We begin by defining a few key terms: “induced seismicity” refers to a seismic event that is caused by pore pressure and stress change associated with human activity, “potentially induced seismicity” describes an induced seismic event that is coincidental with human activity, “microseismicity” are small scale induced seismic events related directly to the controlled deformation process itself, e.g. -5 ≤ Mw ≤ 0 and “tectonic earthquake” is the violent shaking of the earth’s surface due to a sudden energy release and movement in the earth’s crust which is often associated with natural phenomena. Moreover, in seismology, the term “trigger” refers to the initiation of a process which can cause an earthquake. Triggered earthquakes occur after a time delay that can range from seconds to years after the causative event. We also utilize the moment magnitude symbol, Mw, to refer to the size of the energy released by a seismic event or earthquake and ML to denote local magnitude based on the Richter scale.
We advise the reader that these definitions are provided solely for the purpose of this article and in no way reflect their usage in other disciplines, regions and applications or our personal opinions. These definitions should not be used beyond what is intended in this paper nor used in regular geoscientific practice until their meaning is adopted or defined by the greater scientific community.
Summary
The purpose of this article is to provide an overview of the induced seismicity topic as it relates to hydraulic fracturing in the energy industry. The introduction provides a background of hydraulic fracturing and induced seismicity. Next, we discuss the details and current state of knowledge. Then we review the progress made and resources available and finally identify the various efforts taking place on the topic.
Introduction
Felt seismic events from anthropogenic sources were documented as early as 1894 in Johannesburg (McDonald, 1982) and later “attributed to the Witwatersrand gold production” (McGarr, 2002), which had commenced in 1886 (Cook et al., 1966). Since then, many cases of induced seismicity have been identified and postulated as being associated with underground activities. Some of these examples are shown in Figure 1 and include:
- Mining (e.g. ML 5.3, 2005 Klerksdrop, South Africa)
- Reservoir impoundment (e.g. ML 6.3, 1967 Koyna, India Reservoirs)
- Oil and gas exploitation (e.g. ML 4.4, 2004 Rotenburg, Germany Natural Gas Extraction)
- Geothermal energy extraction (e.g. ML 3.4, 2006 Basel, Switzerland Geothermal energy)
- Nuclear waste disposal repository (e.g. Yucca Mountain nuclear waste site in Nevada)
- Waste water injection (e.g. ML 5.6 2011, Oklahoma, USA Injection of wastewater)
- Carbon capture and storage (CCS), construction sites, enhance oil recovery, groundwater recovery and underground nuclear test sites
Seismicity related to petroleum production became evident in the early 1920’s, to “reservoir impoundment in the late 1930’s”, to high pressure fluid injection in the mid-1960’s and to “natural gas production in the late 1960’s” (McGarr, 2002). Much of the debate in the 21st century has been centered on the possible connection between unconventional oil and gas production activities and induced seismicity. This is especially the case because an increase in occurrences has focused attention on the hazard posed by seismicity induced from injection (ISWG, 2015). With this in mind, monitoring considerations has also become an important topic, especially as operators, and regulators evaluate the best methods in which the risks can be managed and mitigated.
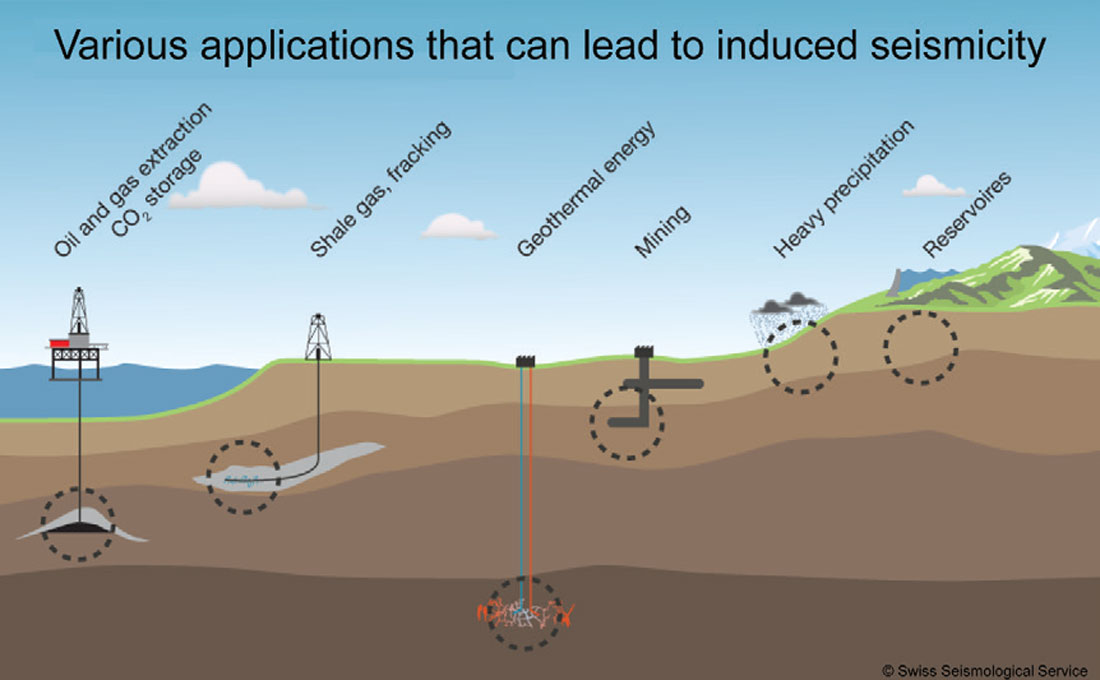
Installations for monitoring mine seismicity began in Europe in the late 1920’s, e.g. Poland’s Upper Silesia coal basin, (Gibowicz and Kijko, 1994). Since then, seismic monitoring systems have been routinely deployed for many purposes both locally and regionally. Regional seismic networks are used widely and continually to locate earthquakes, but mainly to detect tectonic events located in the upper 100m thick crustal layer. Local networks are used for both hazard detection and reservoir development. However, a combination of these two systems can help improve the location accuracy of seismic events and determine the cause of induced, potentially induced and triggered seismicity associated with faults.
We note that natural occurring seismic events happen often and routinely in a number of areas around the world. But, it is difficult to clearly and uniquely differentiate between induced and tectonic earthquakes (ISWG, 2015). Modern seismic imaging methods, as part of geological characterization studies, can be used to identify large faults capable of potentially producing damaging earthquakes but, smaller faults can still go undetected (Zoback, 2012). That is why a combination of site characterization, regional and local seismic monitoring networks is important for reducing the risk to public safety. This is especially important for industry to implement so that they can “manage and continue with operations safely” (Hendrick, 2013).
Hydraulic fracturing is a stimulation tool that requires large volumes of fluid mixed with chemicals and proppant to be injected into the ground to help increase oil and gas production. This technique began in the late 1940’s and millions of treatments have since been performed showing significant recovery rates. The 21st century has observed a significant increase in the number of wells drilled for hydrocarbon recovery. A portion of these wells utilize monitoring technologies, i.e. microseismic mapping to characterize fracture growth, to improve well development and production. The hydraulic fracturing process generates hundreds and thousands of microseismic events, whose magnitudes are too small to be felt at surface. Generally speaking, the actual hydraulic fracturing operation does not pose any risk. The only circumstance that would cause an issue is a rare situation in which the stress induced by the fracture and the fracturing fluid communicates with an existing and critically stressed fault of concern (ISWG, 2015). There have been some reported cases in which induced seismicity is said to be associated with hydraulic fracturing operations, both in Canada and the USA, but the science is ongoing and the research needs to continue so that the risks can be identified and managed.
Figure 2 is an example of the frequency of events that happen versus magnitude for hydraulic fracturing operations in several major basins (Warpinski, 2014). Most of the events in this example occur below Mw = 0, but do not exceed Mw = 1. Microseismic events occur frequently and are commonly not felt by humans. As a frame of reference, earthquakes Mw > 0 are just barely felt at surface, but most induced seismic events are Mw < 5 and are rarely felt by humans (Majer et al., 2012).
About induced seismicity
A survey issued to the CSEG membership in August 2014, asked “how would you describe induced seismicity?” The results in Figure 3 show that 76% of the respondents agreed that they are “seismic events that would have occurred naturally, but are accelerated by man-made activities”. A number of respondents, 41% to 63%, agreed that they are related to man-made sources such as being dam induced, associated with geothermal activity, induced by mining, CO2 injection, hydraulic fracturing and waste water disposal. Less than 10% agreed they are naturally occurring. A detailed summary of the results are provided in the report by Boroumand (2015).
Induced seismicity has been found to occur in the shallower part of the continental crust, near the depths at which the injection wells are drilled, e.g. < 6km depth, while most natural earthquakes on continents occur much deeper, e.g. within 30km of the surface (Shedlock and Pakiser, 2013). Their shallow depth could explain why some of the magnitudes around Mw ≈ 2 have been reported to be felt at surface. Moreover, the results presented by McNamara et al. (2015), for the earthquake occurrences in Oklahoma, suggested that the majority of the induced events seem to be confined to the shallow crystalline basement and happening in the overlying sedimentary rock. However, each region is unique and additional studies will continue to yield new insights.
Figure 3 shows the frequency of events (center) that occur at the various magnitudes (left) and their equivalent energy release (right). For reference, “great earthquakes” (i.e. Mw ≥ 8), occur about once per year, but smaller seismic events (e.g. Mw < 2) occur several hundred times a day worldwide and are rarely felt at surface (IRIS, 2011). Microseismic events resulting from hydraulic fracturing are show below the graph for reference. Thousands of these events occur yearly from hydraulic fracturing operations and cause no harm to humans. The sizes of these events are too small to be felt at surface (See figure 2). Moreover, most induced seismicity are less than Mw = 5, are short in duration and the primary hazard is ground shaking.
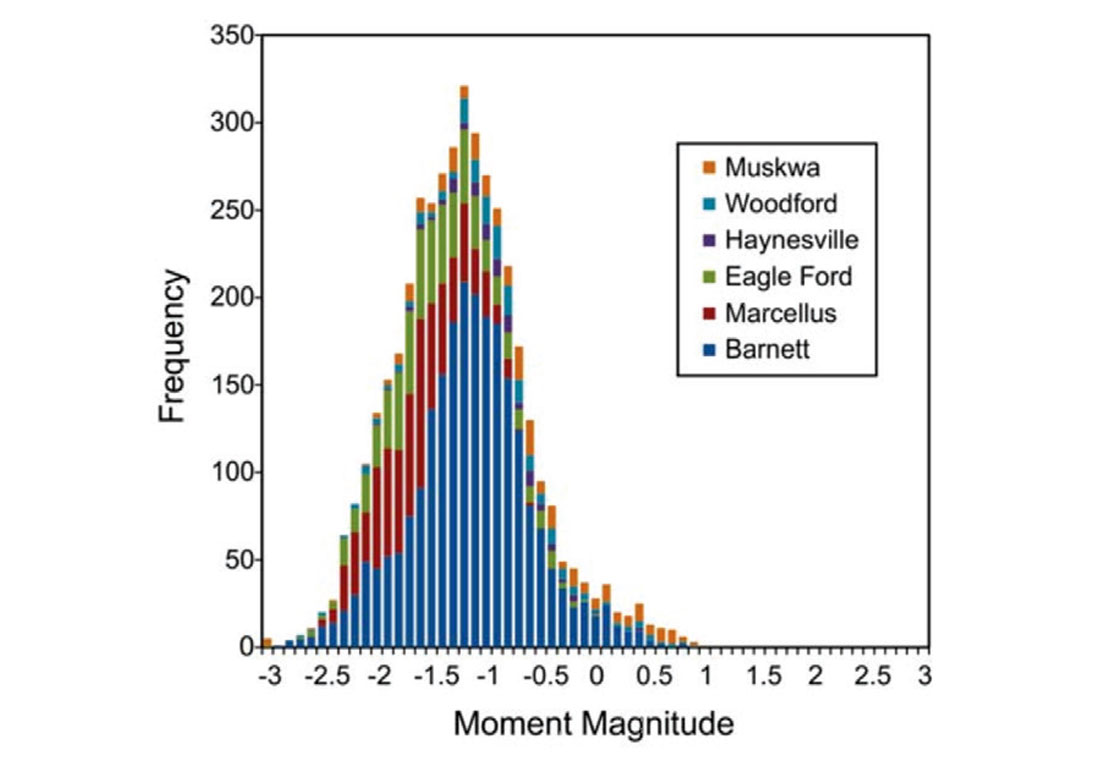
Unlike induced seismic events, microseismic events are an expected side effect of the hydraulic fracturing process. They require local seismic stations, i.e. within 100’s of meters of the expected hypocenters, in order to record and locate the events with enough accuracy. Similar to induced seismic events and earthquakes, microseisms occur as a result of a changing stress and pore pressure field. Understanding empirical features of seismicity and source properties such as frequency-magnitude distribution and stress drop of earthquakes can provide more insights into underlying mechanism governing the earthquakes as well as the inherent similarity and differences between tectonic and induced seismicity (Maghsoudi 2014).
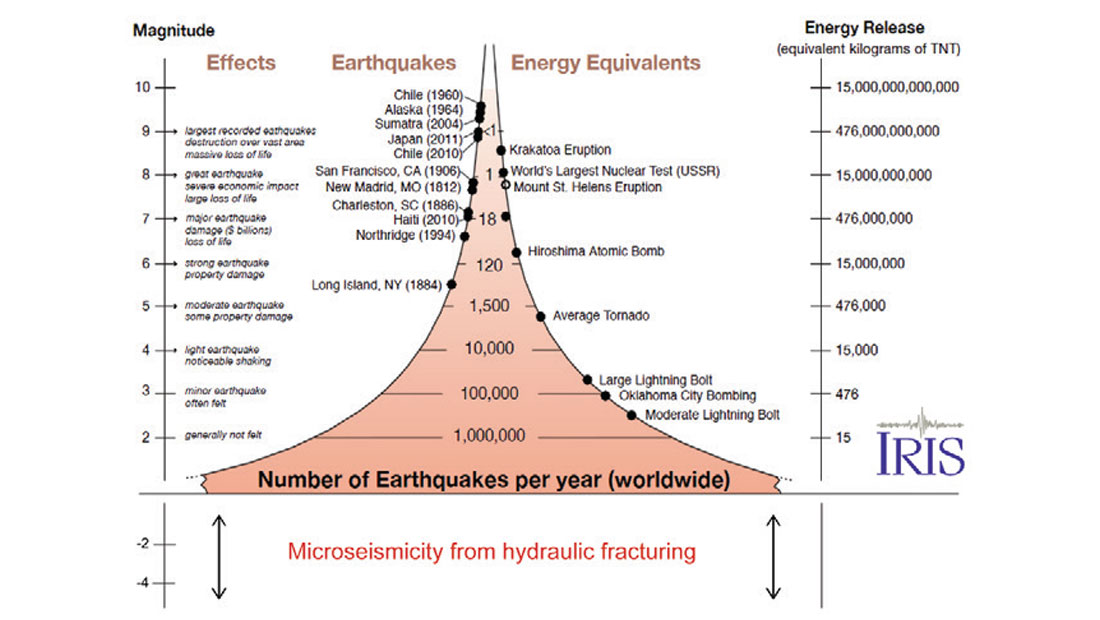
Unconventional reservoirs vary in their definition, but are generally described as volumes of rock that have restricted flow that contain trapped hydrocarbons. They are of particular interest because they are abundant, compared to conventional reservoir, but because of their low permeability composition, are harder to access and expensive to develop. Figure 4 shows the location of unconventional resources including shale gas (orange), tight gas (green) and coalbed methane (light blue) throughout the World (PacWest, n.d.). Given the scale of the potential development in these areas, it is clear that a framework for reducing the risk of induced, potentially induced and triggered seismicity from fluid injection is necessary.
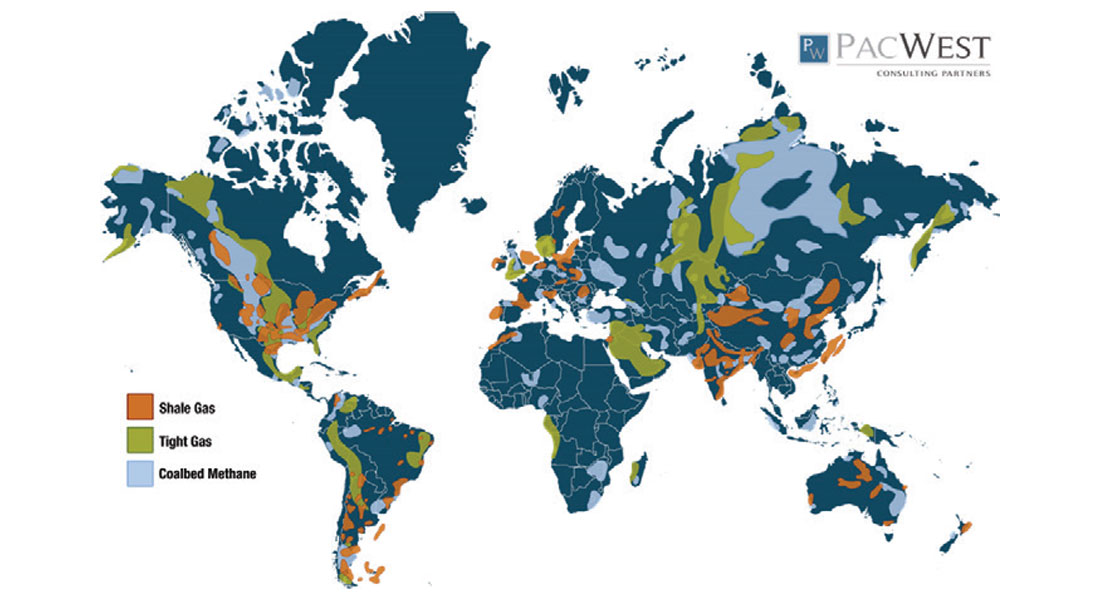
Progress and resources available
First, we start by acknowledging that shortly after the industry became aware of potentially induced seismicity; it organized the first stakeholder meeting in November 2013. This was initiated by the Microseismic Subcommittee of the Canadian Society of Exploration Geophysicists (CSEG) Chief Geophysicists Forum (CGF) (CGF, n.d.). “The objective of that forum was information sharing to establish a common technical basis and identify a vision for environmentally sound operational practices for injection projects. It had representation from government agencies, regulatory bodies, industry organizations and utility, operating and service companies” (Boroumand, 2015). The breakdown by representation is shown in Figure 5. Subsequent forums, meetings and workshops on this topic were broadened and representation from the various groups was increased.
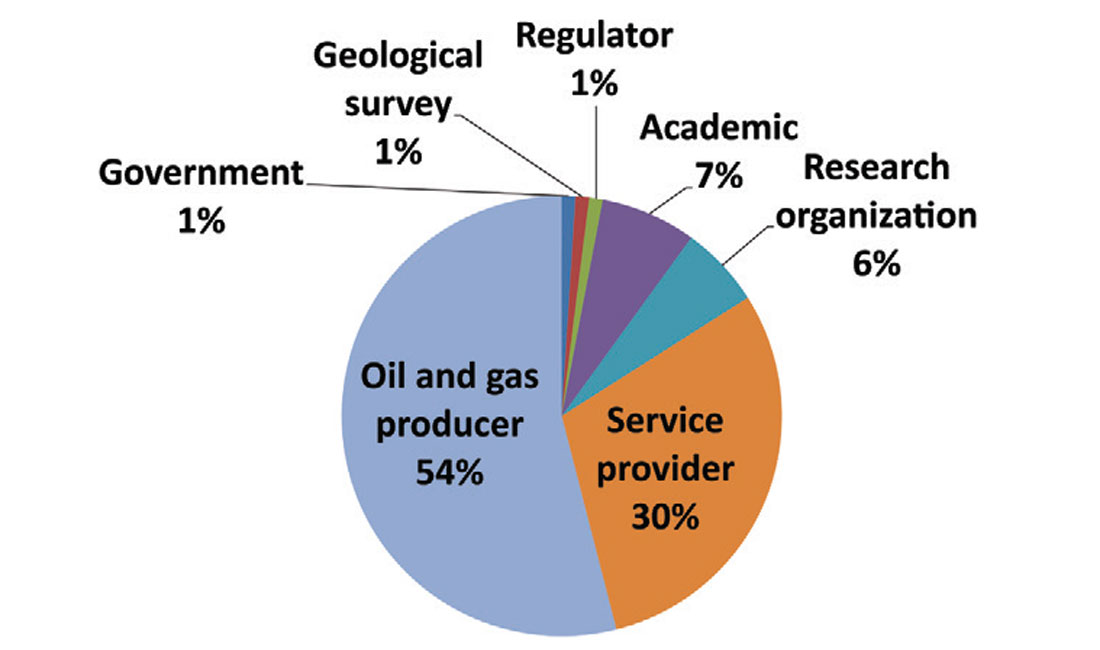
There have already been several efforts made on producing best practices for oil and gas resource development. In 2012, the Canadian Association of Petroleum Producers (CAPP) published a document on guiding principles for hydraulic fracturing and seven operating practices for shale gas and tight gas development (CAPP, n.d.). The Alberta Energy Regulator (AER) issued subsurface order #2 which imposed new seismic monitoring reporting requirements for hydraulic fracturing operations in the Fox Creek area of the Duvernay Zone in Alberta (AER, n.d.). It currently uses a traffic light protocol which states that, 1) if ML < 2, no action is required, 2) if ML > 2, the AER must be informed and a response plan must be invoked and 3) if ML > 4, operations must be ceased and the AER must be informed before proceeding with operations. Currently, this is a non-prescriptive response plan. It does not clearly account for location error, timing of events as it relates to operations, the type of seismic stations that should be used and array density or size. But, as the dialogue continues between industry, regulators, government, academics and the public, some recommendations will eventually emerge and fit-for-purpose strategies will be executed on.
In British Columbia, the Oil and Gas Commission (OGC) has generated two reports on induced seismicity for the Horn River Basin (BCOGC, 2012) and Montney trend (BCOGC, 2014).
The Alberta Geological Survey (AGS) of AER is currently acquiring data from seismic stations to understand seismicity patterns in Alberta. The AGS is currently working with the University of Alberta (UofA), University of Calgary (UofC), Western University (WU) and Natural Resources Canada (NRCan) to increase the density of, install and maintain the seismic stations.
The Geological Survey of Canada (GSC) of NRCan’s Earth Sciences sector (ESS), among its many strategic objectives, is focused on understanding how hydraulic fracturing in shale basins induce seismic hazards and risks to groundwater (GEOSCAN, n.d.). Earthquake locations (See example in figure 6) and seismic stations can be viewed on NRCan’s website. You can also track significant earthquake activity in Canada by following the Earthquakes Canada twitter account at @CANADAquakes.
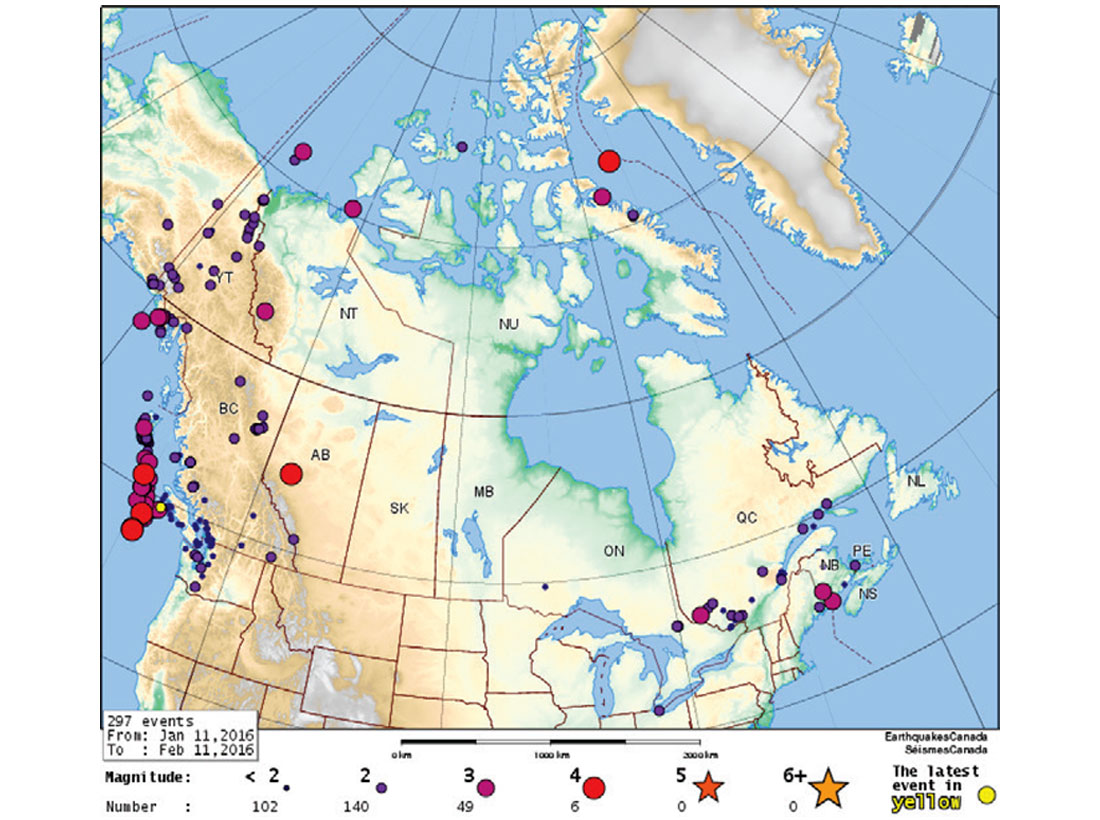
Ongoing efforts
There are several efforts underway by academic groups in Canada, the USA and around the World. We point out only a few of these organizations and their overall research themes: 1) University of Calgary is currently engaged with Industry, Government and Regulators to study induced seismicity associated with fluid injection, 2) the University of British Columbia is focused on induced seismicity in N-E BC’s Horn River Basin, 3) Western University interests in analysis of ground motion from earthquakes and 4) Stanford University has started a Center for Induced and Triggered Seismicity Consortium. There are many more established and ongoing efforts in North America and globally that we do not mention here.
The recent report published by the StatesFirst Induced Seismicity by Injection Work Group (ISWG) which stated that it is “important to develop a communication and response strategy before a response to an incident is needed”. They suggest that the strategy development plan will need to be based on the unique regulatory framework of that jurisdiction, but may generally follow:
- Planning before the event
- Implementing a response
- Evaluating after the response.
The ISWG also suggests that the strategy could focus on issuing public surveys, develop working relationships to educate the public and establish open communication lines with stakeholders so that perceptions and concerns are heard.
Cross disciplinary expertise may be needed to establish a science-based risk management and mitigation plan. Due to the site-specific considerations and technical complexities, multiple specializes may be needed to examine the risk of induced seismicity. These experts may include: Geophysics, Geology, Seismology, Geomechanics, Earthquake Engineering, Reservoir and Subsurface Engineering and Civil and Structural Engineeing (after AXPC SME, 2012).
The research is ongoing and includes: studying stress distribution, pore pressure, risk analysis and permeability, doing site characterization using 3D seismic to identify and predict slip on faults, conducting preliminary job modeling for risk assessment and lastly data integration which means assembling a multi-disciplinary team to evaluate all of these questions. There are many techniques developed to evaluate stress and pore pressure changes in the subsurface. This approach would consider available input data, data quality and uncertainty, analytical calculations, computational models which stimulate 3D reservoir with fluid and stress interactions, reservoir geomechanics, seismic and geology.
Join the Conversation
Interested in starting, or contributing to a conversation about an article or issue of the RECORDER? Join our CSEG LinkedIn Group.
Share This Article