Since the acquisition of seismic data in the 1960’s, impact structures have been seen in seismic data. These are structures that were created earlier in the Earth’s history from a meteorite impact and have since been buried underground. While many of these unique structures are known to be located in the Western Canadian Sedimentary Basin, we also find these buried impact craters around the world with many well-known astroblemes located in the US. Some of these structures occur in areas with oil and/or gas concentrations and are of interest to the industry for economic reasons, while others exist in zones without any hydrocarbons and are of scientific interest in gaining a better understanding of these structures and events. As an astrobleme hunter and seismic processor the goal of this project is to seek out these buried impact structures and study them using the seismic data processing methods conventional to the Canadian oil and gas industry.
Theory
Before we can begin to analyse these complex impact craters, first we need to know how they are created. We will take a step into the realm of astronomy to understand why we see these unique structures on the Earth.
Our solar system is full of leftover debris from its formation. When one of these smaller meteoroids passes near the Earth, the gravity of our planet pulls it towards the surface. If the meteor doesn’t burn up in our atmosphere, the resulting meteorite impacts the surface of the Earth and creates a circular imprint structure that we then refer to as an impact crater or an astrobleme. “Impact Crater” is the astronomical term and refers to a depression that results from the impact of a natural object from interplanetary space with Earth or with other comparatively large solid bodies such as the Moon, other planets and their satellites, or larger asteroids and comets. “Astrobleme” is the geoscience term that comes from ancient Greek “astron” and “blema” (which translates to “star wound”), and describes the remains of an ancient meteoriteimpact structure on the Earth’s surface, generally in the form of a circular scar of crushed and deformed bedrock. For the sake of this project, both “impact crater” and “astrobleme” can be used interchangeably.
Remarkably, the general structure of these impact events does not depend on the composition of the meteorite nor the pre-existing rock on the surface; rather it depends on the size of the meteorite which is proportional to the amount of energy released in the impact (Melosh, H.J., 1989). Simply put, the larger the meteorite, the more energy is involved in the impact and so the larger and more complex the structure. Impact craters are categorized into two main groups depending on their size and complexity of the overall structure and are called simple craters or complex craters (Melosh, H.J., 1989). Figures 1 and 2 show examples of the structure and features seen in both types of craters.
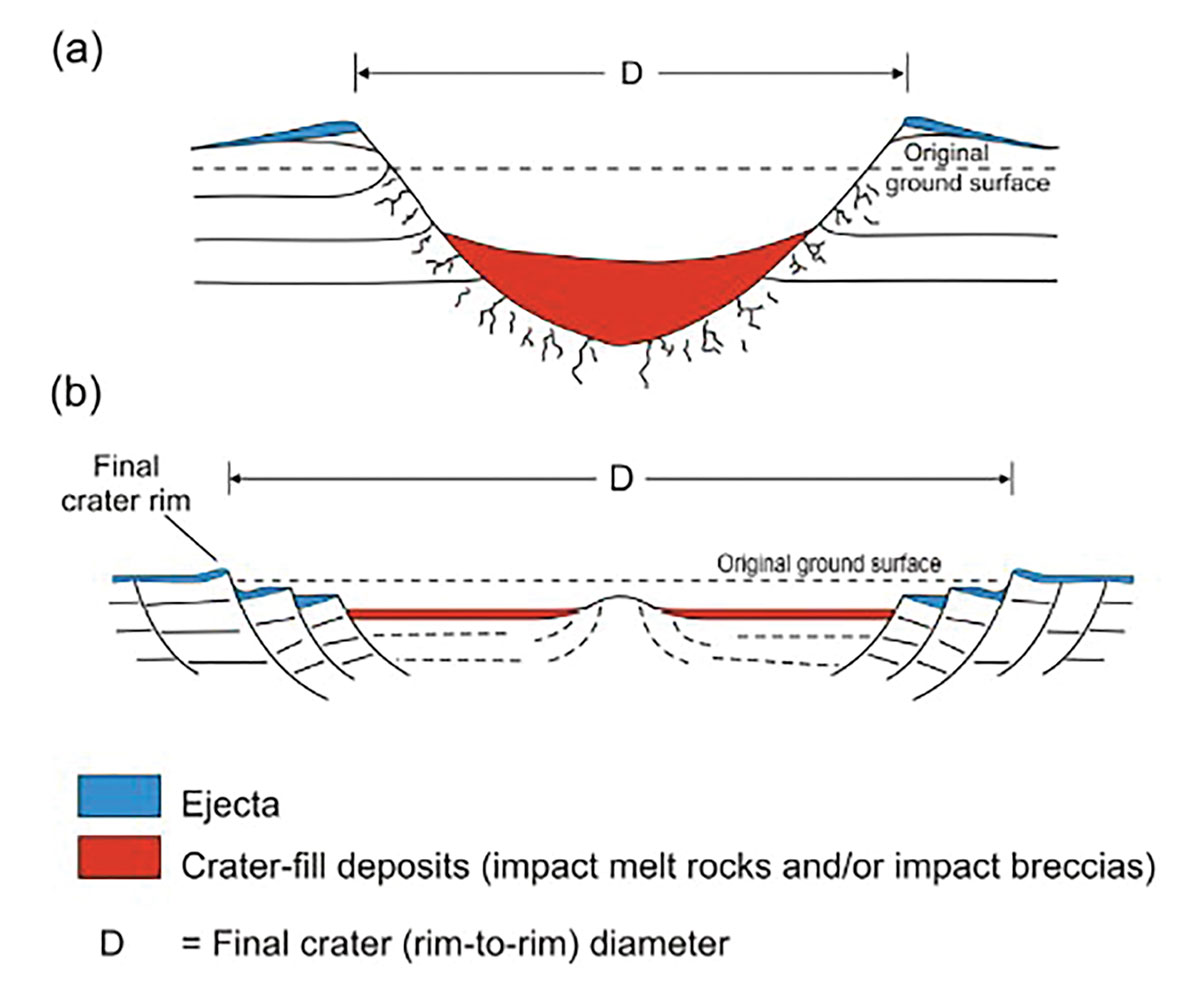
While both have a circular bowl-like shape to them, in the case of the complex crater, the bedrock material in the center of the impact side has rebounded creating what is called a central peak structure. The presence or absence of this central peak is the main defining feature between a simple crater and complex crater. In both types of craters we often see the structure filled in with breccia impact melt which is a combination of the melted meteorite along with any surface material melted during the impact, along with any material that was ejected during the impact that had fallen back into the new depression after the event occurs (Melosh, H.J., 1989). Circular rings of faulting that parallel the rim of the structure are also common in larger impact events and this is especially important for the hydrocarbon industry as this is often what creates the traps for the oil and gas associated with these events.
Now that we have a basic understanding of these impact structures and what causes them, let’s take a look at some well-known astrobleme discoveries.
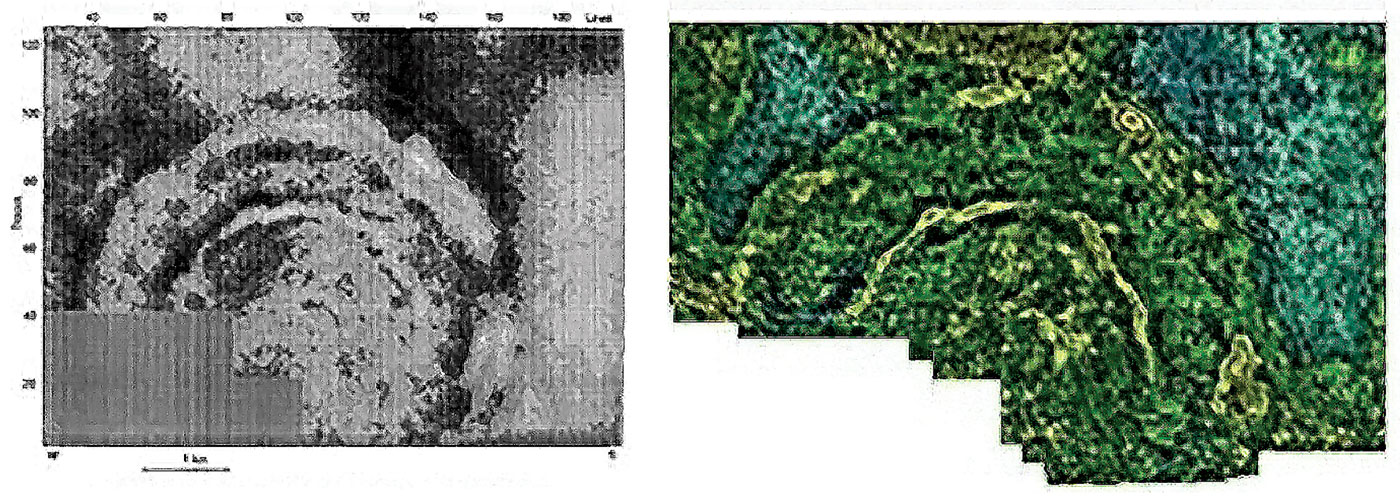
Past Discoveries
Over the years many sub-surface impact craters have been discovered through acquiring and interpreting seismic data. While they have been found all over the Earth here are two examples of astroblemes that are well known to the Canadian oil and gas industry.
VIEWFIELD: Discovered in 1969 and located in southeastern Saskatchewan, the Viewfield structure was among the earliest impact structures discovered in seismic data. While being an interesting find, this crater gained notice because of the large hydrocarbon accumulation associated with it, predominantly along the rim on the structure. Over the years there have been over 50 active wells in the rim structure of this astrobleme with a reserve of 27 million barrels (Sawatzky, H. B., 1972).
STEEN RIVER: Located in northern Alberta, the 25 km diameter Steen River structure is the largest known impact crater in the Western Canadian Sedimentary Basin. The oil, and subsequently the impact structure, were discovered in 1963, but the area remained dormant for many years due to the remote location and lack of pipeline infrastructure. When the assets were bought in the mid 1990’s exploration and drilling in this region resumed (Robertson, G. A., 1997). Since then, more than 130 seismic lines have been obtained across this feature, including several 3D seismic data projects, and over 40 wells have been drilled around the rim (Mazur, M.J., et. al, 2000). This impact crater also has striking magnetic field anomalies around the central peak, and concentric gravity anomalies that correlate to both the magnetic anomalies and the crater structure itself (Hildebrand, A. R., et. al, 1997).
New Techniques
As new and improved seismic data processing techniques are developed, so we improve our ability to analyse and understand these buried astrobleme events. Using new processing techniques on old or well-known impact event is a great way to increase our understanding of these structures, but also test these new processing techniques to confirm that they do indeed work in complex structural environments. In particular two new processing techniques have proved valuable in this regard and they are Divestco’s diffraction imaging and SPRINT 6D data interpolation.
It is well known that interpolation on seismic data is valuable. Using a 5-D minimum weighted norm interpolation (MWNI) has become standard to interpolate random or regularly missing traces from a seismic dataset. Divestco has improved upon this method with SPRINT 6D interpolation that uses an angular weighted apriori model in the frequency-wavenumber domain that has a dealiasing capability, especially for datasets that have regularly missing traces or in complex structural areas. This is especially important for old seismic datasets that contain astroblemes. Since they are very unique structural events we need interpolation software that will maintain the correct structure. Also, if we are going to get more detail out of sparse old datasets we need to upsample the data which creates regularly missing traces that need to be accurately interpolated. Using SPRINT 6D we are able to upsample and improve the image of astroblemes in seismic data. For more information about how SPRINT 6D works, read the paper by Mark Ng, Structure-Preserving 6-D Interpolation.
Diffraction imaging is a new processing technique that focuses on discontinuities in seismic data. Usually these diffractions are difficult to see because they are masked by the prominent reflection events, otherwise referred to as specular energy. While this main reflection energy is the information we typically want to interpret, there is value in looking at just the diffractions hidden in the seismic section. By removing the specular energy and just leaving the diffraction behind we are able to see smaller details and discontinuities like faults and fractures stand out. This is very valuable for impact structures because it allows us to really see the fractured structure created during the impact and can also clearly highlight the rim and central peak structures. If there are rings of faults along the rim we can also identify them easily in a diffraction imaging volume of the data. Overall diffraction imaging allows us to see more details of the overall astrobleme structure that standard seismic data would not allow us to see. If you would like to know more about diffraction imaging, read the 2015 abstract by Carter Edie titled Hiding in Plain Sight: Improving Seismic Resolution Through Diffraction Imaging Technique.
Central Alberta Foothills Cryptoexplosion Event
Located in the foothills of Alberta is an impact crater that is 2.4 km in diameter and buried at a depth of 4500 m in Cambrian sediments. The seismic data for this structure were acquired in 1986 and a paper detailing this astrobleme and the initial processing of these data was published in 1993, titled 3-D Seismic Characterization of a Cryptoexplosion Structure, by J. Helen Isaac and Robert R. Stewart. In 2015 reprocessing of these data began with the focus of improving the image of the impact structure. Using Divestco’s SPRINT 6D interpolation to improve this sparse dataset, and diffraction imaging to better resolve the fractured and faulted nature of the structure, a more detailed image was obtained. By pushing the limits of the data using new processing techniques we improved upon our knowledge of this impact crater.
Hotchkiss Impact Structure: 2D Data example
A well-known astrobleme in the Western Canadian Sedimentary Basin, the Hotchkiss impact structure is located in NW Alberta and is a complex crater approximately 3.5 km in diameter. Shortly after this impact event occurred, this region was subject to erosion that removed a significant portion of the astrobleme structure before being buried below layers of sediment. It is thought that the original impact was 4.5 km across. We are able to deduce this due to the Gething-Debolt unconformity see in the area, and also from looking at a cross section of the astrobleme in seismic data. We see a distinct central uplift, but crater rim cannot be seen and instead the bowl shape of the impact structure only extends to the approximately the same height as the central uplift. Using Divestco’s SPRINT interpolation software, a more detailed cross section of this fascinating impact structure can be seen. In a 1999 CREWES research report it was stated that this astrobleme has gas production from draped sediments overlying the central uplift.
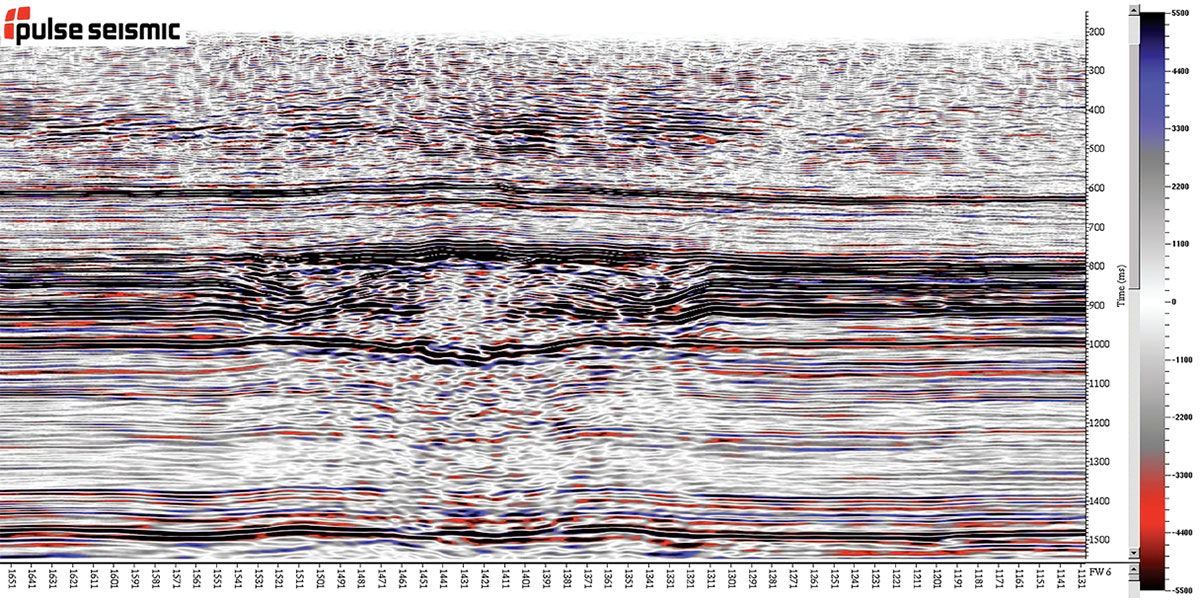
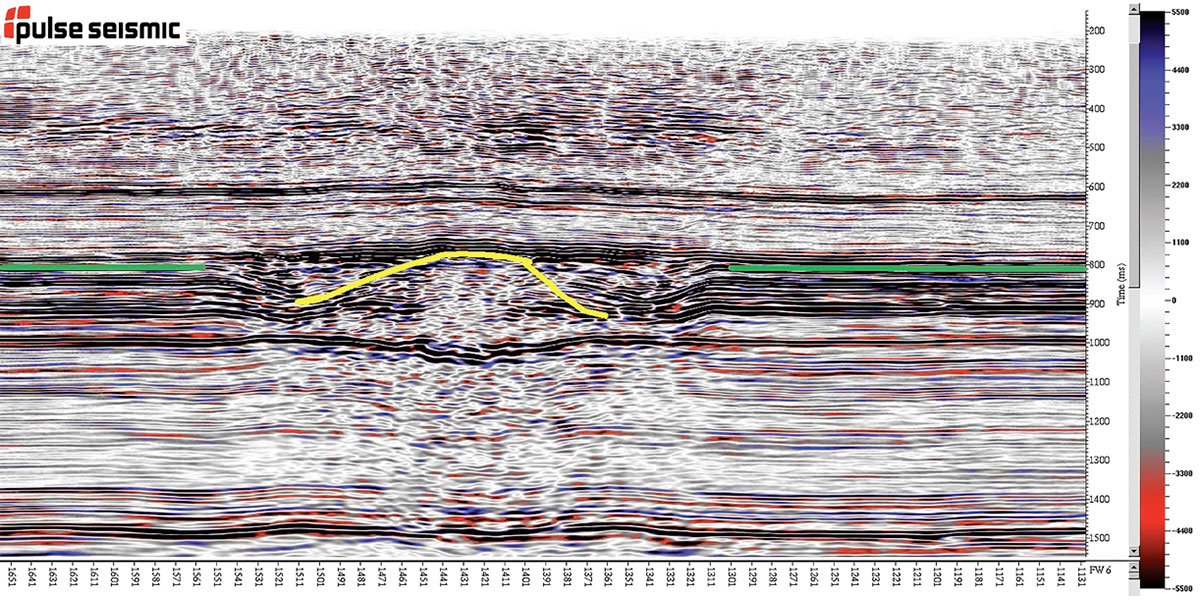
New Discoveries
Hidden away in existing seismic data are many astroblemes that remain undocumented. Those who have worked with the data are aware of their existence, but due to various reasons, there are no publications detailing these structures. The final part of this project seeks to process and analyse astroblemes like these so that a size and age for these impacts can be established and added to databases of known impact structures.
Manyberries Astrobleme: South Eastern Alberta
Near the town of Manyberries in south eastern Alberta is an astrobleme that was undiscovered until seismic data was acquired in the region. No evidence existed on the surface of what lay buried below. Figure 5 shows the location of the astrobleme. Though this impact is a simple crater with a diameter of about 1.5-2 km, it adds to the long list of known impact craters that have been discovered in the Western Canadian Sedimentary Basin.
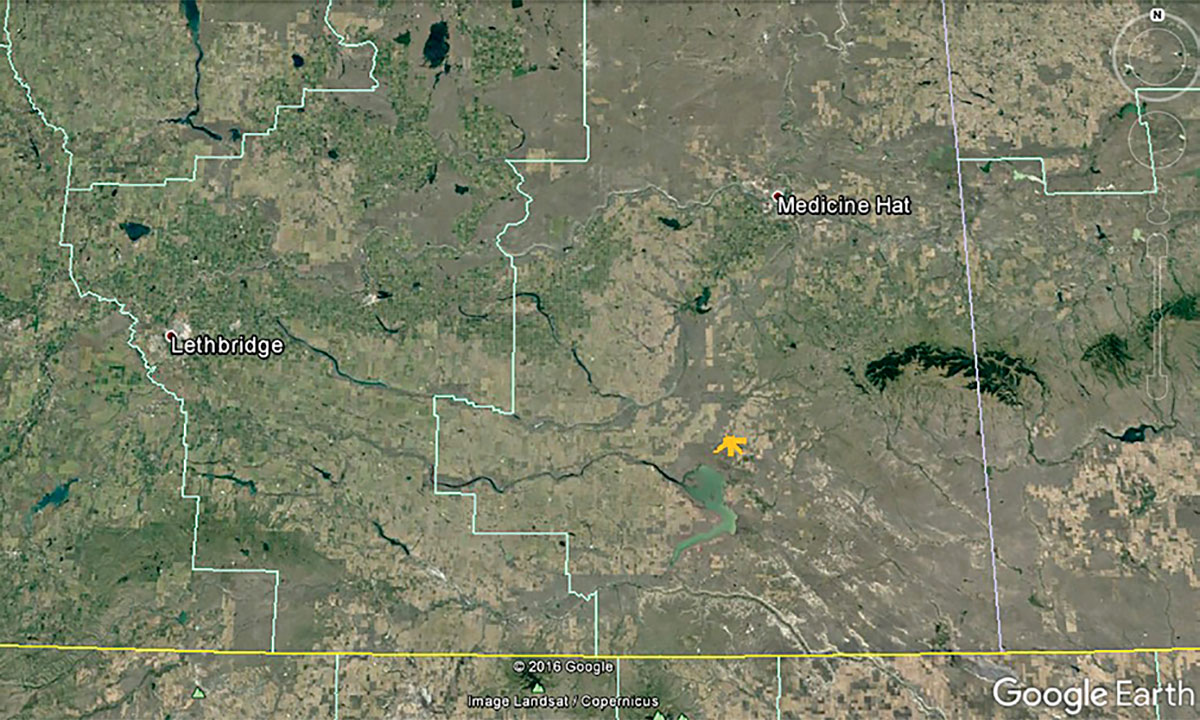
The seismic data that are used for this analysis of the Manyberries astrobleme include four 2D lines that were originally acquired in 1978/79 and are being used with the permission of Pulse Seismic. These lines intersect in a star shape (shown in Figure 6) allowing for a full 360 degrees of azimuth of the impact crater to be analyzed when the data are interpolated between the available lines.
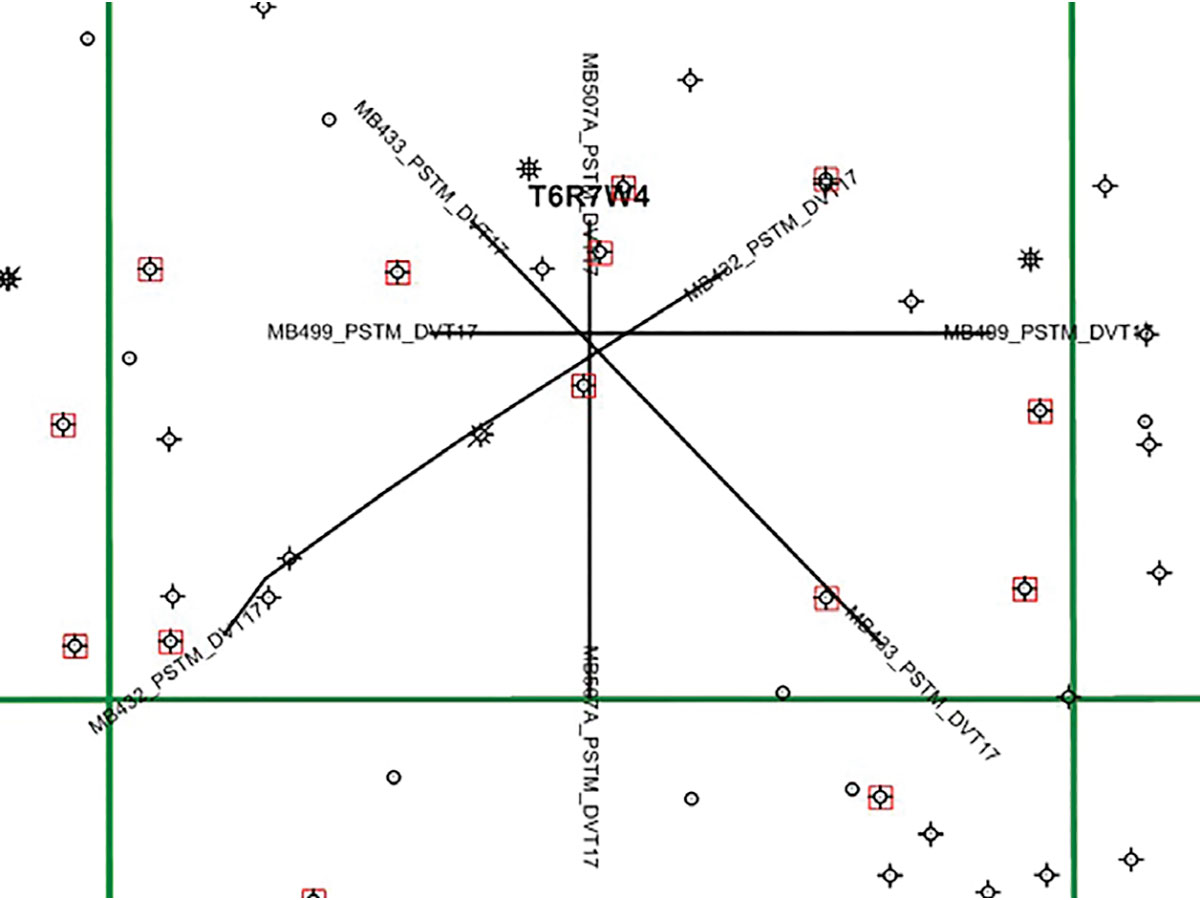
The processing of the seismic data for these four lines followed a conventional processing flow and included the latest 5D interpolation algorithms available to enhance the quality of the data. When the data is stacked, the profile of the impact structure can be clearly seen, shown in Figure 7.
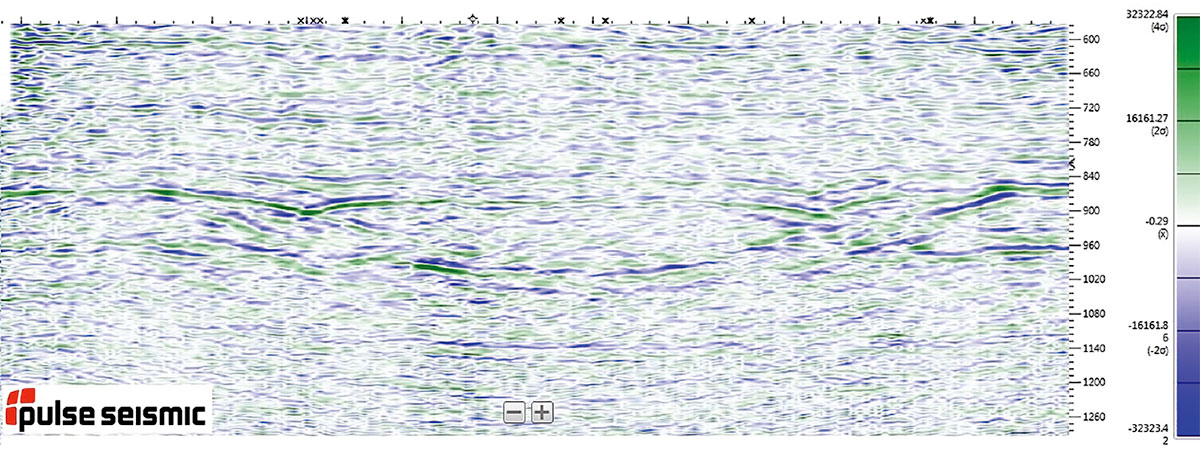
Bringing the lines together, two wells were used to QC the data and determine what layers in which the impact occurred. Wells 16-9 and 13-15 of T6R7W4 were used and tied very well with the seismic data. It also showed that the impact occurred later in the Mississippian or late Jurassic; unfortunately this time frame cannot be narrowed down any further as an erosional event removed the layers around this time period. Using Divestco’s Glass interpretation software, the data from the 2D lines was then extrapolated to fill in a grid surrounding the lines to create a 3D image of the impact structure. Using the Mississippian layer as a guide, we can extrapolate what the astrobleme would have looked like when it was on the surface after the initial impact. Figure 8 shows the astrobleme at this Mississippian layer. From this interpolated image the size of the crater can be calculated to be approx. 1.5-2 km in diameter. Due to the eroisional event mentioned previously, it is difficult to determine precisely where the original crater rim peak occurred to accurately measure its size.
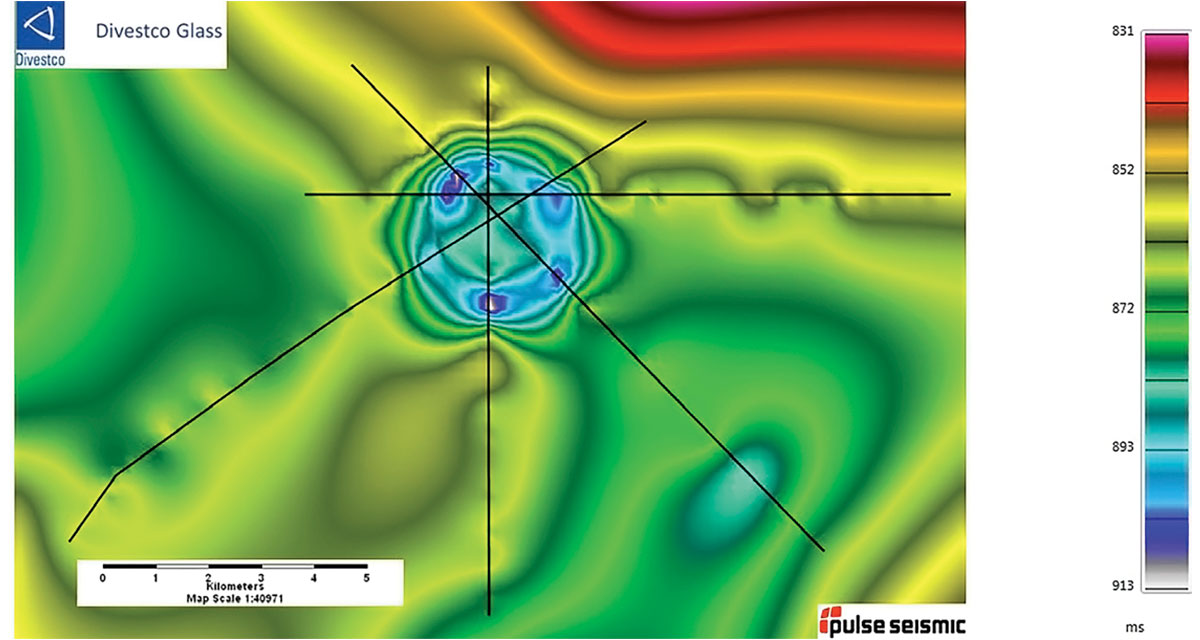
Though this simple crater does not have any hydrocarbons associated with the structure, the exercise of completing the geophysical and geological analysis to determine that this crater is 1.5-2 km in diameter and occurring during the Mississippian to late Jurassic is valuable. This astrobleme can now be added to databases of known impact craters on the surface of the Earth and could be a location of further scientific study of these structures.
Future Work
The main directions for future work for this project involve obtaining new datasets containing astroblemes, and looking into the geology and rock physics properties in the existing impact crater datasets that I have for this project.
For new astrobleme datasets, currently the data for the Australian impact structure Tookoonooka are available to me, and I hope to be able to work on this dataset in the near future. The search for new datasets containing astroblemes is ongoing.
As for the geology and rock properties, it is my hope that the geological features that are known to be associated with surface impact craters can also be detected in these buried impact craters, thus confirming that these structures are indeed of extraterrestrial origin. I also want to see if there is a correlation between the rock properties detected and the presence or absence of hydrocarbons with these astroblemes. If there is a connection between these factors, then this will improve our search and analysis of impact craters for the oil and gas industry.
Conclusion
Impact craters are an important part of the Earth’s history and by uniting astrophysical knowledge with geophysical processing and interpretations we can gain a better understanding of these structures. This will add value for both the oil and gas industry that seeks to extract hydrocarbons from these structures and the scientific community that seeks to better understand and catalogue these impact events.
Acknowledgements
I’d like to thank Pulse Seismic for allowing me to use some of their data that contains astroblemes and to Divestco for allowing me to present this work. In particular I want to thank Todd Keedwell for his help with the interpretation of the seismic data, Neil Watson for his help with the geology interpretation of the Manyberries astrobleme, and George Fairs for his assistance in obtaining the data used for this project. Finally I want to give an extra thanks to Carter Edie, whose encouragement got me started on this project that has become my passion.
Join the Conversation
Interested in starting, or contributing to a conversation about an article or issue of the RECORDER? Join our CSEG LinkedIn Group.
Share This Article