The evolution of the North American “unconventionals revolution”
The discovery in the early 1990s by George Mitchell and his team at Mitchell Energy of how to profitably exploit the Barnett Shale play fuelled the North American unconventionals revolution, aided by (1) oil and gas price increases; (2) improvements in drilling and completions technologies; (3) readily available capital and credit; and (4) changes in the international business environment including the rise of political risk in many countries, and a decline in the number of licensing rounds in prospective areas outside of North America [1].
Early euphoria regarding the prospects of these new super-large “low-risk” resource plays was contagious. Encana, a “growth-oriented pure-play natural gas company” and industry leader (used as a proxy for the unconventionals industry in this article), made headlines in March 2010 [2] when Randy Eresman, then President and CEO, told institutional investors at the company’s Investor Day in Calgary: “We are so convinced of our potential, in fact, that we have set the goal of doubling Encana’s production over the next five years.” They were not alone, and annual industry CAPEX associated with unconventional plays increased from around $5 billion in 2006 to more than $80 billion in 2013 [3]. The unconventionals revolution significantly increased oil and gas production in North America, and displaced many smaller onshore complex structural and stratigraphic exploration and development projects.
The Common Risk Segment mapping approach is used by the geoscience team to identify unconventional resource play regional sweet spots. During this process, maps of the first-order factors that impact recovery potential, such as reservoir thickness, depth and pressure are superimposed, and the reservoir sweet spots are identified as the places where the critical play elements overlap. Development drilling often follows EnCana’s Gas Factory strategy, whereby multiple wells are drilled from the same surface location (well pad) and simultaneous operations are employed to capture economies of scale [4]. In the days of robust oil and gas prices, this approach encouraged the placement of the well pads on a regular surface grid, and the development of these resource plays was considered to be largely an engineering optimization process.
Time and the law of supply and demand have tempered the euphoria surrounding unconventional plays. This was, to a certain degree, predicted: “In this new energy paradigm, we expect natural gas supply to outpace demand and prices to be lower than recent historical averages.” [2] Declines in gas, then oil, prices led to the shrinking of the size and profitability of the regional economic sweet spots of these plays. Figure 1 shows how Marcellus shale formation drilling has become concentrated in certain discrete sweet spots since 2011, and also shows the Marcellus thickness map.
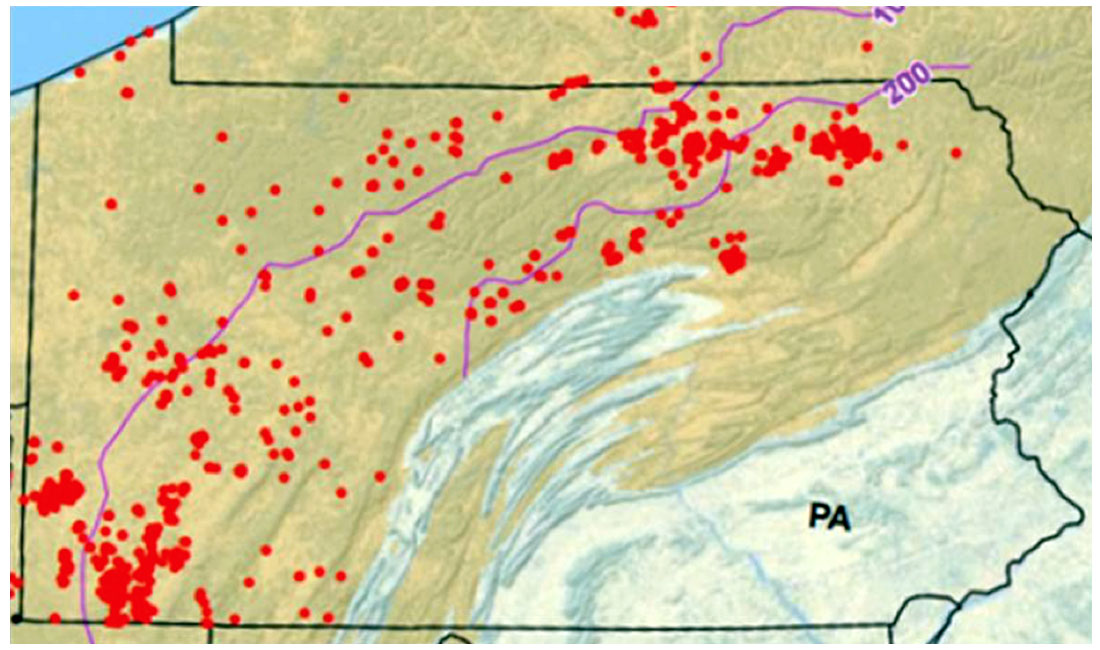
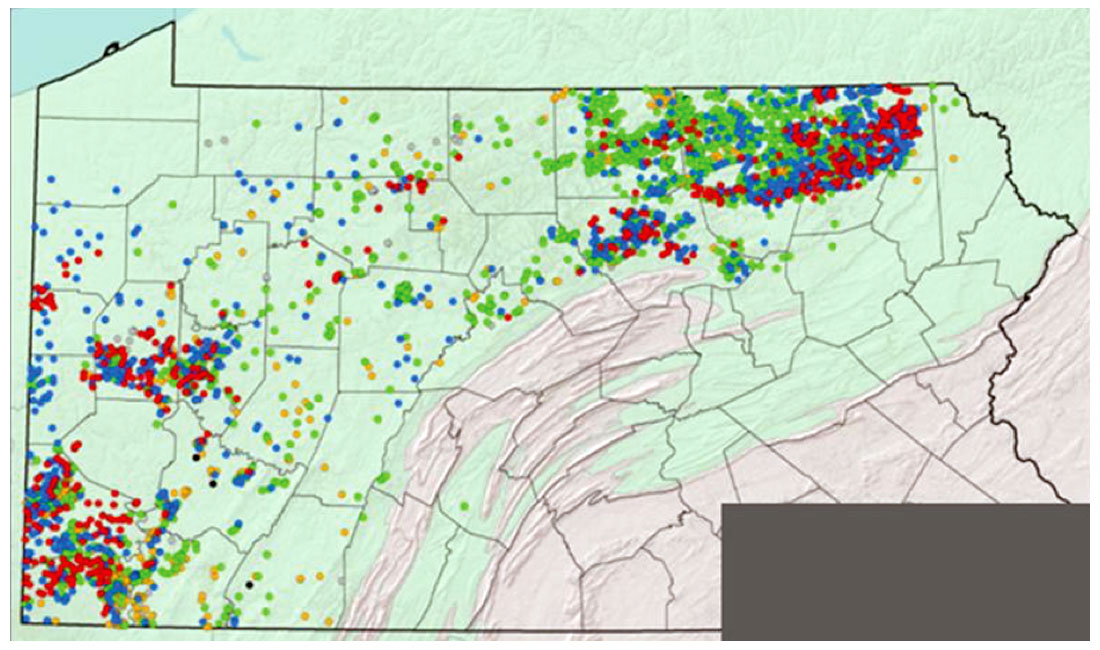
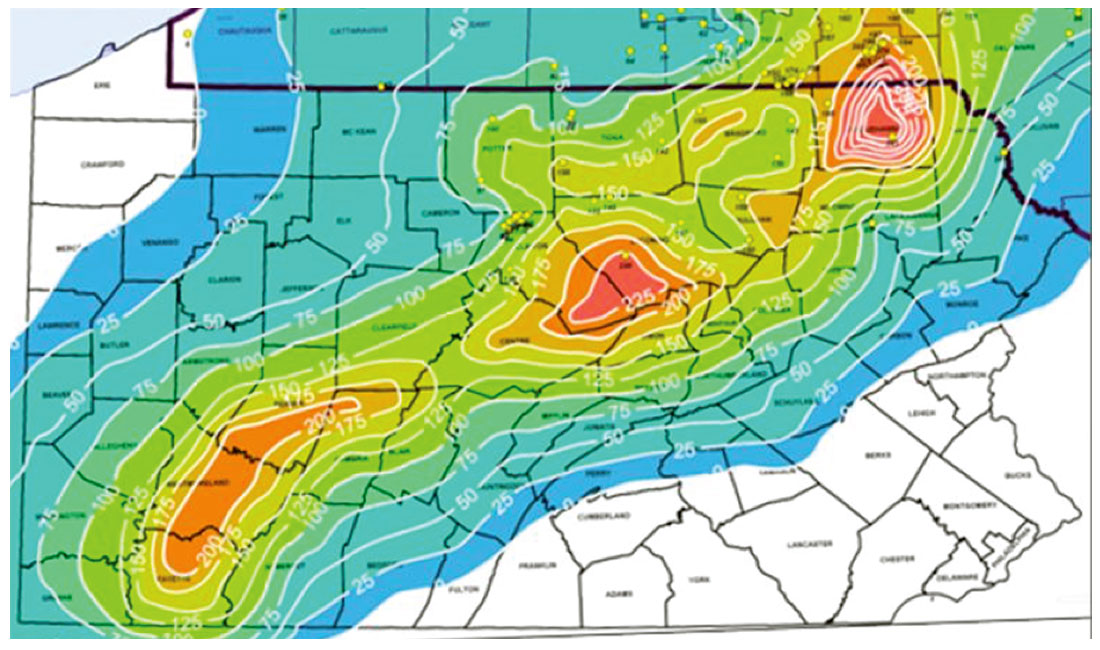
The impact of the gas price crash of 2008 was tempered by the high prices that oil and NGLs realized at the time. Heavily gas-weighted operators revised their strategies and sought out new liquids-rich plays. Then came the 2014 oil crash. The resulting impact has been huge. Operators, burdened by the debt accrued entering these plays, were forced to rapidly slash CAPEX and OPEX and delay projects, based on parallels drawn with, and lessons learnt from the oil crash of 1986. This has resulted in massive job losses at both service companies and operators, and system-wide financial stress. Despite its early leadership position in unconventionals, Encana was driven to completely change its strategy from that of 2010 (it now focuses on just four “high-margin liquids plays and low-cost natural gas plays”), and was forced to reduce its workforce by “55 percent in just over 2 years”, according to current CEO Doug Suttles (24 February 2016) [6].
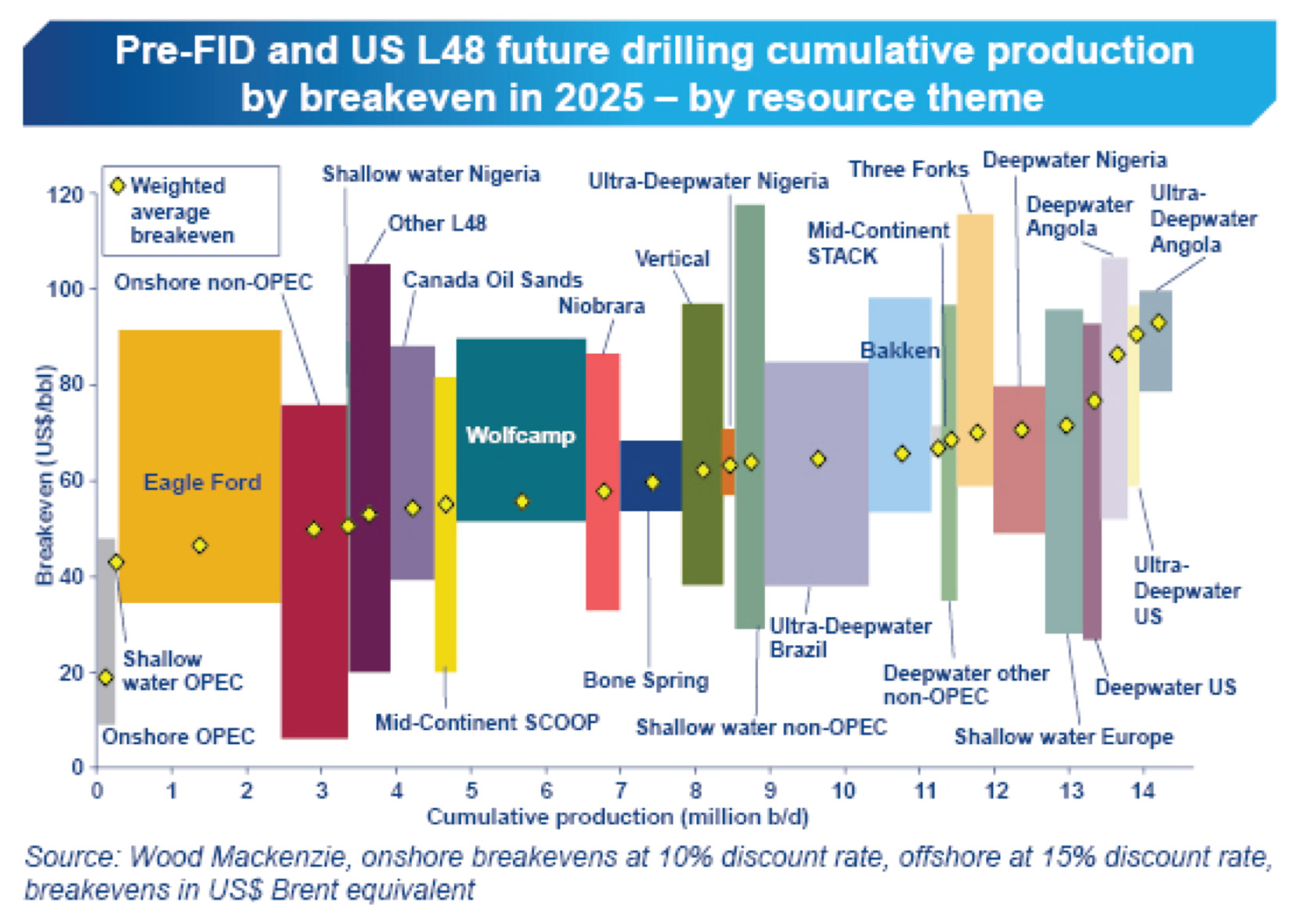
Despite recent improvements in operational efficiencies and reduced costs, operators are still economically challenged as today’s oil price falls below the average breakeven price of many North American unconventional plays (Figure 2). Bankruptcies are increasing as companies struggle to meet their debt repayment obligations [7], and perhaps the full impact of the low oil price has yet to be felt.
Where is the value of geophysics for mature unconventional plays?
When operators start to get a foothold in a new unconventional resource play, a significant amount of effort is devoted to characterizing and mapping the play. This is done at the same time as the first wells are being drilled and completed to test the critical play concepts. Over time, land positions become established, and the successful projects mature into development projects which are ready for “harvesting”. Then the full weight of the Gas Factory engineering optimization approach is applied, with the goal of turning the play concept into economic reality.
It would be quite convenient at the harvesting stage to simplify the reservoir in the development area, and we may perhaps be tempted to treat it as being homogeneous. The reality is that the subsurface is always more complex than we would like to think, and it is also more complex than our limited data suggests.
Geophysics has three main applications at the harvesting phase of a mature resource play – geohazard mapping, detailed reservoir characterization, and completions monitoring. It should always be at the forefront of our minds that: (i) we will eventually encounter each and every subsurface geohazard in our development area as we drill more and more wells into our reservoir; and (ii) that all accidents that occur in the field are first designed in the office. The $61.6 billion BP Macondo disaster reinforced the learning that the personal and financial costs of HSE incidents can far outweigh the savings achieved through short-term cost cutting programs.
We struggle as geophysicists with geohazard mapping and detailed reservoir characterization for many of our shale reservoirs. First, our standard seismic processing workflows have difficulty imaging low reflectivity, relatively noisy shale reservoirs with sufficient resolution to resolve the internal reservoir architecture and many of the subtle structures and low-throw faults that are associated with gas kicks or cause other problems during drilling (Figure 3). Secondly, simple seismic mapping exercises and standard probabilistic reservoir characterization approaches often yield low resolution geophysical attributes, which can be hard to directly link to the reservoir properties used to estimate production (e.g. porosity and permeability).
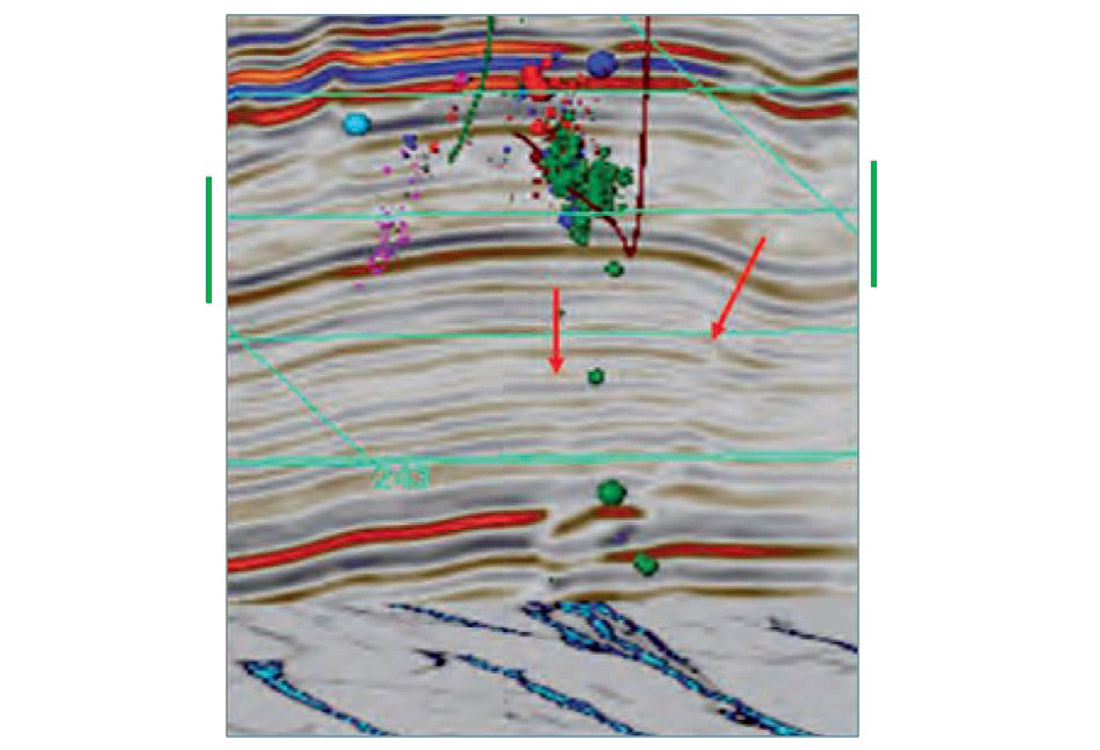
When we encounter difficulties confidently identifying subsurface geohazards and mapping critical reservoir properties, we will undoubtedly struggle to effectively communicate our value, and fail to convincingly answer the business-critical questions: “Does geophysics add additional value during the harvesting stage of unconventional shale exploitation projects? And, if so, how do we measure this value?”
Our struggle to communicate the value of geophysics, combined with the narrative that the play is ready for engineering optimization, can reinforce a false management perception that geophysics generates little value at the harvesting stage of unconventional resource plays. In my opinion, this was the root cause of the widespread layoffs of geophysicists earlier in the current downturn.
I firmly believe that the value proposition of geophysical data increases as the price of oil and gas declines; and that we need to improve our understanding of our unconventional shale reservoirs and identify the very best drilling locations if we are to successfully negotiate our way through today’s very challenging economic headwinds.
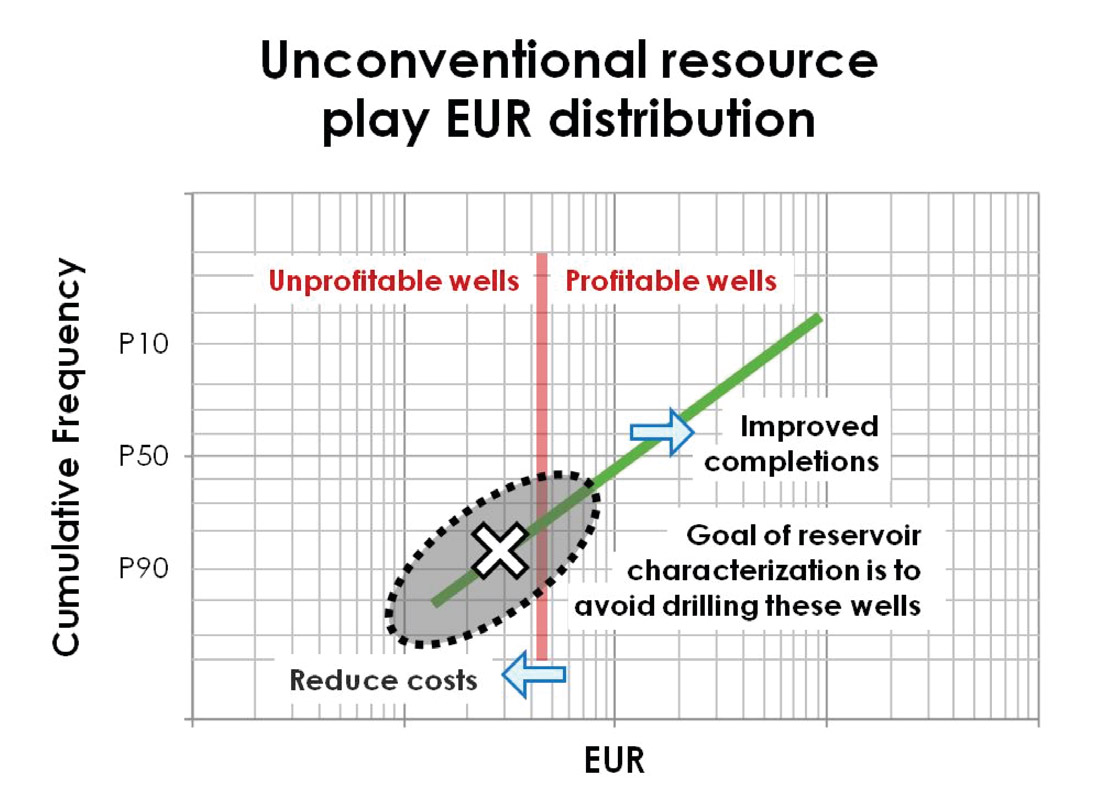
Consider, for example, a typical distribution of well EURs from a particular part of a producing shale formation, as shown by the green line in Figure 4. We observe that there are a few wells with very low production, a few with very high production, and the rest are somewhere in the middle. We do not have the same production from well to well because reservoirs are not homogeneous. The EUR required for an individual well to be profitable is sensitive to the prices of oil and gas, and increases as prices decrease. For a successful venture, the average (P50) well needs to be profitable. We can improve our profitability by improving drilling and completion techniques, and by cutting costs. However we still have a range of well performances, and we will still drill wells that are not profitable. I suggest that the only way we will understand this spread of EURs is by fully characterizing our reservoirs using geophysical and other subsurface data. Then we will be able to avoid drilling the wells that will never be economic, and beat the statistical odds.
Quantifying the value of geophysics for unconventional plays
To counter the false perception that geophysics has little value at any stage in a project, every geophysicist has to be able to express their value in the language of the executive team, and articulate how geophysics: (1) improves business decisions and risk management; (2) reduces uncertainty, cycle time, CAPEX or OPEX; (3) increases the accuracy of reserves estimates; and/or (4) accelerates or increases production.
We need to do more than this. During tough economic times, the executive team will naturally implement policies that decrease costs, as the savings flow through to the bottom line. Unfortunately, the bottom line impact of long-term value destruction that arises from program cuts is hard to measure, and is therefore easily overlooked. This puts those of us who work on projects directed towards generating longer term value in a precarious position. We must go beyond simply describing the value proposition of our work. We need to be able to quantify exactly how much geophysical data and analyses improve the key project economic metrics – VIR (value investment ratio), NPV (net present value), and ROACE (return on average capital employed).
This is not easily done. Decision tree analysis effectively calculates the value of geophysical information for conventional plays, during which a relatively low number of relatively expensive wells are drilled. In contrast, a number of factors make it quite difficult to quantify the value of geophysical information during the harvesting phase of an unconventional shale project, when hundreds to thousands of wells are to be drilled, including:
- Unconventional resource development projects are far larger and more complex than typical conventional exploration and production projects. Reservoir behavior is not fully understood, and the key factors that impact production vary from play to play. Due to complexities associated with the sheer size of the resource play, there’s more to a successful development than just finding the subsurface sweet spots. To achieve efficiencies of scale, many laterals are drilled from the same well pad, and decisions around the pace of development and the locations of the well pads are driven not just by subsurface factors, but are also heavily influenced by the availability of land and infrastructure, as well as other economic and non-technical factors.
- The uses of, and value extracted from, geophysics are dynamic and subjective. As we are still on the relatively steep part of the learning curve for unconventionals, the perception of value of different geophysical techniques goes up and down over time, and varies from person to person and from company to company. For example, there are divergent opinions about the validity of natural fracture network property predictions generated by the analysis of azimuthally-varying seismic attributes.
The reality is that rather than providing critical information that drives key project decisions at the harvesting stage of an unconventional project, geophysical analysis is just one of a large number of inputs that are taken into consideration when development decisions are made. In such a system, economic modeling is required to estimate the value of the different sources of information used for decision making, and their impact on the total project economics. Even when changes are recommended after geophysical analysis, a significant number of hurdles, not the least being organizational inertia, need to be overcome before they are implemented and the value realized.
The bottom line, however, is that despite their best efforts, drilling and completions engineers can only improve production so much; and accountants can only cut costs so much. The quality of the reservoir is the dominant factor in determining returns. Ultimately, therefore, we need geophysicists, expert at using geophysical data and analysis techniques to improve our collective understanding of the subsurface.
Identifying the best reservoir within and outside of our current land holdings enables us to drill the best wells first to pay down accrued debt; and helps us to acquire and divest acreage to upgrade our drilling portfolio. This is critical for long term success.
Four ways that we can increase the value of geophysical data for mature unconventional plays
The power of 3D seismic data lies in the fact that it is our only data that enables us to observe and measure changes within our reservoir between our existing wells. We can move beyond basic 3D seismic mapping, and improve our reservoir understanding, by jointly analyzing seismic and microseismic data (Figure 5). Microseismic data gives us clues about the interaction between the hydraulic fracs and the reservoir. Seismic data helps us understand why the microseismic data, and by inference, why the hydraulic frac behaviour changesspatially.
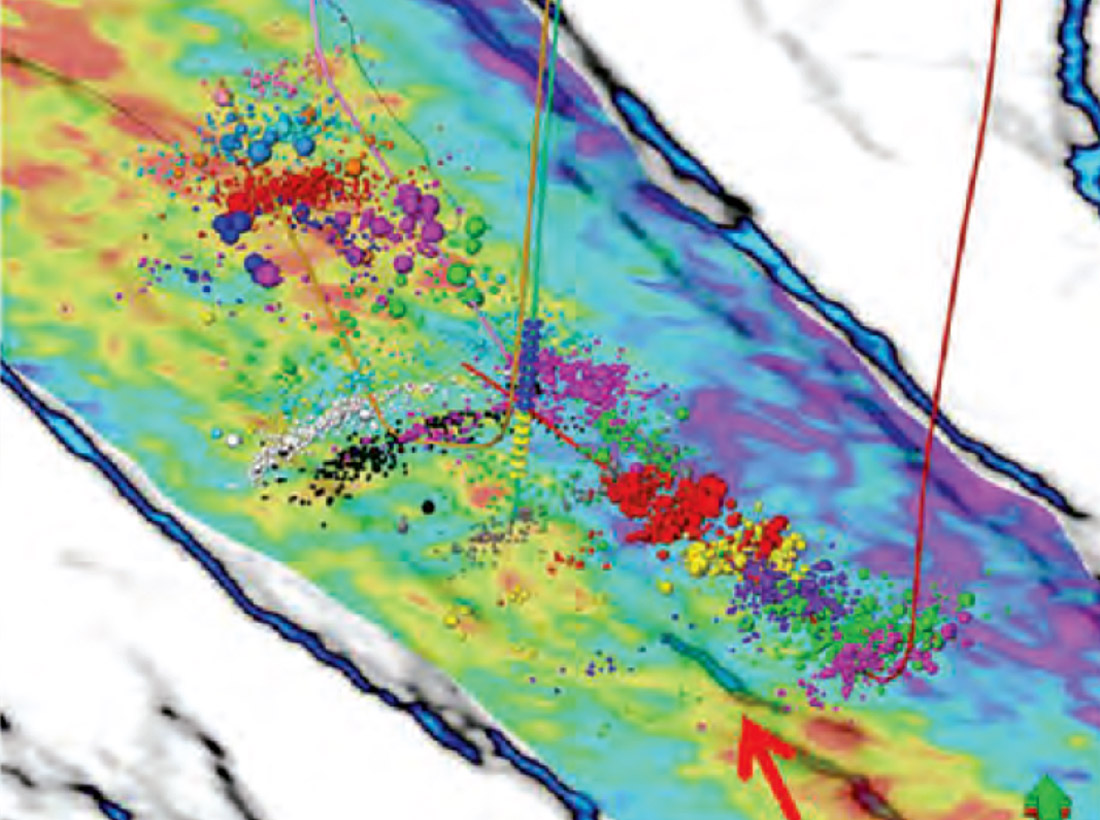
For maximum impact, we need to apply new techniques to increase the value extracted from 3D seismic data. Over the last decade or so, a number of approaches have been used to do this for conventional plays, including:
- Acquiring seismic data at a higher density has significantly improved subsurface imaging quality for shallow heavy oil plays, for complex structure plays beneath the desert in the Middle East, and for offshore subsalt targets.
- Improving seismic imaging workflows through the use of more advanced migration algorithms such as anisotropic Kirchhoff pre-stack depth migration and reverse time migration. More improvements will come from emerging technologies such as least squares migration.
- Interpreting new attributes including spectral decomposition, curvature and fault tracking attributes have enabled us to identify subtle facies changes and structural details in our 3D seismic volumes.
- Using rock physics models to separate reservoir from non-reservoir facies, and to predict the types of fluids within our reservoirs.
With the exception of increasing our acquisition intensity (too costly), these newer techniques are now being applied to improve our understanding of onshore unconventional plays. Whilst the lessons learnt from one play type do not translate seamlessly across to other play types, the underlying principles do.
We are therefore now moving up a new learning curve for unconventional plays (Figure 6). We first applied conventional interpretation techniques to map the structural variations of our plays, identify the major faults, and look for simple correlations between attributes and EUR. We then applied experimental techniques such as azimuthal analysis to see if we could identify which areas of our reservoir have a higher density of natural fractures, and to see if we could correlate the predicted fracture properties with EUR. More sophisticated, recently- developed approaches try to predict internal reservoir properties such as facies or mineralogy, which enable us to visualize how the reservoir changes spatially and temporally. The final step is to use integrated workflows to link geophysical data to the key reservoir and completions properties.
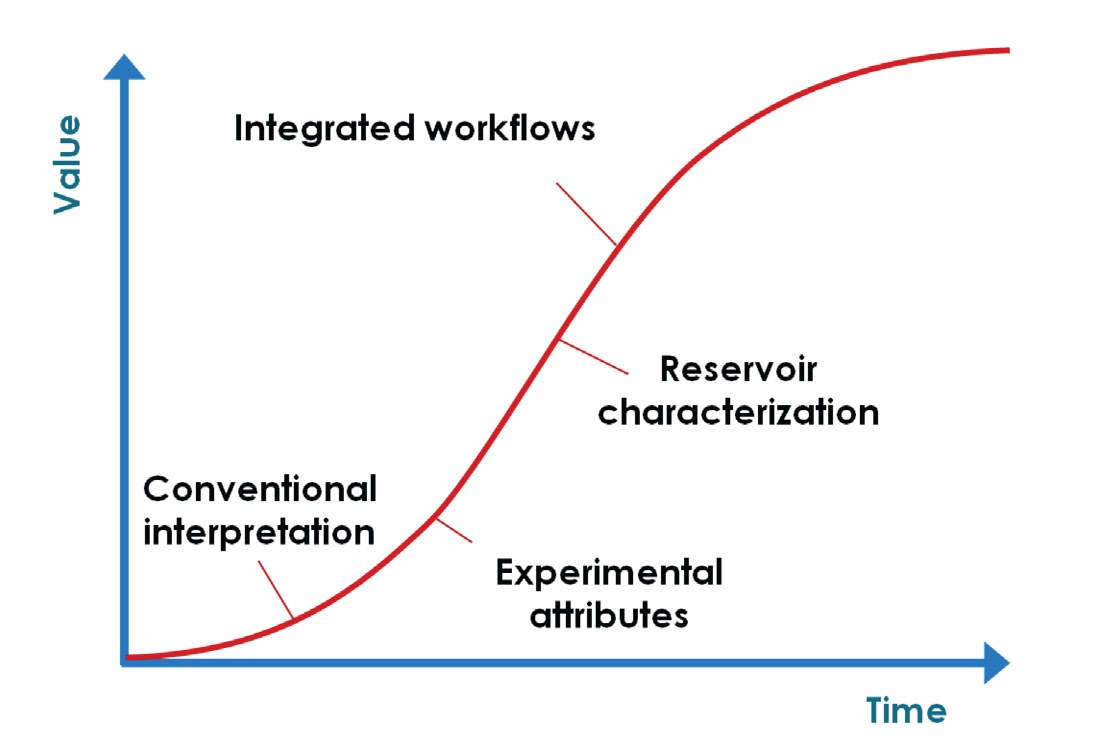
It is my opinion that there are four areas where we can extract significantly more value from our existing geophysical data than we commonly do right now, namely:
- Seismic processing
- Reservoir characterization
- Proactive management of induced seismicity
- Data integration
1. Improved seismic imaging
We can improve our seismic imaging by applying new workflows and algorithms (e.g. Figure 7). I recommend the use of anisotropic pre-stack depth migration for every imaging project. Anisotropic pre-stack depth migration focuses features such as faults and channel flanks better than pre-stack time migration, even in unstructured areas. Beyond this, imaging improvements may be obtained by tuning up many parts of the standard processing workflow including statics, noise attenuation and migration pre-conditioning. Cascading many small process improvements results in a noticeable increase in the bandwidth and signal-to-noise ratio of the output migrated pre-stack and post-stack data, and ultimately increases the quality of information extracted from within our low reflectivity unconventional reservoir zones.
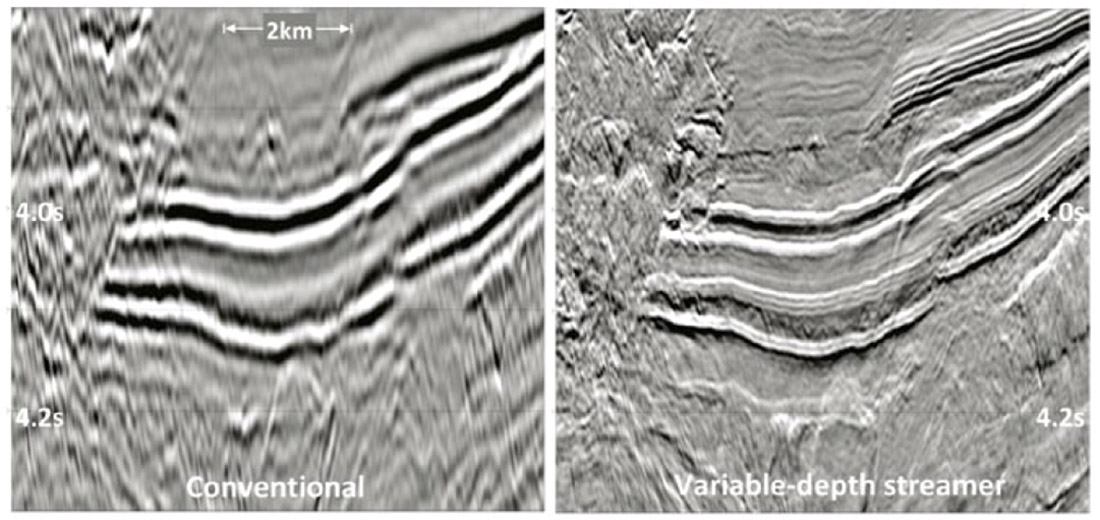
I am sure that there are tremendous reprocessing opportunities for the seismic processing companies that are able to achieve significant improvements in onshore seismic data quality, similar to those shown in Figure 7. Such improvements are doubly of importance, since this data is used for both reservoir characterization and drilling hazard studies.
2. Quantitative reservoir characterization
Secondly, I am convinced that the application of new reservoir characterization workflows is a critical step towards improving our understanding of our shale reservoirs, and for our search for regional and local sweet spots. These workflows are enabled by rock physics models, applied at both well log and seismic scales.
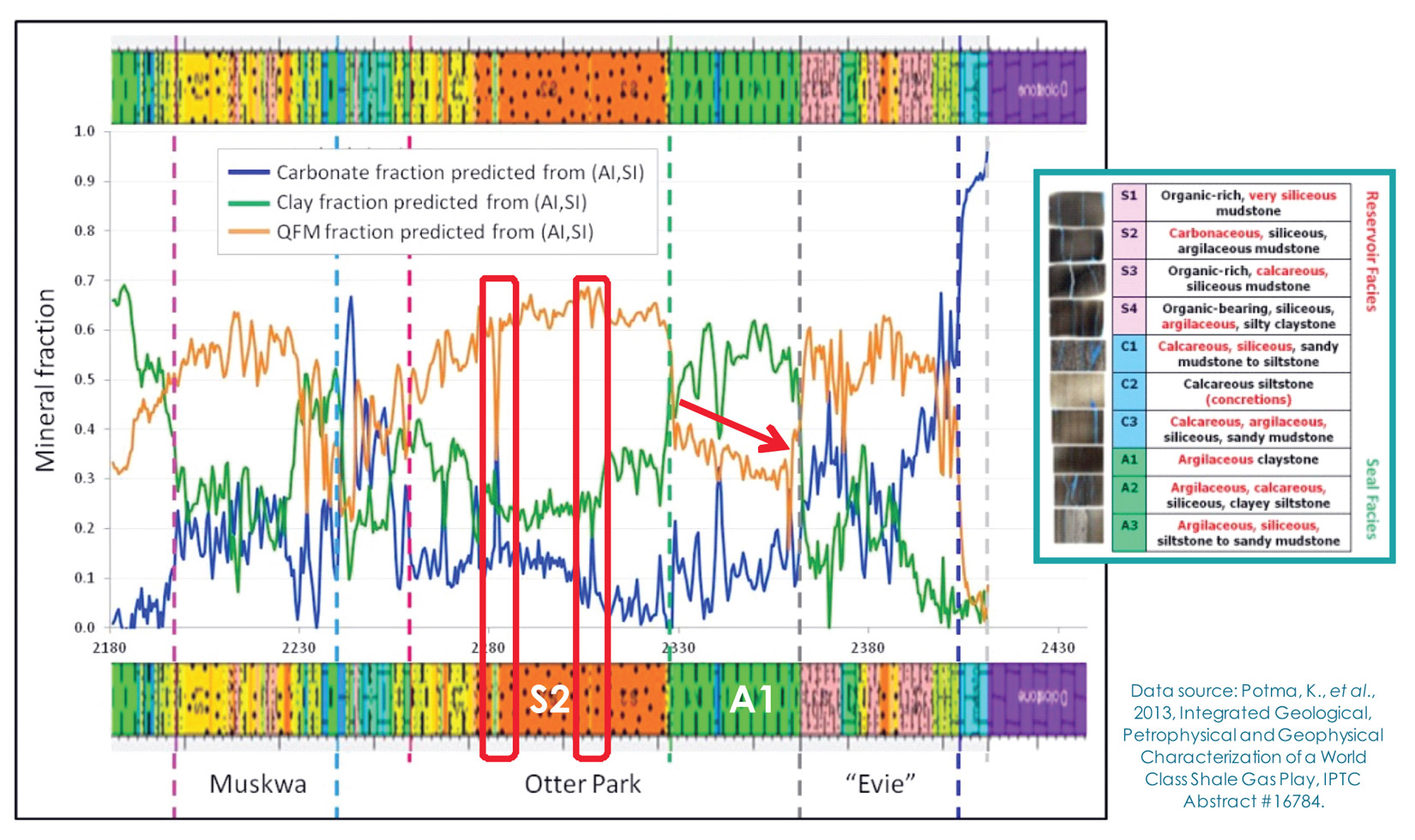
At the log scale, for example, we can use rock physics models to predict mineralogy from elastic rock properties (i.e. AI and Vp/Vs) calculated from our sonic and density logs. In the example shown in Figure 8, the model was calibrated using the available lithofacies data. Here, as expected, the siliceous mudstones shown in yellow and orange have a high quartz content, the claystones in green have high clay content, and the more carbonaceous siltstones in light blue and the carbonates in blue and purple have high carbonate content. We also observe a good agreement between the predictions and our expectations at all scales, and find additional information in the predictions that was barely or not at all resolved in the more blocky lithofacies data (red boxes and arrow).
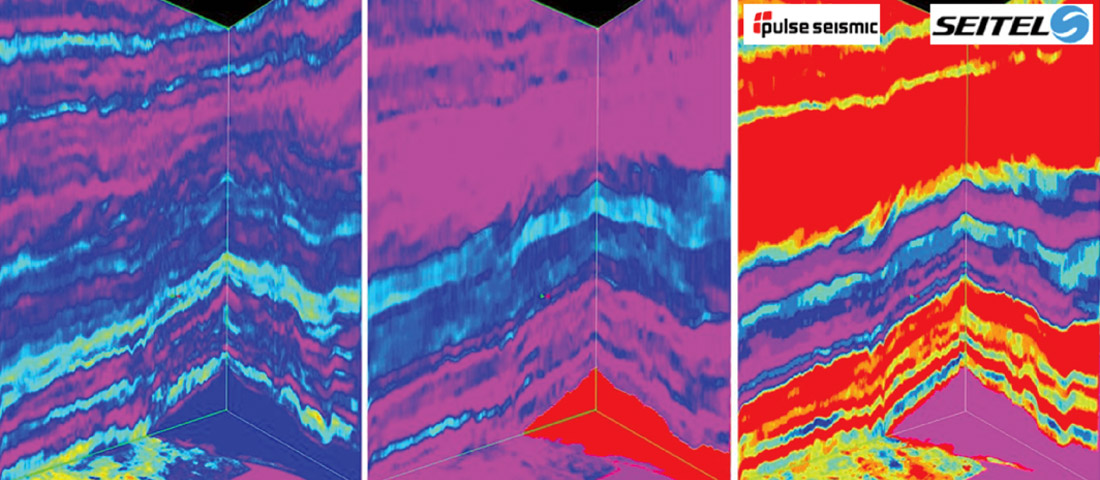
Once a rock physics model has been built and calibrated, it can be applied to all of the other wells in the surrounding area, including legacy wells. We can also use the rock physics model to transform 2D or 3D seismic AVO inversion data into volumes of predicted quartz, clay and carbonate, as shown in Figure 9. Different geological units are readily identified on these mineral volumes. Potential reservoir units may be identified on the quartz volume (left). Transforming seismic data into geological data in this way makes it far more accessible to technical experts from other subsurface disciplines, and also to management.
3. Pro-active management of induced seismicity
My third example addresses the issue of induced seismicity, which is a topic of growing and justifiable public concern.
Our licenses to operate are in danger of being withdrawn when our fracking or water injection operations generate high magnitude seismic events that are clearly felt at the surface. Currently we use a reactive approach for managing this risk, as we stop our operations when the magnitude of the induced seismicity exceeds a predetermined threshold.
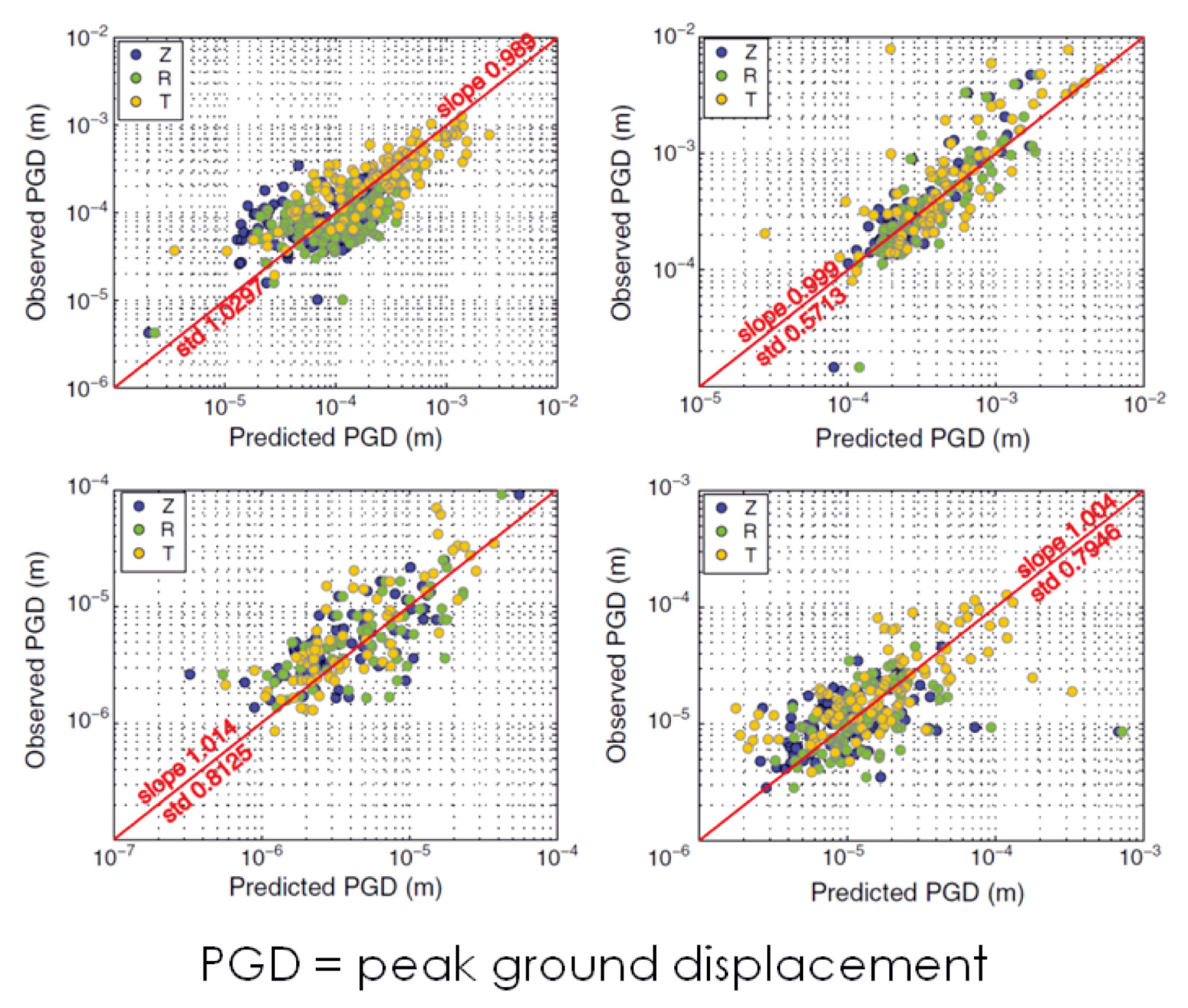
The next step is to become proactive in our management of induced seismicity, and predict how the felt effects vary from one surface location to the next. Marine Denolle showed in her 2013 Stanford University Ph.D. thesis [12] how we can characterize the near surface using ambient geophysical data. The amount of ground motion generated by the induced seismicity appears to correlate with these near surface properties (Figure 10).
I suggest that this is a new area of geophysical analysis that warrants investigation, particularly for operators who are generating significant amounts of felt seismicity near population centers, sour gas processing plants and pipelines, and other critical infrastructure.
4. Integrated workflows
We observe correlations between petrophysical (log and/or core) and geophysical (seismic) properties in some unconventional plays. The microseismic behavior of hydraulic fracs, and reservoir geomechanical properties, are calculated from Young’s modulus and Poisson’s ratio, which are derived from sonic and density logs, or from seismic data. Completions pressures vary as geomechanical properties vary. There’s a lot of synergy here!
Integrated workflows enable all of the technical subsurface and engineering disciplines to work together. In my opinion, well log and seismic geophysical data lie at the heart of these integrated workflows – a conceptual example is shown in Figure 11.
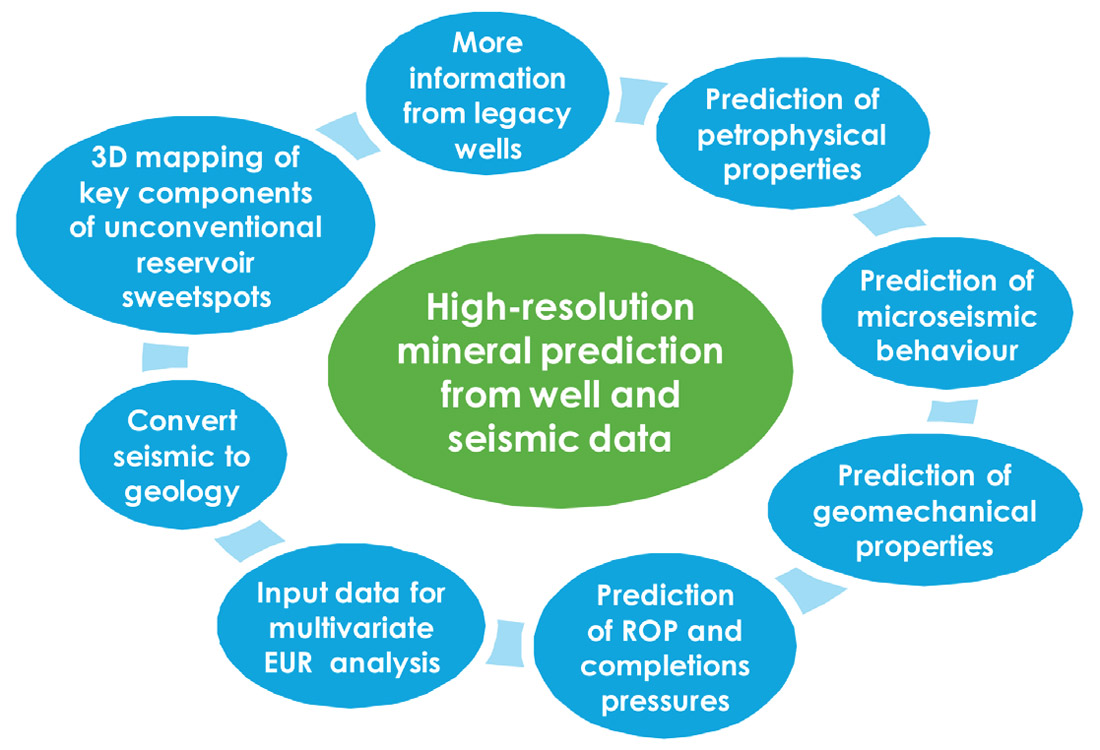
Summary
Despite the fact that the impact of subsurface characterization for unconventional resource plays increases as the prices of oil and gas fall, geophysicists have struggled to quantify their value and impact on the key project economic metrics, as this requires economic modeling and communication in the language of the executive team. I remain optimistic that there is a good future for geophysicists working on unconventional resource plays in the following areas:
- Processing: There is considerable scope for processing companies to improve the quality of their processed seismic data, to image more reflectivity within our unconventional reservoirs, and to image the subtle faults and structures that create expensive drilling problems.
- Reservoir characterization: New reservoir characterization workflows will lead to a deeper understanding of our reservoirs, the identification of subsurface sweet spots, and ultimately to better business decisions.
- Management of induced seismicity: We clearly need to demonstrate continuous improvement in order to retain our license to operate in places where induced seismicity is a justifiable public concern. We may be able to use geophysical techniques to become proactive in our management of the risks of induced seismicity.
- Data integration: Integrated workflows will unlock more value from geophysical data. It may not be easy to get everyone working together, but it is definitely possible.
Ultimately, identifying the best reservoir within and outside of our current land holdings enables us to drill the best wells first to pay down accrued debt; and also helps us to acquire and divest acreage to upgrade our drilling portfolio. The ability to demonstrate that we can develop our unconventional resources safely is critical for our industry. As is the case for all other resource plays, these are the keys to long term success.
Join the Conversation
Interested in starting, or contributing to a conversation about an article or issue of the RECORDER? Join our CSEG LinkedIn Group.
Share This Article