsurface information.
The first two step-change types listed above lead to Stages 1,2 and 3 in the Table. Step-change type 3 leads to Stage 4 in the evolution of seismic processing.
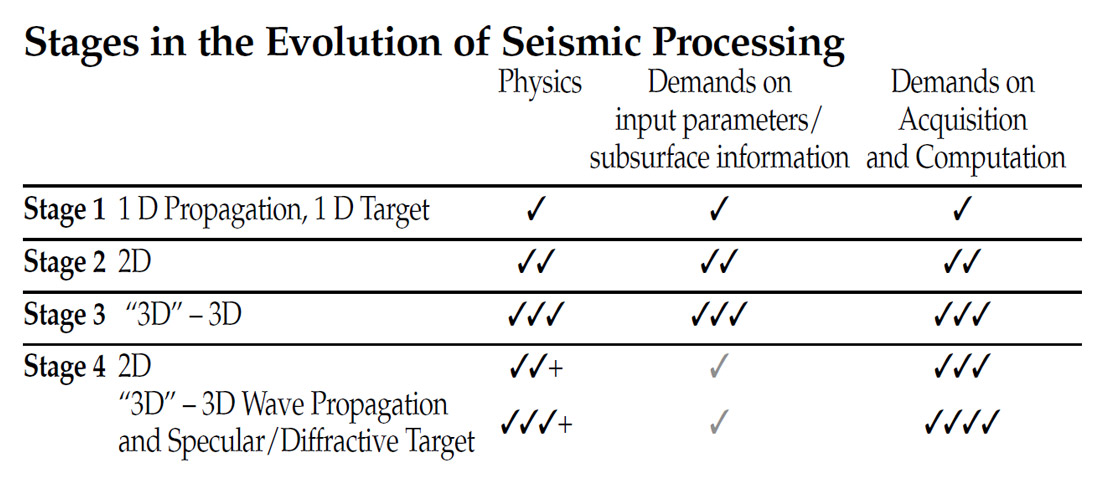
The first three evolutionary stages represent progress in dimension, and/or physics model and predictability and have a concomitant increased demand on subsurface information, and completeness of acquisition and computer time and cost. For example, the modeling and subtraction of 2D ocean bottom multiples is both more accurate (for a 2D water bottom) and demanding of ocean bottom information and computer time, than a 1D counterpart. Moving from time migration to depth migration (within a given dimension) is both more accurate and more demanding of an accurate velocity model and computer power.
The requirements on adequate velocity information to achieve adequate imaging can be an unattainable demand under complex geologic conditions, e.g., sub-salt, sub-basalt and sub-karsted sediments, and has caused our current leading-edge imaging techniques, which are all Stage 3 on this evolutionary scale, to often produce sub-optimal, unacceptable or misleading results.
Stage 4 in evolution requires the language jump and associated processing for primaries and multiples described in step-change (3). The promise of Stage 4 in the evolution of processing is to relax the demand on subsurface information for processing multiples/ primaries, especially under complex geologic conditions, without lowering our processing objectives, broaden the 3D physics of propagation and reflection, but at increased demands on the definition, (e.g., the source signature) and completeness of acquisition, and higher computer costs. The practical significance of the step to Stage 4 processing is hard to exaggerate. It represents an empowerment, where those willing to pay more (for acquisition and compute time) have the opportunity to achieve more. In contrast, if you have a Stage 1, 1D demultiple algorithm, no amount of money and increased acquisition will allow it to accommodate a diffraction. Similarly, if your velocity model is inadequate-money and acquisition won’t impress a Stage 3 imaging algorithm to cooperate or to be sympathetic to your dilemma of finding an accurate image in space. The transition from Stage 3 to Stage 4 represents a shift in responsibility for providing detailed subsurface information upwards to the surface of the earth where acquisition and complex algorithms with higher computer costs allow intelligence and money to directly impact effectiveness. Computers get faster and cheaper. Furthermore, the added costs pale in comparison to the economic risks, potential benefit and technical challenges, e.g., in deep-water E&P.
The attenuation of free surface and internal multiples are at Stage 4 in evolution; the current leading -edge imaging and inversion algorithms for primaries are at Stage 3. The next big step is to bring imaging and inversion to Stage 4,and thereby raise overall seismic empowerment. We know that bringing multiples to Stage 4 wasn’t easy, and considerable conceptual and practical hurdles were faced and overcome; we anticipate that in bringing primaries to Stage 4 we will face higher hurdles yet, but the research efforts are underway, and enthusiasm, courage, and the sense of adventure are on our side.
Join the Conversation
Interested in starting, or contributing to a conversation about an article or issue of the RECORDER? Join our CSEG LinkedIn Group.
Share This Article