In the fall of 2012 we conducted a field test of a trenched fibre optic Distributed Acoustic Sensing (DAS) system to trenched and surface 3C geophones in the Daly area of southwestern Manitoba, Canada. The test was designed to provide a comparison of the acoustic wavefield as measured by the trenched fibre, the trenched 3C geophones and surface 3C geophones and also to look at the full elastic wavefield (PP and PS). And as such, various azimuths were recorded using all sensors. The geophone data is of exceptional quality as is normal for this area. The fibre (DAS) data show clear reflection, refraction and coherent noise signal but at a lower signal-to-noise ratio than the geophone data. It was also noted that for our acquisition geometry, it was difficult to separate the different modes based on particle motion and so we had to rely on PP and PS velocities for this. Furthermore, we were not able to separate the radial and transverse PS modes on the fibre data.
Distributed Acoustic Sensing (DAS) using fibre optic cables is an emerging technology that has many applications in the energy, security and environmental sectors (Figure 1).
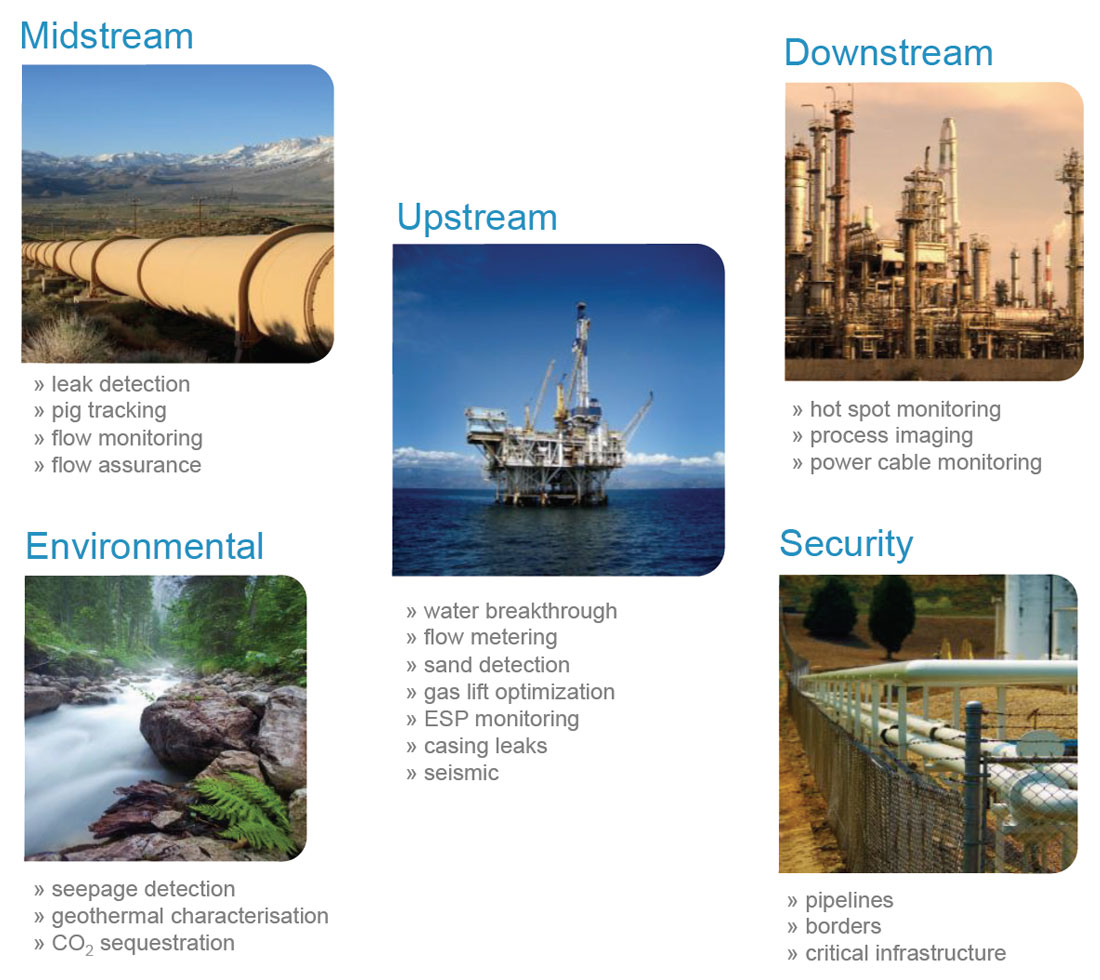
We are interested in using DAS for the measurement of ground motion in a land surface seismic environment using fibre optic cables.
Historically, discrete fibre optic sensors have relied on a Bragg diffraction grating (Bostick, 2000; Hornby et al., 2005) and typically in the borehole for flow monitoring, temperature measurements and VSPs. Recent advances have shown that the fibre cable itself can act as a sensor (Daley et al., 2013; Mestayer et al. 2011) thus potentially reducing cost and increasing spatial sampling.
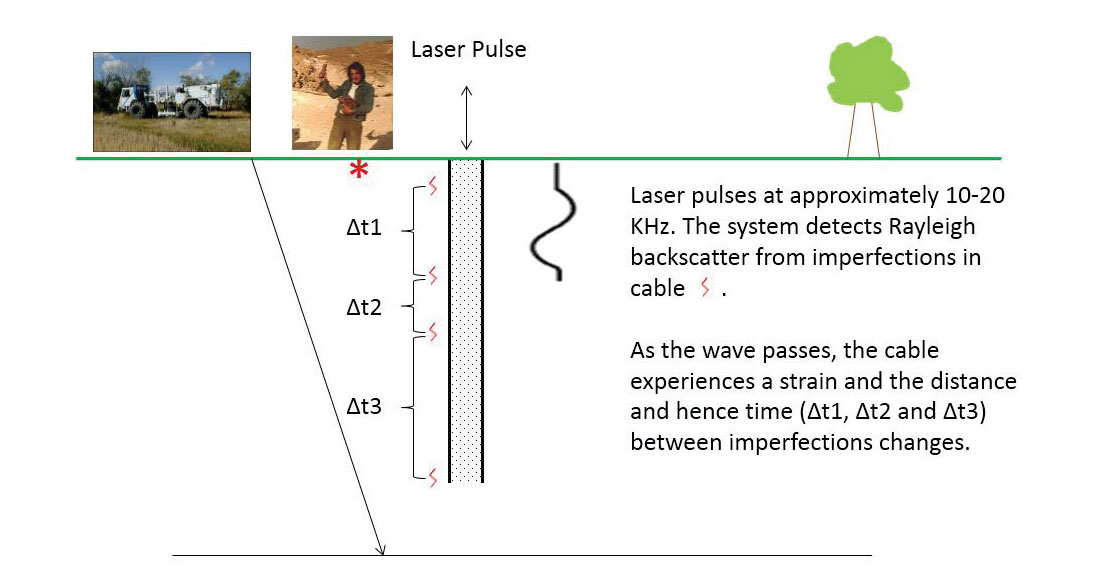
It has been shown in lab settings that DAS will detect and replicate various acoustic signals from different segments of the cable without optical crosstalk or interference of any sort (Farhadiroushan, personal demonstration). Daley et al., 2013 describe Rayleigh backscattering in a continuously backscattering sense such that the light “echo’s” can be sensed. As the fibre experiences different strains, the backscattered light properties are changed. These changes are related to stress, strain and surface displacement (Figure 2).
Field Test
In October, 2012 we embarked on a field test near Daly, Manitoba, Canada that was intended to compare a trenched fibre optic Distributed Acoustic Sensing (DAS) system to trenched and surface 3C geophones. The test was embedded in a conventional 3D-3C surface seismic program. The receiver layout is shown in Figure 3. Three lines were trenched (N-S, NE-SW and E-W) and the fibre optic cables were laid in the bottom of the trench along with 3C geophones at a 5m interval. The 3C geophones were drilled in to the bottom of the trench as with the surface 3C geophones and the orientation was measured. The trench was then backfilled and compacted using a Bobcat. The 3C surface geophones were deployed 1m adjacent to the trench also at a 5m interval. The receiver lines were 320m long. Therefore, we had 65 3C geophones in the trench and on the surface for each receiver line (Figure 5 and 6).
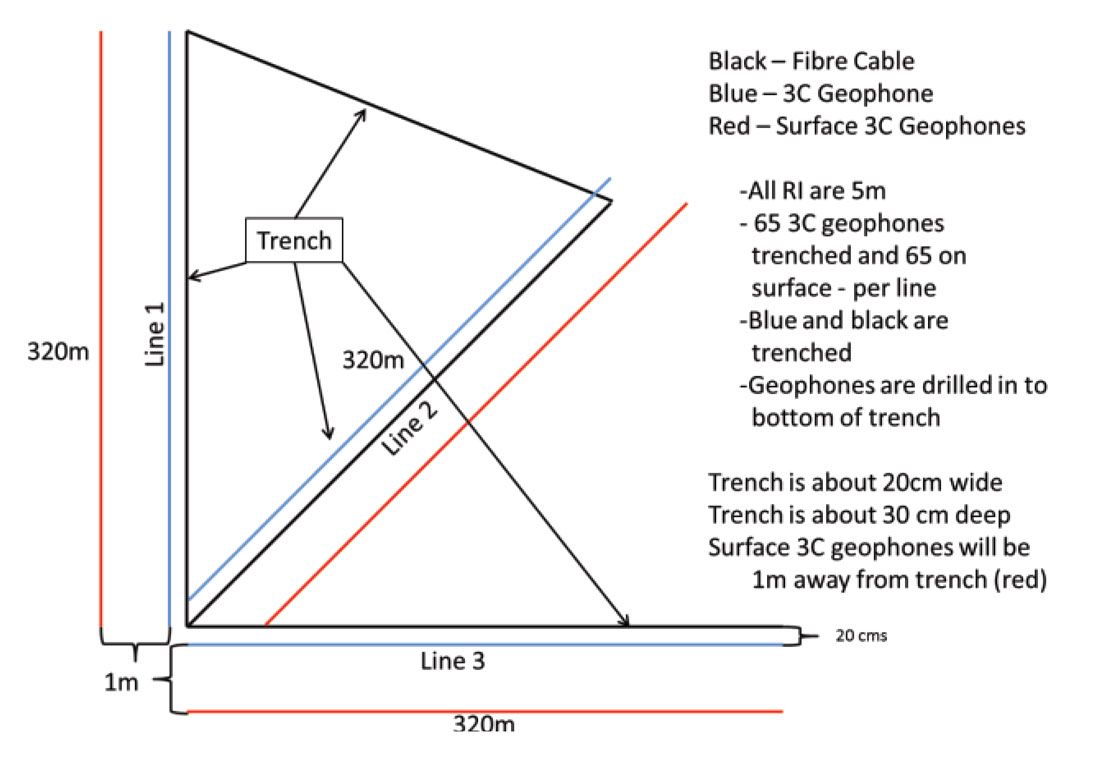
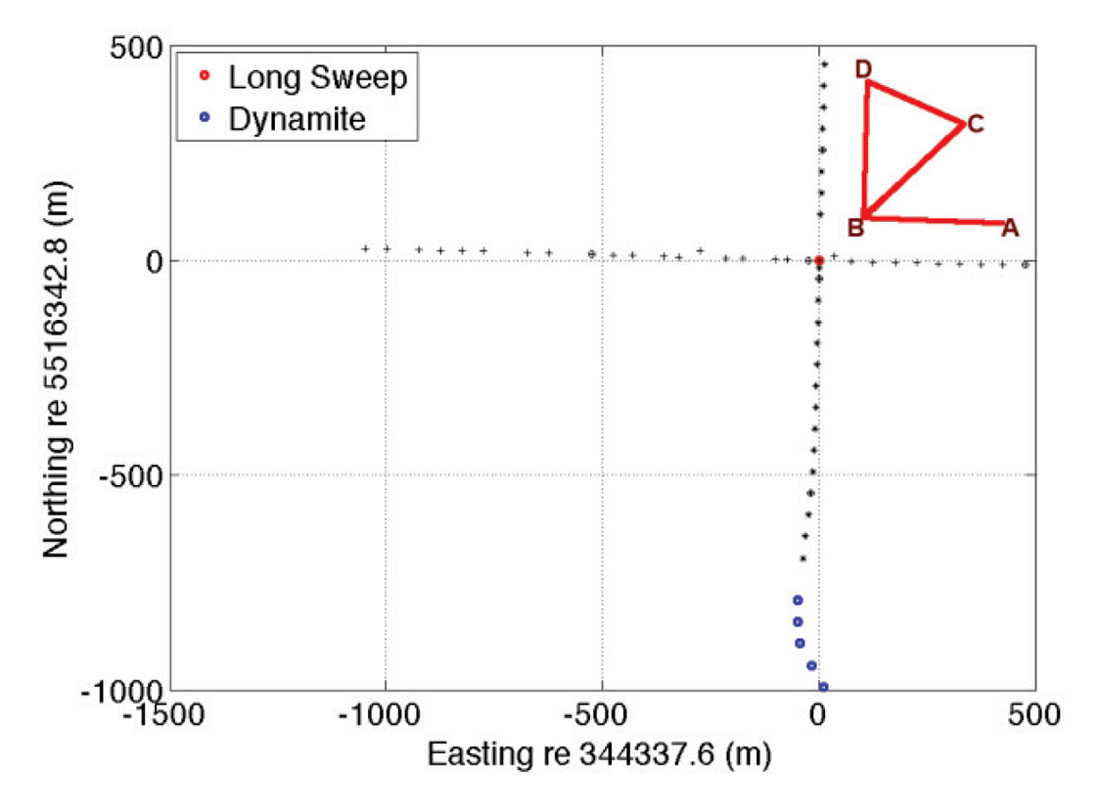
The source layout is shown on Figure 4. We tested various source types including dynamite (0.5 kg at 12m and 1.5 kg at 12m), long sweep vibroseis (2 vibes by 8 sweeps for 50 sec. over 10-250 Hz) and the production sweep (2 vibes by 8 sweeps for 12 sec. over 10-250 Hz).
The entire test was laid out and acquired over two days after the crops were off during non-frozen conditions.
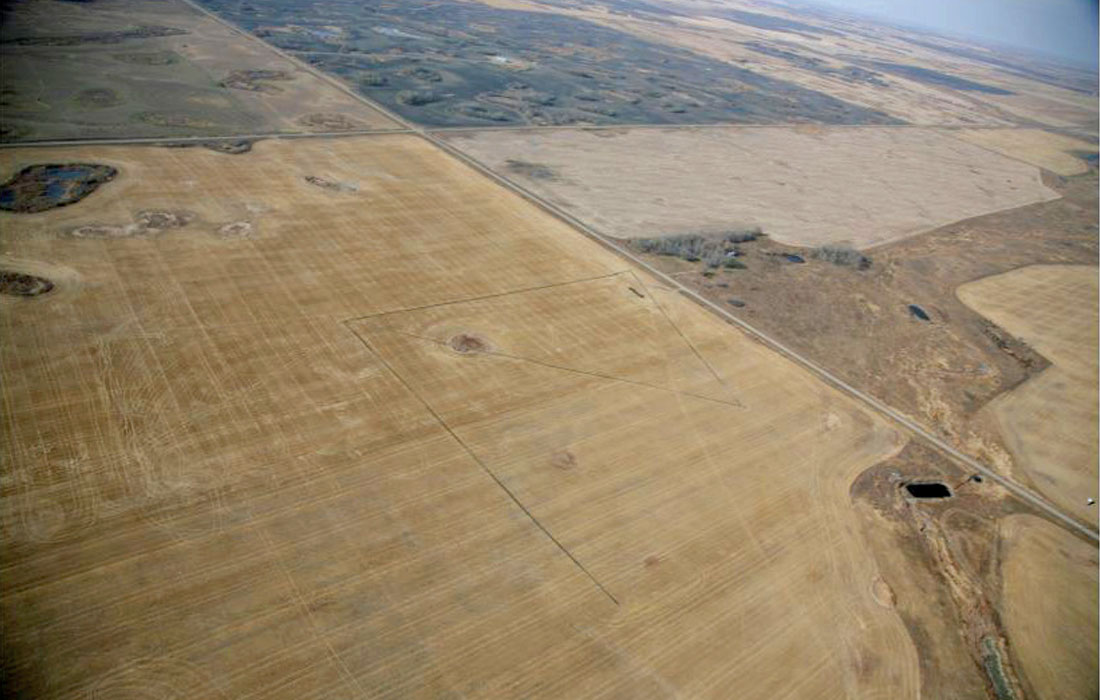
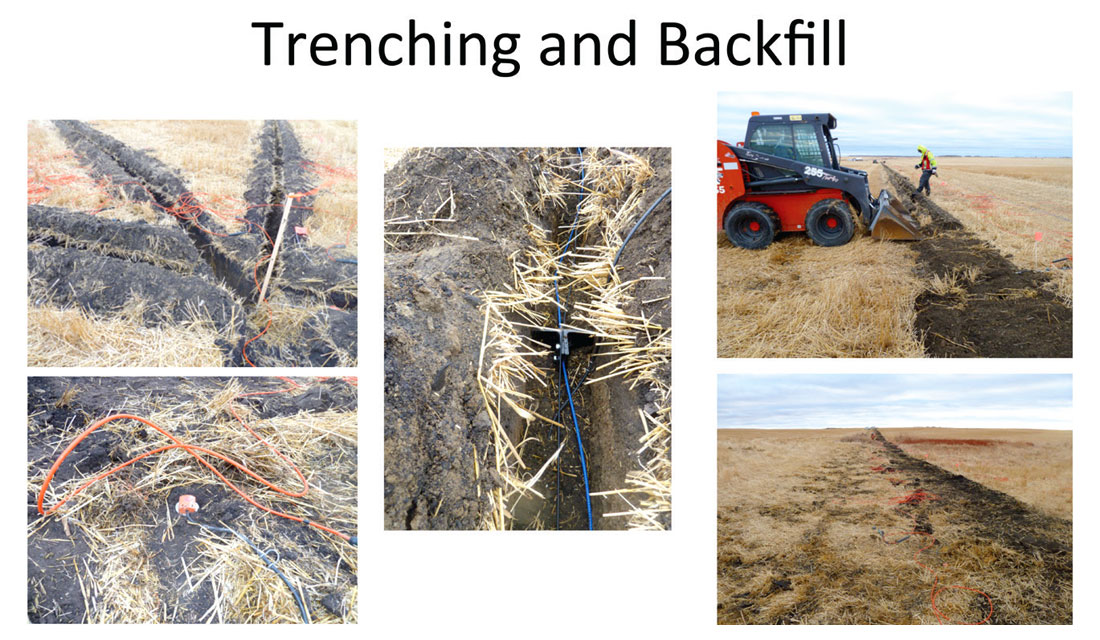
Results
Figure 7 shows a single shot record (production vibe source) from the N-S line with the source about a third of the way down the receiver line. The first break energy and the groundroll energy are clearly visible on both the fibre (left) and vertical geophone data (right) but the reflection energy is clearer on the vertical geophone record. Figure 8 shows a Common Offset Stack (COFF) of the fibre data with the various wave modes interpreted as: coherent noise (black); reflected PP signal (yellow) and; reflected PS signal (red). The coherent noise event (black) that goes to the bottom left of the COFF is interesting and believed to be a result of the end-on geometry and perhaps represents a multimode refraction event. It should be noted that the surface sampling of the geophone is at 5m and for the fibre data it is 2m. A pre-processing step takes the continuous optical data and converts it to SEGY formatted data at 2m (Silixa). We then re-gridded the fibre data to 5m to match the geophone data.
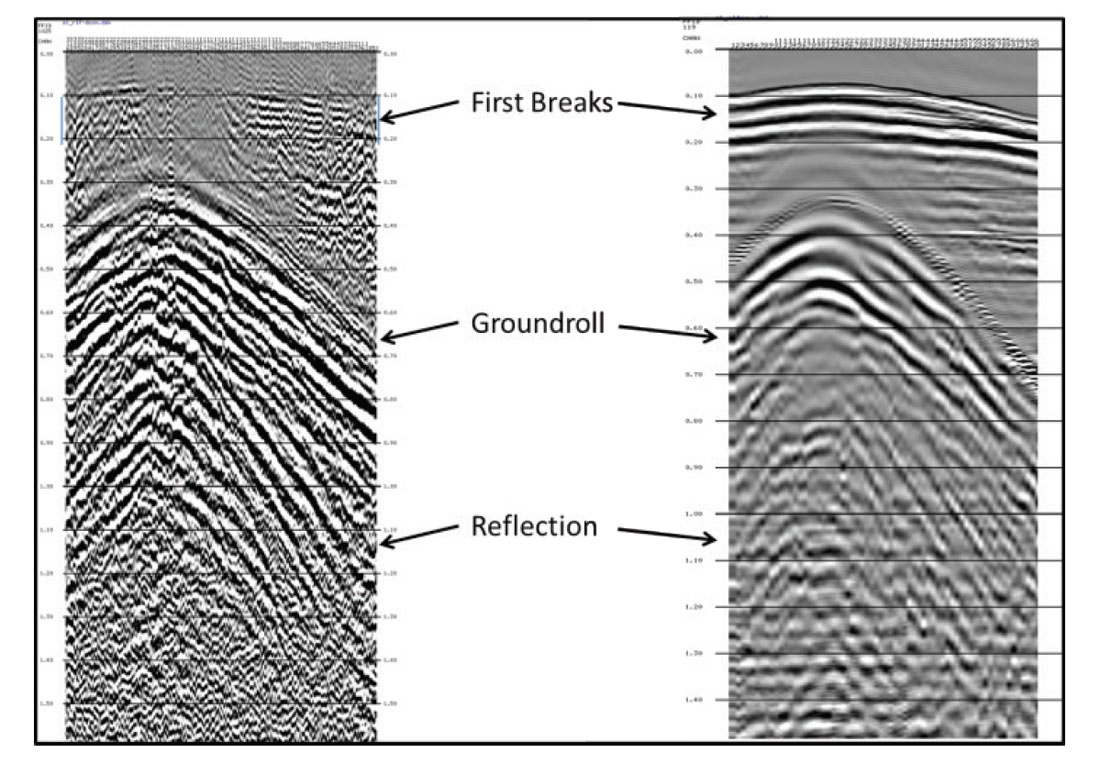
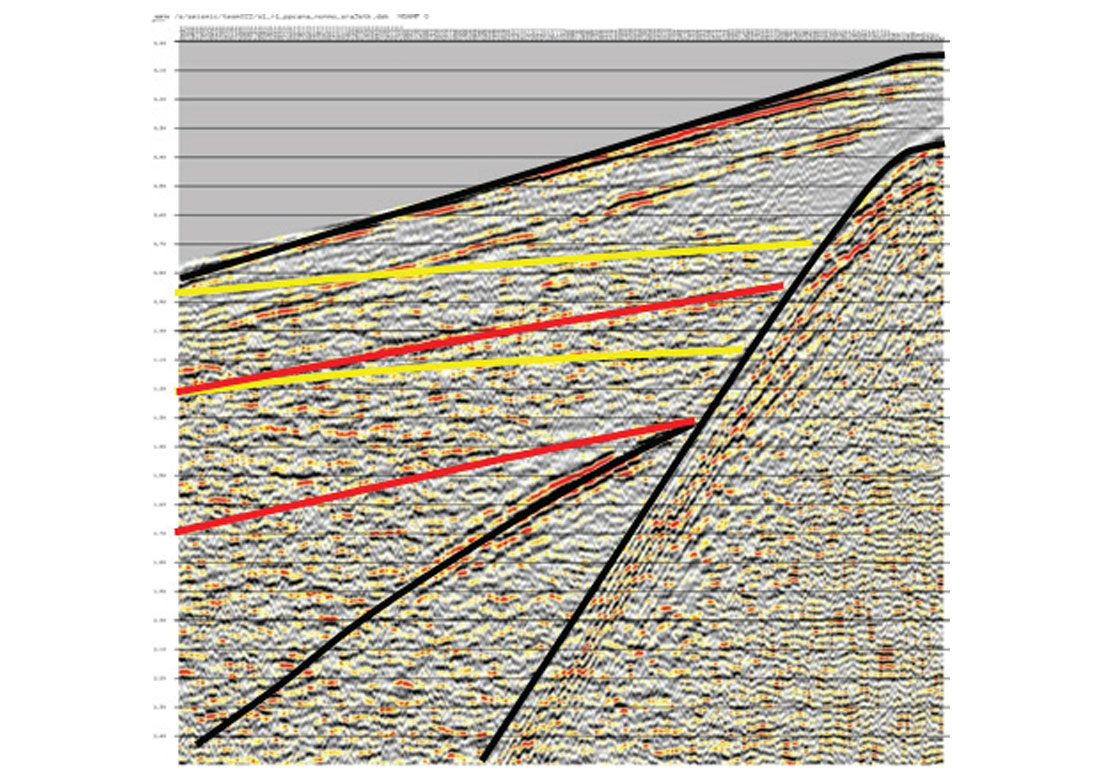
Figure 9 compares the migrated vertical geophone data to the migrated fibre data. While both sections show similarities in reflection energy it is also evident from Figure 8 that there will be modal contamination on the optical fibre data. Since we do not have a way of separating the various wave modes in a particle displacement sense we had to rely solely on the PP and PS event velocities to stack these events. These velocities were derived from the geophone data and applied directly to the fibre data. The same was done with the statics. PS processing was only taken to the brute stack stage as there was insufficient PS signal to do effective noise attenuation. Also, as mentioned previously, we were not able to rotate to the radial and transverse components system or to separate the different PS modes.
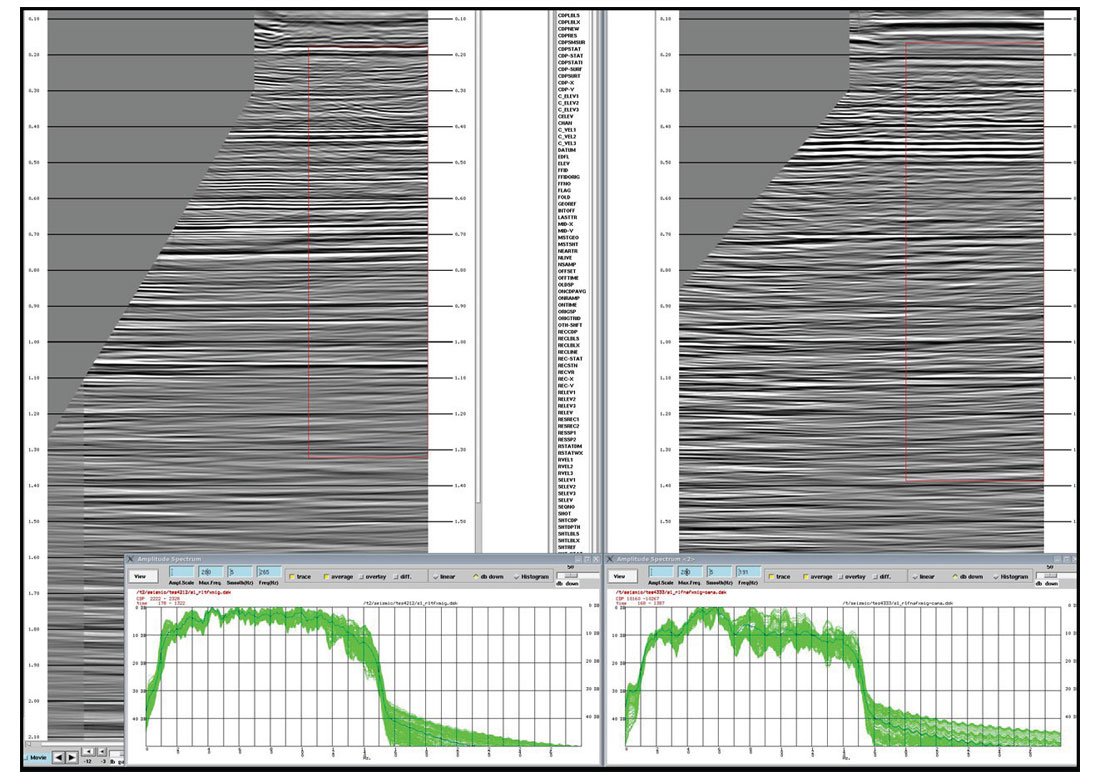
Conclusions
Distributed Acoustic Sensing (DAS) using fibre optic cables is an emerging technology that has many applications in the energy, security and industrial sectors. We have shown that using fibre optic DAS recording systems we can record signal that is generated from active seismic sources much as a geophone does. We were able to produce PP and PS seismic sections using the fibre data with the velocities and statics that were derived from the geophone data. In particular, the PP fibre and geophone sections are comparable yet not identical. One reason for this is that we are not able to differentiate the various elastic modes as measured by the DAS system. So the “P-wave” fibre section has modal contamination (e.g. PS-wave) that is interfering with the pure mode PP data. The second issue is one of signal-to-noise (S/N) on the DAS data. The PS-wave data is most notably affected by the low S/N.
Further testing of acquisition geometries is necessary to enable mode separation based on particle motion and to improve S/N.
Acknowledgements
The author wishes to thank Tesla Exploration Ltd for acquiring the field data. In particular, Dick Madden, Kevin Anton, Richard Vogel and Gary Burke). Silixa Ltd provided the iDAS system and the crew to operate it. Key Seismic for the processing – Blaine Frehlich, Richard Bale and Tobin Marchand. Finally, thanks to an oil company who wishes to remain anonymous for allowing us to embed our test in their 3D program.
Join the Conversation
Interested in starting, or contributing to a conversation about an article or issue of the RECORDER? Join our CSEG LinkedIn Group.
Share This Article