Introduction
Our goal with the reflection seismic experiment is to obtain the most clearly defined, high-resolution image of the subsurface geology, free of contaminating noise. This goal can be achieved by acquiring seismic surveys with dense and even sampling in both time and space.
Historically though, 3D marine streamer acquisition has been forced to compromise spatial sampling in the name of efficiency and cost. This compromise translates to noise and degraded resolution when processing the data. A significant component of the seismic “noise” contaminating 3D images actually arises during processing, as an unfortunate and inescapable artifact from poor 3D spatial sampling. Aliased data create noise during the application of any multi-channel processing operation, notably prestack migration. If the cross-line acquisition dimension could be sampled at an equally small interval as the inline dimension, a much larger frequency bandwidth than typical of standard 3D marine streamer acquisition could be preserved throughout all stages of processing, free of aliasing and free of artifacts.
The High-Density 3D (HD3D™) marine streamer acquisition method explicitly addresses the issue of tight 3D spatial sampling. HD3D acquisition offers a data product that is properly sampled in both spatial directions, is of high quality, and is cost efficient.
Since 2001 the ability to routinely tow 12 to 16 streamers at very small separation (37.5 to 50 m) has delivered a great leap forward in the marine streamer acquisition scenarios previously considered impossible – all at a comparable cost. Single-source shooting delivers high fold, increased signal-to-noise (S/N) ratio data, and inline processing fidelity, while dual-source shooting delivers the tight 3D cross-line spatial sampling required for high resolution imaging of highly dipping events.
This article includes two recent high-density 3D case studies, acquired by PGS in the NW Palawan Basin, Offshore Philippines, and in the North Madura Platform, East Java Sea area. Stringent resolution requirements and steep dip imaging were survey objectives in both cases. Consequently, survey planning recommended that both surveys be acquired with 12 streamers at close separation, in dual-source shooting mode. This approach allowed the recording of steeply-dipping data free of aliasing in any direction, and enabled a high resolution processing approach to preserve a large frequency bandwidth throughout all stages of processing. In both cases, data quality and resolution are excellent, enabling the ability to confidently interpret a highly complex set of geological features.
A powerful demonstration is made that HD3D marine streamer acquisition delivers the optimum foundation for high resolution, high quality 3D data – provided with efficiency (i.e. no loss in downtime) and with comparable cost to standard marine streamer 3D acquisition and processing.
HD3D Fundamentals
Modern seismic vessels, such as the Ramform design (Figure 1), with their massive towing capacities, have changed the way in which modern seismic data are acquired.
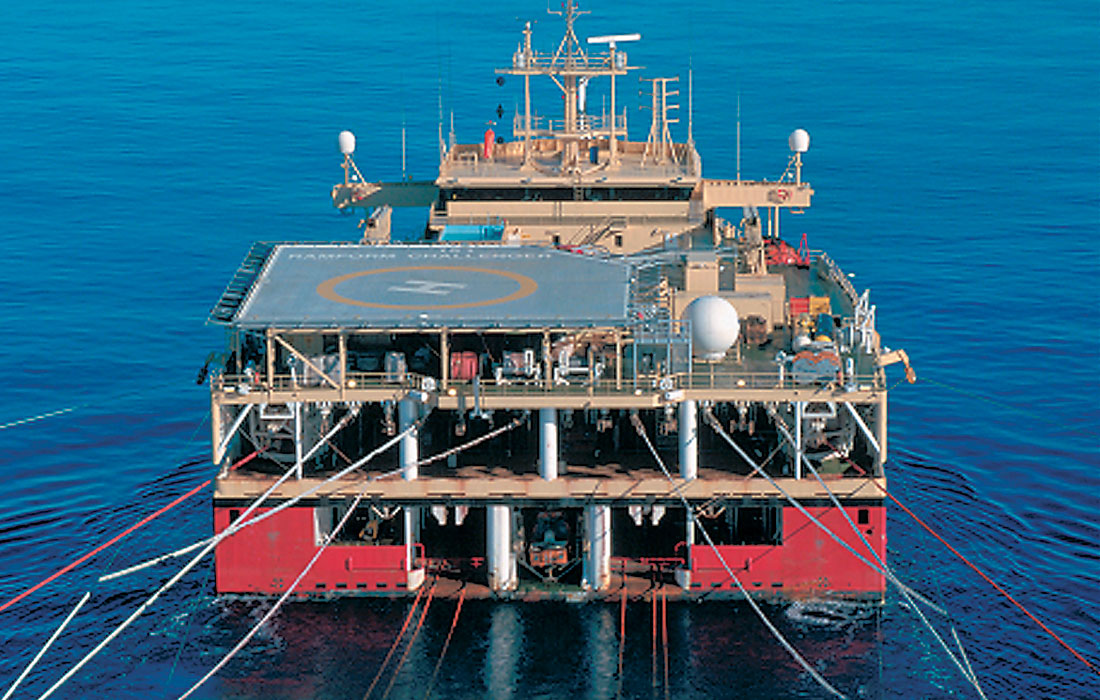
Dual-source shooting has historically dominated streamer acquisition, by comparison to single-source shooting, because the streamer separation with single-source shooting must be halved to preserve cross-line processing fidelity. In the days of limited streamer capacity, small streamer spreads using small streamer separation were prohibitively expensive to deploy. Since 2001, the ability to tow 12 to 16 long streamers at 37.5 to 50.0 m separation, with no loss of efficiency (i.e. no increase in downtime), has reduced costs so that single-source acquisition is cost effective (Hegna et al., 2001; Long et al., 2003). Such surveys have benefits of increased fold, improved 3D spatial resolution, and improved imaging quality. At the same time, certain survey locations are more amenable to dual-source shooting in high-density (close streamer separation) 3D mode, where the gains in cross-line spatial sampling more than offset the compromise in fold.
As the best possible resolution for a given midpoint range can be obtained with a 3D zero-offset gather (Vermeer, 1999) in the case of no noise, full offset stack resolution will be inferior to the resolution of that zero-offset gather. However, in a noise environment, where fold is required, resolution is a function of spatial sampling, frequency bandwidth, aperture size (Chen and Schuster, 1999), and subsurface illumination. Regone (1998) demonstrates that coarse spatial sampling in any direction will compromise migration success far more seriously than limited fold.
At this stage it is worthwhile considering the merits of the inline shot density. In comparison to dual-source shooting, single-source shooting doubles CMP fold (increasing the S/N ratio by 40%) and halves the trace spacing in the common-offset, -receiver, and –midpoint domains, thereby optimizing all multi-channel pre-stack processing operations. However, the associated doubling of the cross-line bin size for a given s t reamer separation will compromise cross-line (and full volume) resolution unless the streamer separation is correspondingly halved. In the case of 37.5 m streamer separation, single-source shooting with 2:1 receiver summation typically yields 18.75 x 12.5 m bins at high fold. Alternatively, for 50 m streamer separation, dual-source shooting with 2:1 receiver summation typically yields 12.5 x 12.5 m bins with halved fold (compared to single-source shooting), and doubled inline shot separation. Overall, dense streamer acquisition provides tight receiver sampling in all directions and tight shot sampling in the shooting (inline) direction.
Many reservoir targets have complex structural settings and are associated with significant diffraction energy emanating from faults and steeply dipping features. To properly image such data, it is critical that diffraction energy is not aliased. In general, on unmigrated seismic data, the energy that is reflected and scattered from near-surface features appears as a series of linear events in the seismic data, being extensive across most arrival times. In 3-dimensional space, these events are actually hyperbolae that have an apex at the point of scattering, i.e. the location of the near-surface discontinuity. The most effective means of removing these events from the target events is through proper 3D migration. The basic problem often encountered in the migration of these scattered events is that, because of their steep apparent dip on the unmigrated seismic data, they are usually severely aliased. When migration of these aliased events is performed with most migration operators, the operator can become aliased (Biondi, 1998), resulting in “noise” being introduced into the data, contaminating the seismic section and reducing the resolution of events. In areas where diffraction noise is prevalent, it is possible that much of the (random) noise inherent throughout the data may be the result of migration operator aliasing. It should be remembered that the purpose of correctly migrating these diffraction events is not to correctly image the near-surface discontinuities that generated them, but to remove the diffractions, and their associated migration noise, from the reflection events near the target depth. Therefore, bin dimensions should be as small as possible prior to migration. Figure 2 (collated from Yilmaz, 1987) demonstrates the effects of migration aliasing.
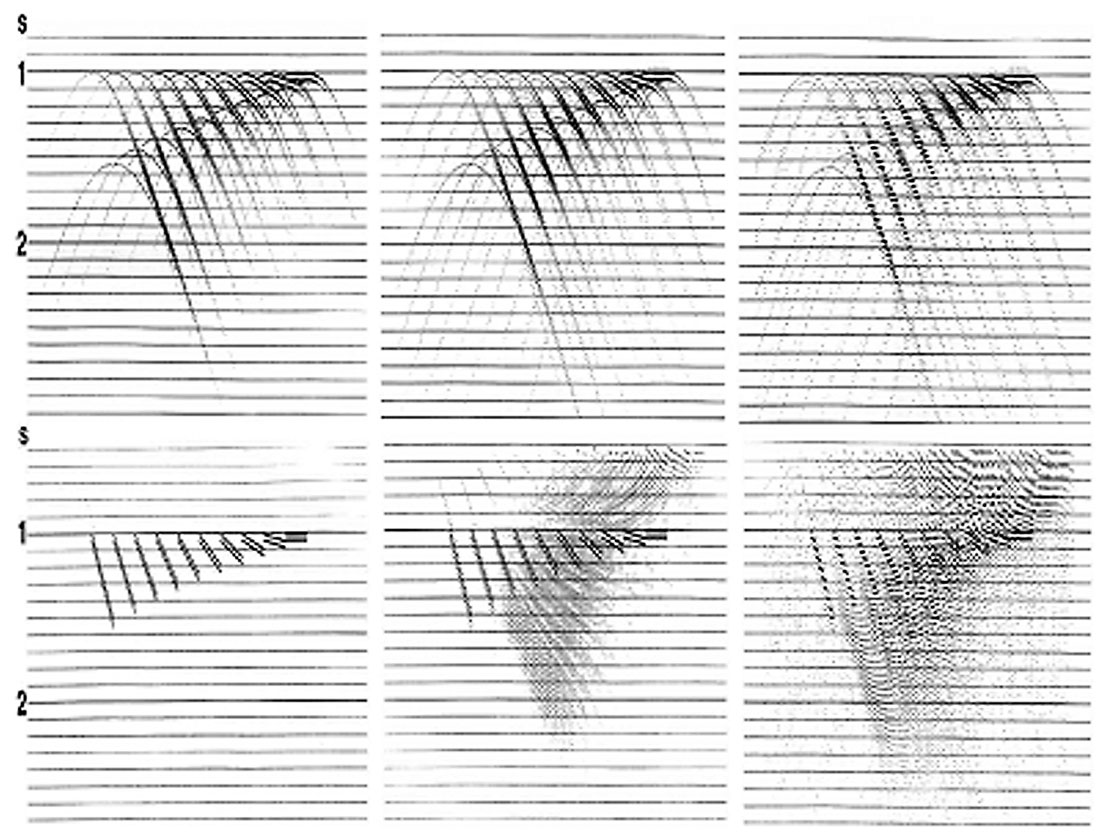
Overall, the high fold and dense spatial sampling offered by HD3D acquisition leads to 3D data with higher resolution and higher S/N ratio. Furthermore, significant improvements are possible in many of the necessary steps in data processing such as velocity determination, multiple removal, noise attenuation and migration imaging solutions.
Offshore Philippines HD3D Case Study
PGS acquired the 1100 km2 Offshore Philippines multi-client HD3D survey during 2002 (Figure 3). A structurally complex carbonate reservoir occurs at about 3.2 s TWT, below a very rugose, deep, water bottom, and undercompacted, stratigraphically complex overburden. Previous 3D data (refer to Figure 5) had failed to yield satisfactory images of the overburden, and many structural ambiguities at the deep reservoir level confronted the drilling and production development of reserves.
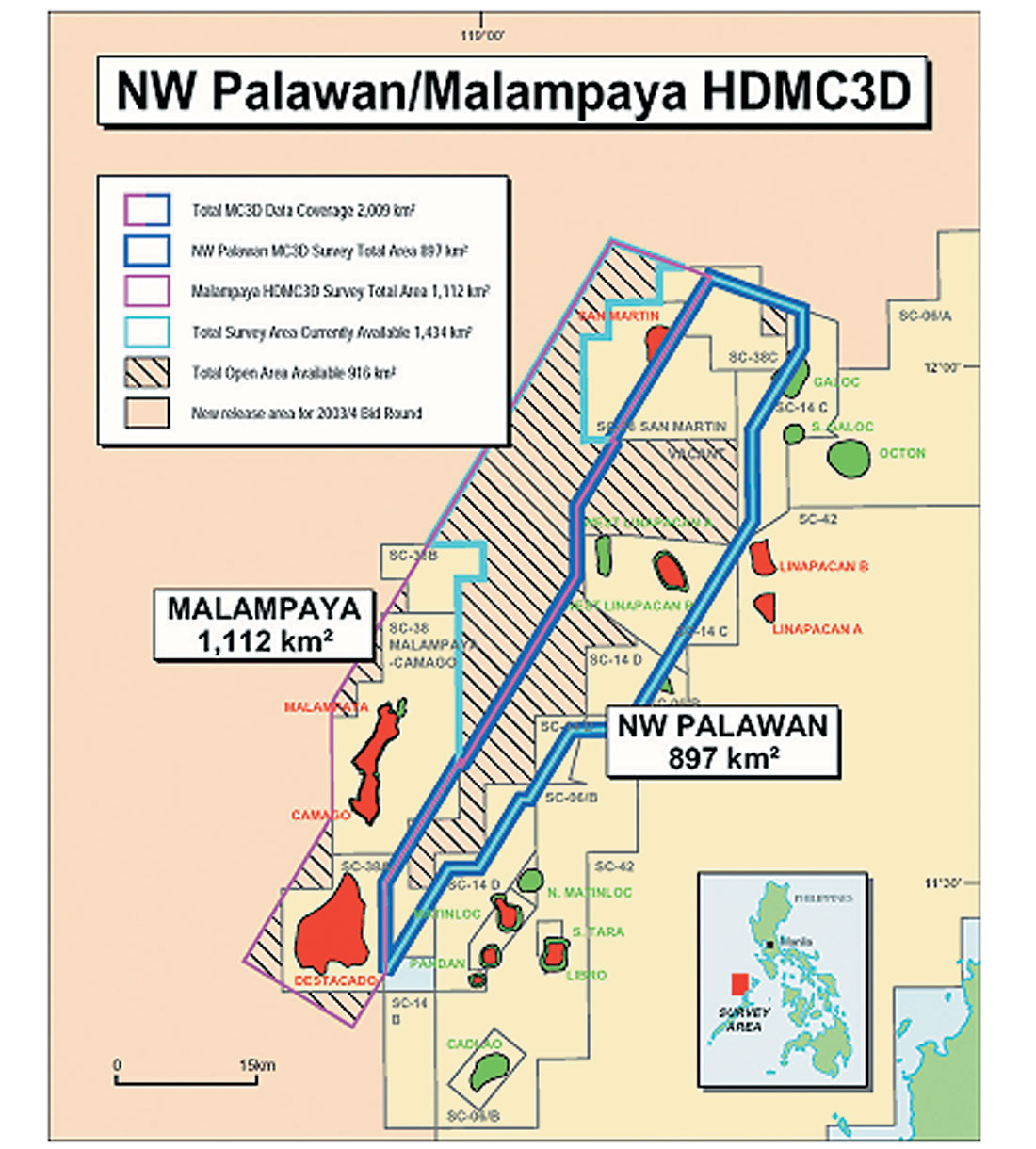
Pre-survey planning by both PGS and the multi-client survey participants concluded that “strike” shooting would be used with anti-parallel sail line shooting to improve the uniformity of subsurface target illumination coverage. Strict vertical resolution requirements sought 70 – 80 Hz frequencies at the 3.0 – 3.2 s TWT target level. Consequently, source/streamer depths of 5.0/6.0 m were used. Modeling of the horizontal resolution requirements indicated that to image the steepest dips, the premigration bin size must be no larger than 12.8 m. In the pursuit of optimal resolution, it was decided that 12.5 m cross-line spatial sampling would be acquired.
The M/V Ramform Challenger was used to tow 12 x 4500 m streamers at 50 m separation, shooting in dual-source mode at 18.75 m shot interval. Thus, the natural CMP bin size (inline x cross-line) was 6.25 x 12.5 m, at 54 fold. In terms of trace densities, the new HD3D survey yielded 691,000 traces/km2, in comparison to 95,000 traces/km2 corresponding to existing 3D data in the area.
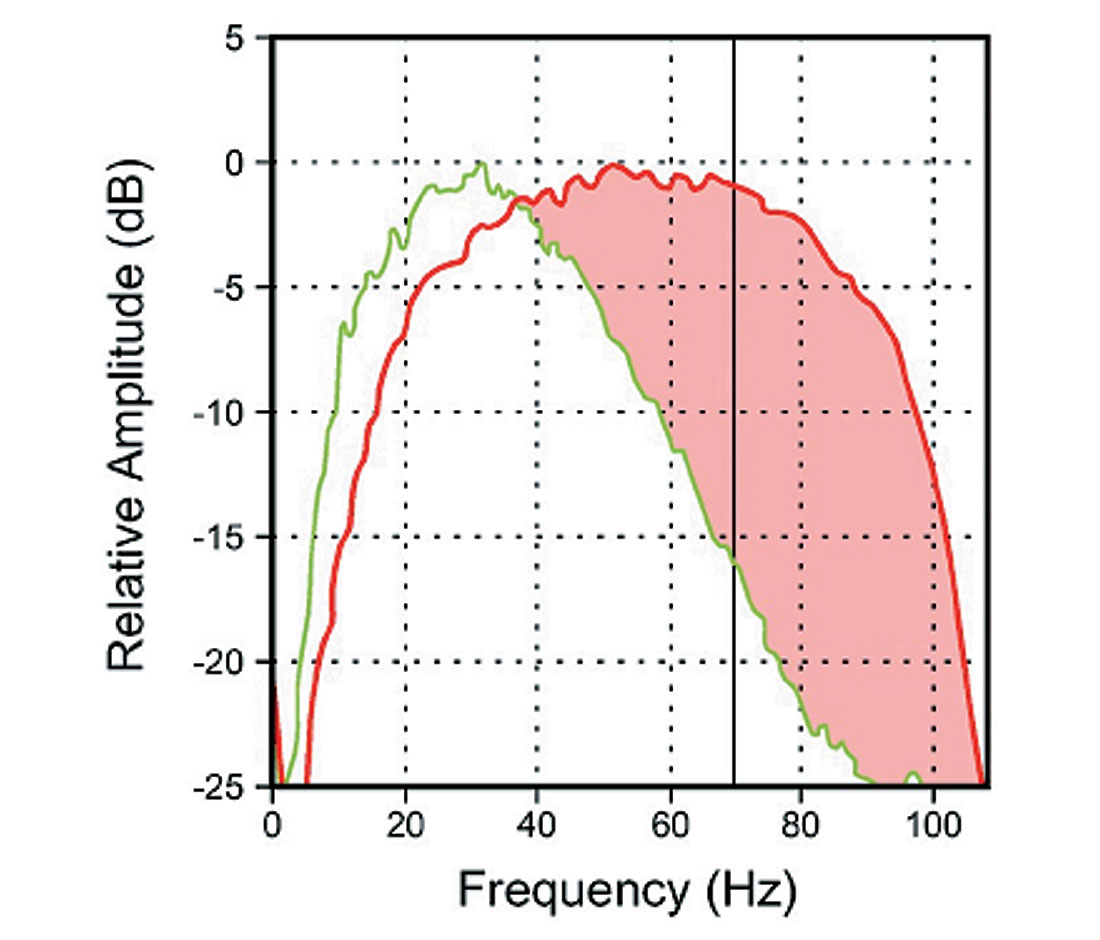
Following the completion of acquisition, full Kirchhoff pre-stack time migration (PSTM) processing was employed. Data comparison to existing 1991 and 1993 3D data indicated significant improvements in the structural imaging and resolution of the target events (Figures 5 and 6). Figure 4 superimposes the comparative amplitude spectra extracted from the data in Figures 5 and 6. At 70 Hz, the new HD3D data above the target is 15 dB stronger than the existing 3D data – without any spectral whitening or Q compensation being applied during processing!
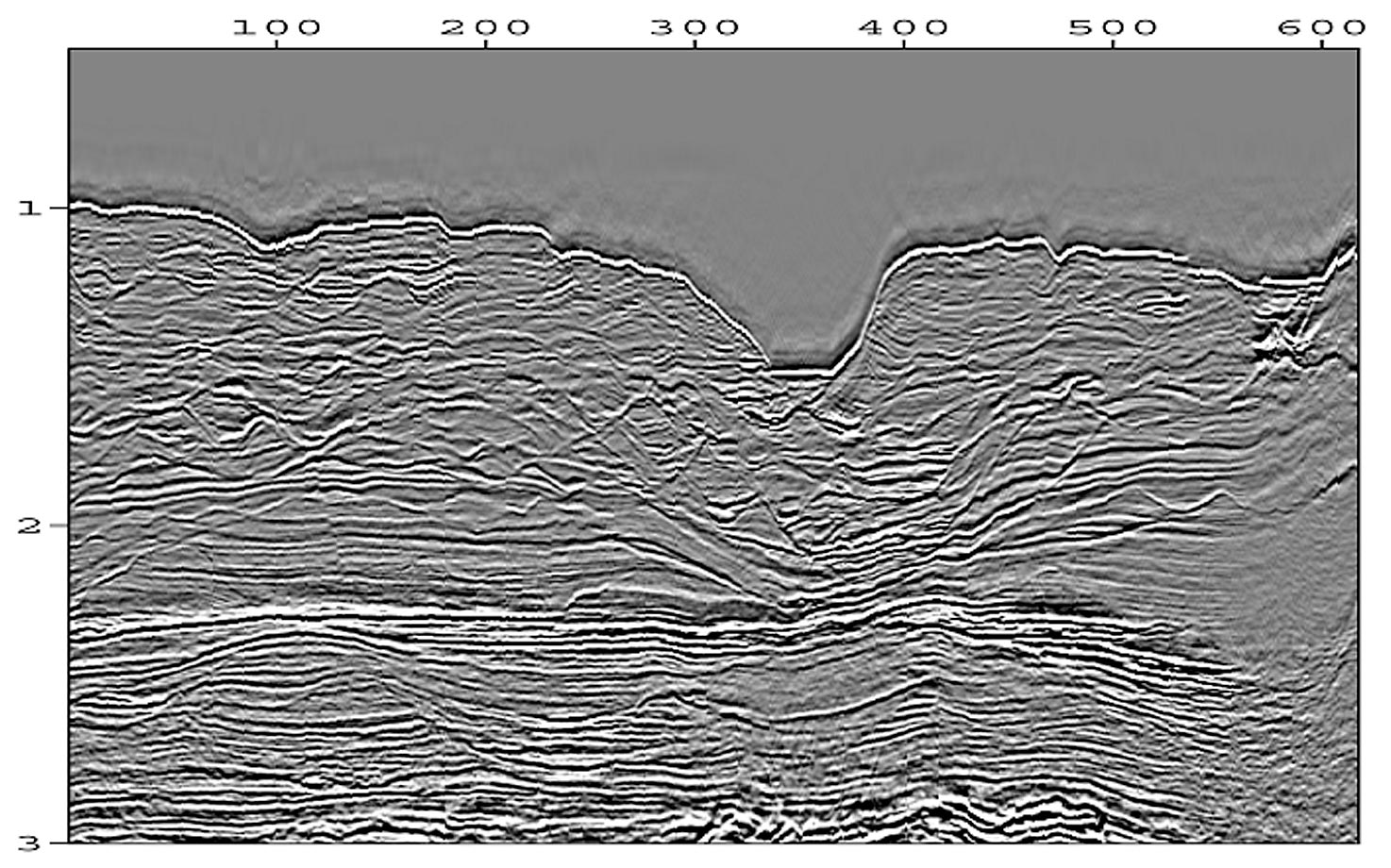
Furthermore, the 2002 PSTM data have better fault resolution than pre-stack depth migration (PSDM) versions of the earlier 3D data sets. Such statistics are testament to the power of high-density 3D acquisition, even in an area containing rugose water bottom and chaotic stratigraphic trends.
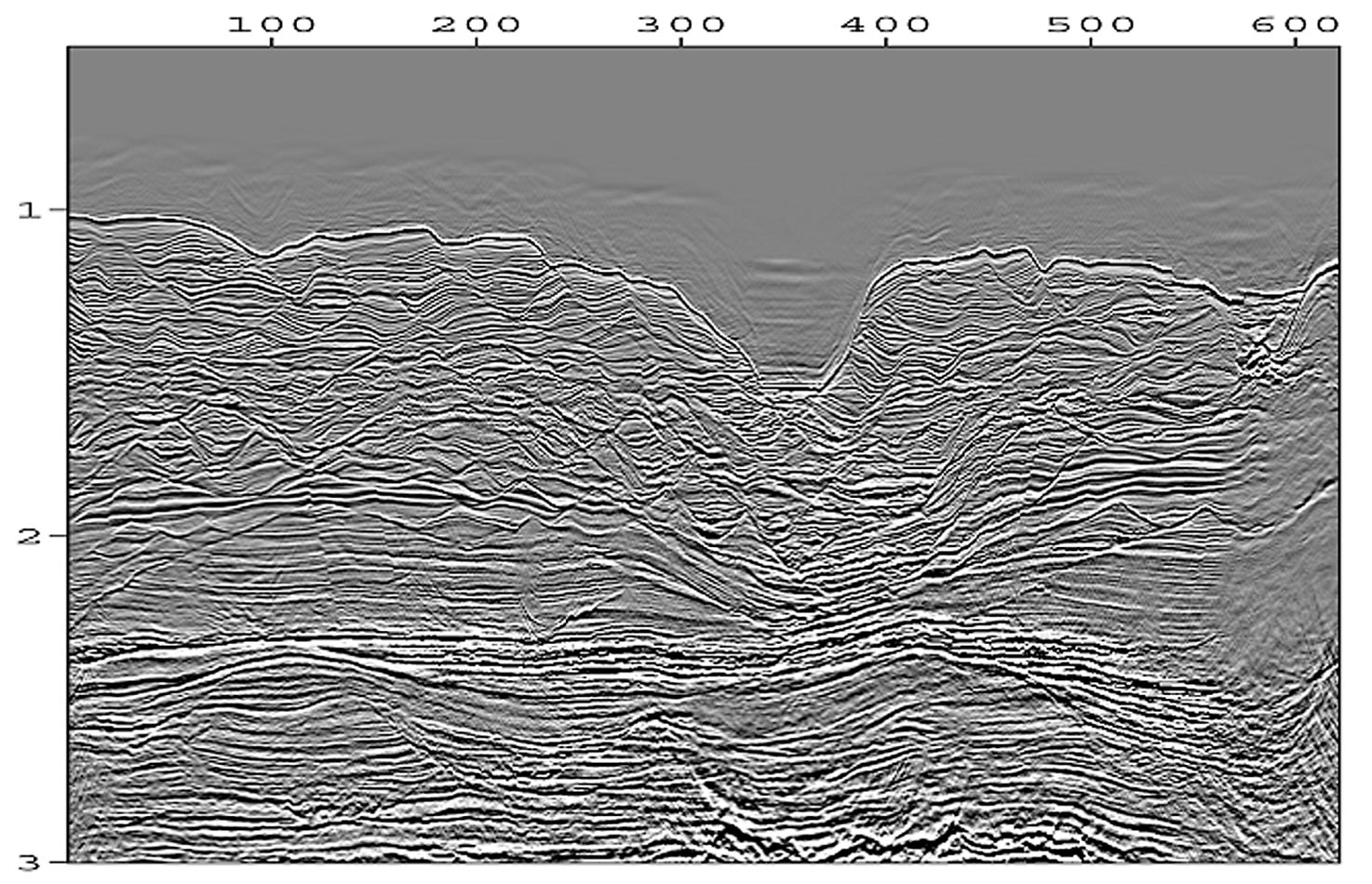
Why is the difference in frequency bandwidth between Figures 5 and 6 so dramatic? Post-survey analysis of all the acquisition parameters contributing to frequency content indicates that the difference in cross-line spatial sampling is by far the most important factor. In the original 3D case, steeply-dipping shallow events and diffraction energy cannot be imaged with frequencies larger than about 60 Hz. In the HD3D case, more than twice as many unaliased frequencies can be acquired and processed. At depth, the effects of anelastic Earth attenuation restrict the highest frequency that can be used in both cases. Furthermore, no data contamination from processing and migration noise has affected the HD3D data. Overall, tight 3D spatial sampling has allowed a much larger frequency bandwidth than normal to be acquired unaliased in the field and preserved throughout all stages of processing – notably all those steps that operate on more than one trace at a time (multichannel processing). The net result is the significant improvement in resolution and interpretability observed in Figure 6.
East Java Sea HD3D Case Study
PGS acquired a 2729 km multi-client 2D survey in 2002 and a 3963 km2 multi-client HD3D survey in 2003, both located in the East Java Sea (Figure 7).
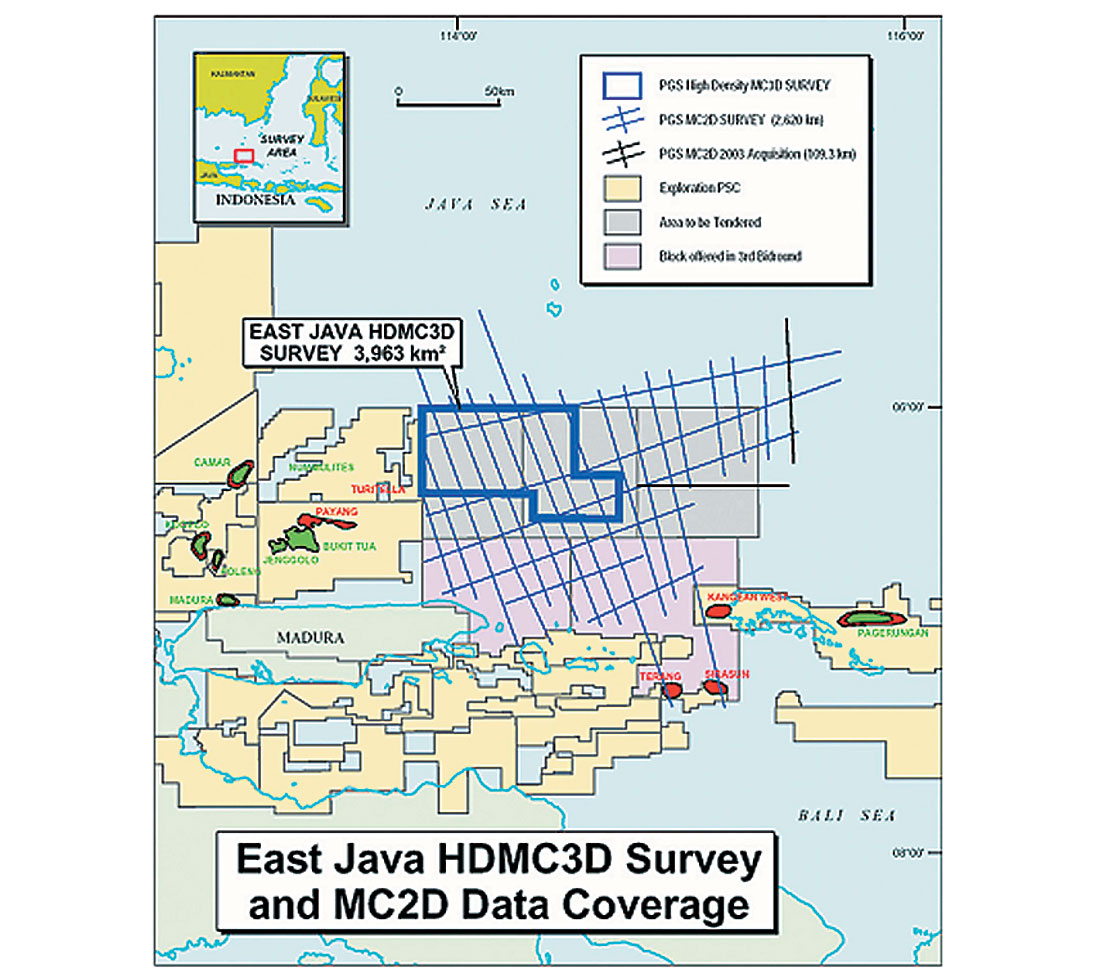
Interpretation of the 2D data allowed for the identification of source rock kitchens, migration fairways, and the main tectonic events in the area. Several prospective features were interpreted and influenced the location of the subsequent 3D survey. A complex series of shallow (Kujung) carbonate build-ups dominate the HD3D area and were given special attention during the pre-survey planning phase of the 3D survey. In particular, the ability to pursue high-resolution interpretation of shallow, steeply-dipping features demanded an emphasis upon very tight 3D spatial sampling.
The M/V Ramform Challenger was used to tow 12 x 3600 m streamers at 62.5 m streamer separation, shooting in dual-source mode at 12.5 m shot interval. Record length was 4.0 seconds and nominal fold was 72. In comparison to standard 3D acquisition, this represented a significantly increased emphasis upon tight spatial sampling in all directions combined with maximum fold. In terms of trace densities, the HD3D survey with its 6.25 x 15.625 m CMPbins yields 3.2 times the trace density per square kilometer (737,280 vs. 230,400). As previously established, high trace densities are an essential prerequisite for successful pre-stack migration – a key processing step in the East Java Sea HD3D survey.
The high signal-to-noise quality and excellent resolution of the East Java Sea HD3D data are testament to the virtues of tight 3D spatial sampling possible with the HD3D acquisition method (Figure 8). The HD3D seismic data have revealed the presence of numerous, often complex prospects on both the upper and lower K/0ujung levels as well as at the deeper basement levels. Some of these structures may have closures of greater than 100 km2 (Johansen, 2003). Migration pathways can thus be interpreted, making detailed prospectivity analysis possible.
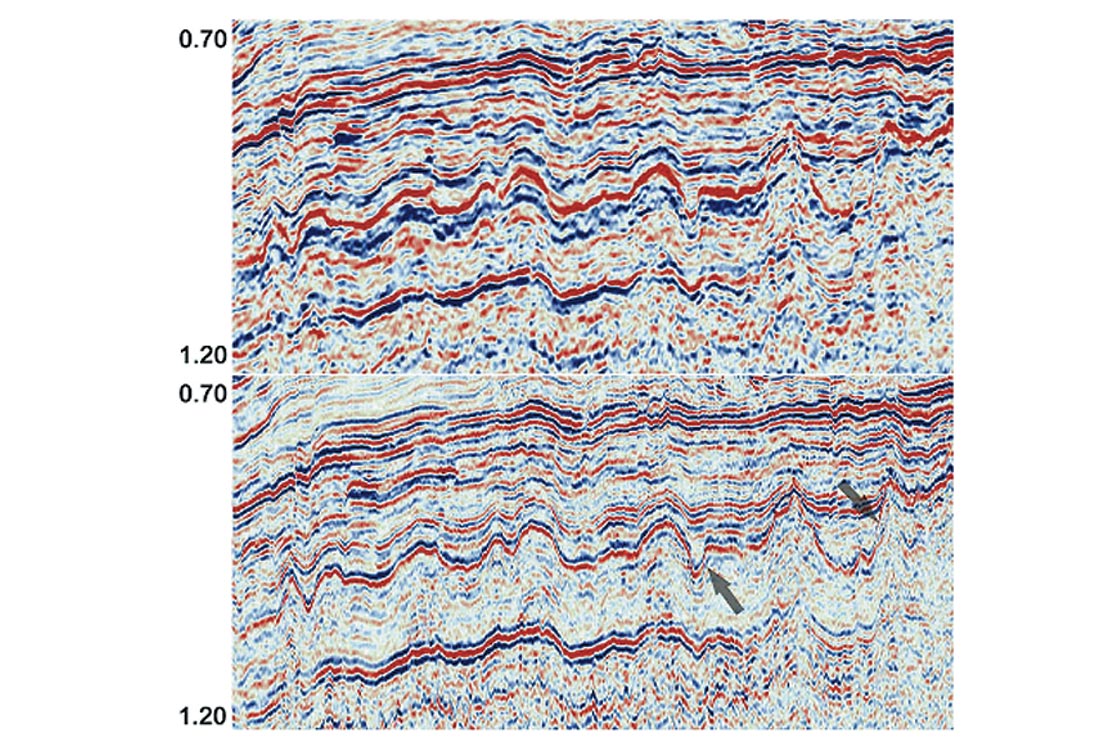
A common frustration with 3D acquisition is that high-quality resolution observed on existing 2D data is not observed at a comparable standard on new 3D data. Ideally, the structural imaging power of the 3D method combined with high resolution in all directions is desired. As standard 3D acquisition typically samples 4 to 6 times more finely in the shooting (inline) direction, resolution is compromised in the cross-line direction. Figure 8 shows that the resolution of the HD3D data is significantly improved compared to the 2D data, demonstrating how 3D pre-stack migration benefits from tightly sampled, unaliased 3D data.
Figure 9 presents a migrated time slice at 0.15 s TWT. Note the negligible acquisition footprint, even at such a shallow depth. The resolution and definition of complex meandering channels systems is quite astounding, testament to the resolution power of the HD3D acquisition method in dual-source shooting mode.
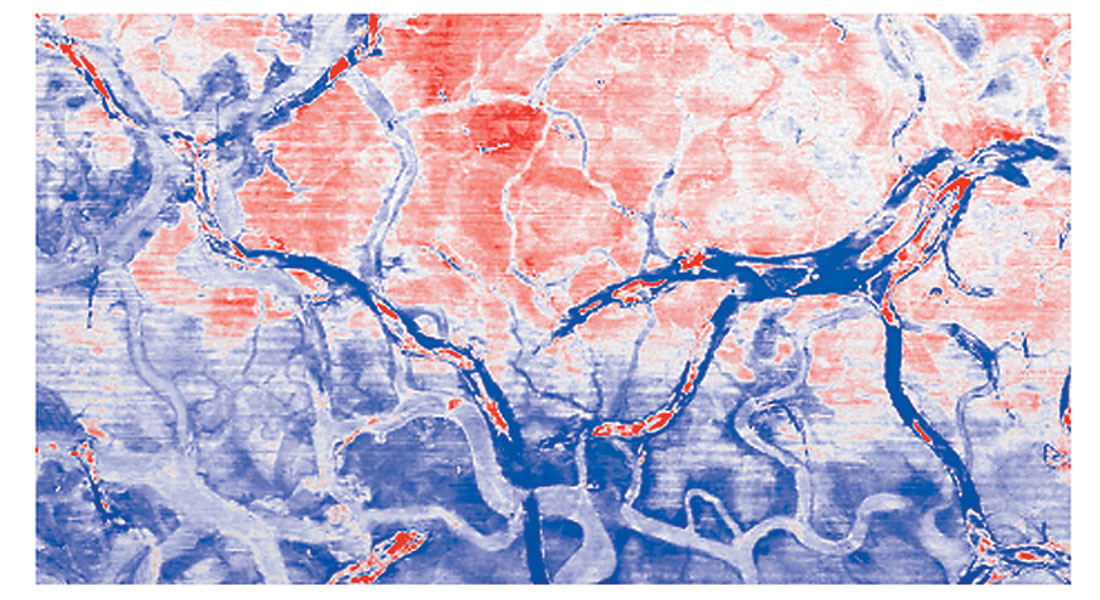
Figure 10 presents a deeper time slice (1.0 s TWT), intersecting the Kujung carbonate features. Again, resolution is excellent, evidenced also in the interpreted 3D surface plotted in Figure 11. Frequency analysis indicates primary event frequencies in excess of 80 Hz throughout the target region before any Q compensation or spectral whitening. Such processes will obviously improve the frequency bandwidth even further.
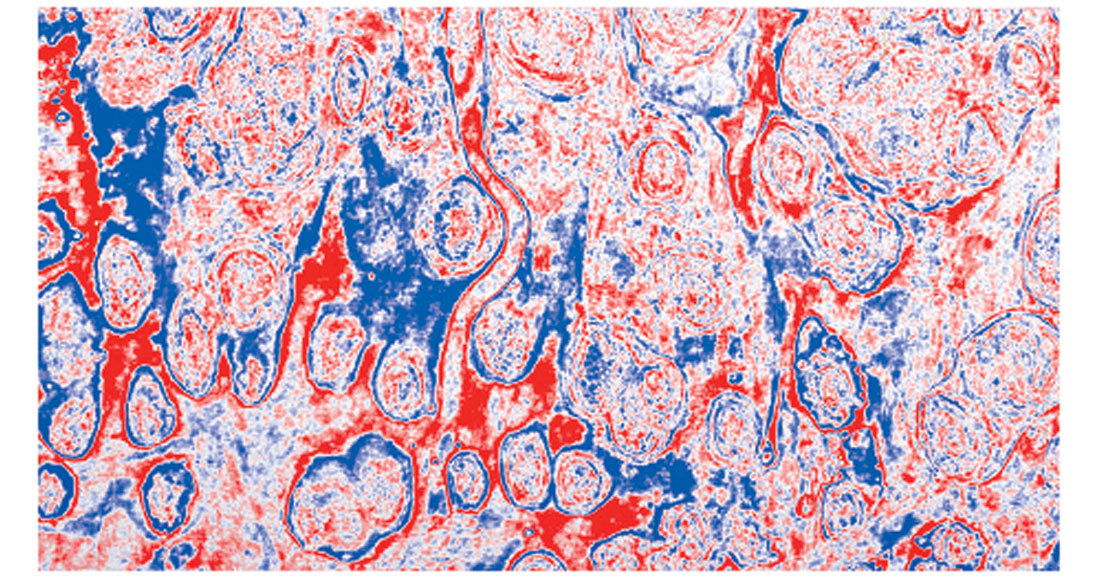
Looking back at Figure 8 serves as a reminder that seismic data will always be heavily contaminated with complex 3D scattering and diffraction energy. It is no surprise that out-of-the-plane events have rendered the 2D data comparatively low in resolution. However, if tight 3D spatial sampling (close streamer separation, and dual-source shooting) had not been used in this area, the final 3D images would have been similarly degraded in resolution.
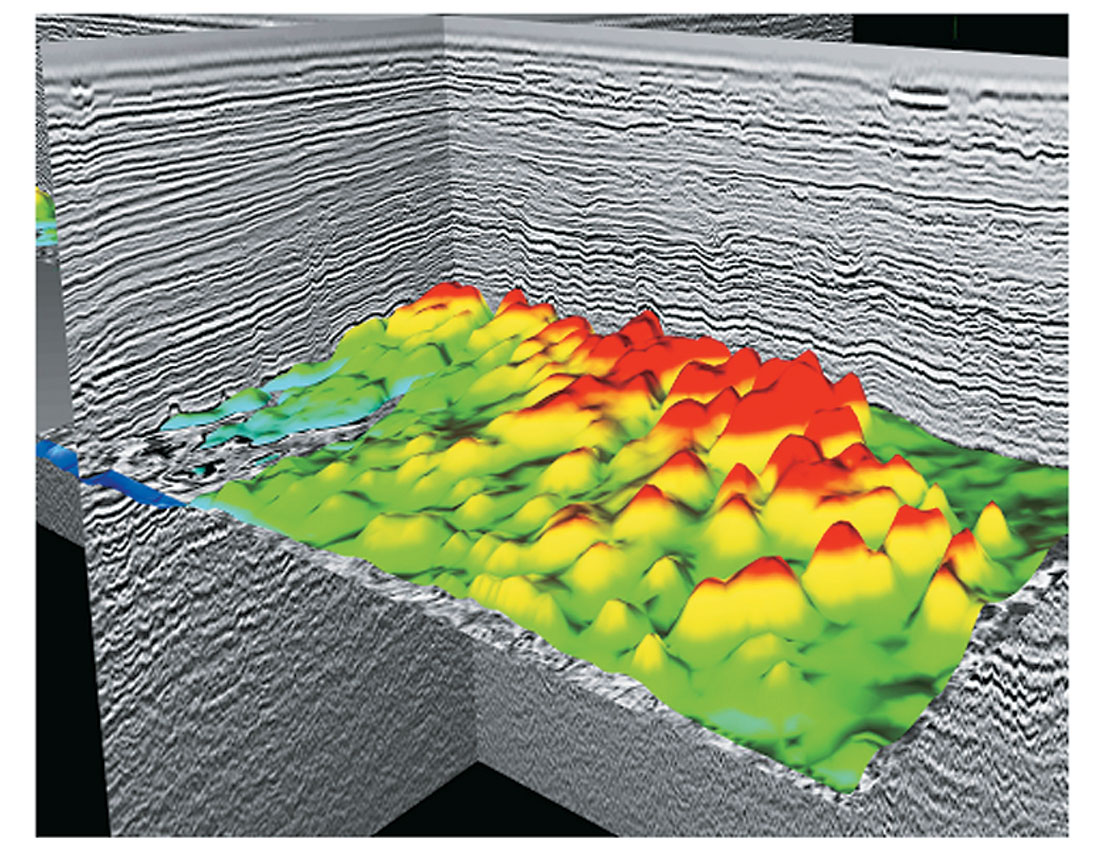
Conclusions
We have seen that high-density 3D acquisition in dual-source shooting mode yields very tight 3D spatial sampling. The benefits for significantly increased data resolution (both temporal and spatial) are evident at all data depths and locations. Small-scale faults and stratigraphic trends become quite dramatically evident, even at very shallow depths where efficient scale multi-streamer spreads might have historically been regarded with caution. It is demonstrated that the ability to acquire and use a large frequency bandwidth from shallower events, free of aliasing, prevents processing noise fro m degrading deeper data quality and resolution. At depth, the only limiting factor upon frequency bandwidth is then natural anelastic attenuation.
In the Offshore Philippines HD3D survey, the deep, structurally complex carbonate reservoir was imaged with great clarity and resolution despite the severely rugose water bottom and stratigraphically complex overburden. In the East Java Sea HD3D survey, very steeply-dipping, shallow carbonate flanks were also imaged with great clarity and resolution, enabling the precise mapping of the many small and large prospective structures that are pervasive throughout the area. In addition, deeper migration pathways and source kitchens below the complex Kujung I, II, and III carbonate layers are imaged with high signal-to-noise quality, thanks to the imaging power possible with densely acquired data.
The significant increase in frequency bandwidth observed at all arrival times and the associated improvements in data resolution and signal-to-noise quality are a direct consequence of the tight 3D spatial sampling inherent in the HD3D acquisition method. In both the cases discussed here, a powerful demonstration is made that very high resolution is only achievable when tight 3D spatial sampling is the centerpiece of acquisition.
Acknowledgements
Thanks to PGS Marine Geophysical for permission to publish these data, and to the members of the East Java Sea HD3D and Offshore Philippines HD3D QC committees for their project management.
HD3D and holoSeis are registered marks of Petroleum Geo-Services.
Join the Conversation
Interested in starting, or contributing to a conversation about an article or issue of the RECORDER? Join our CSEG LinkedIn Group.
Share This Article